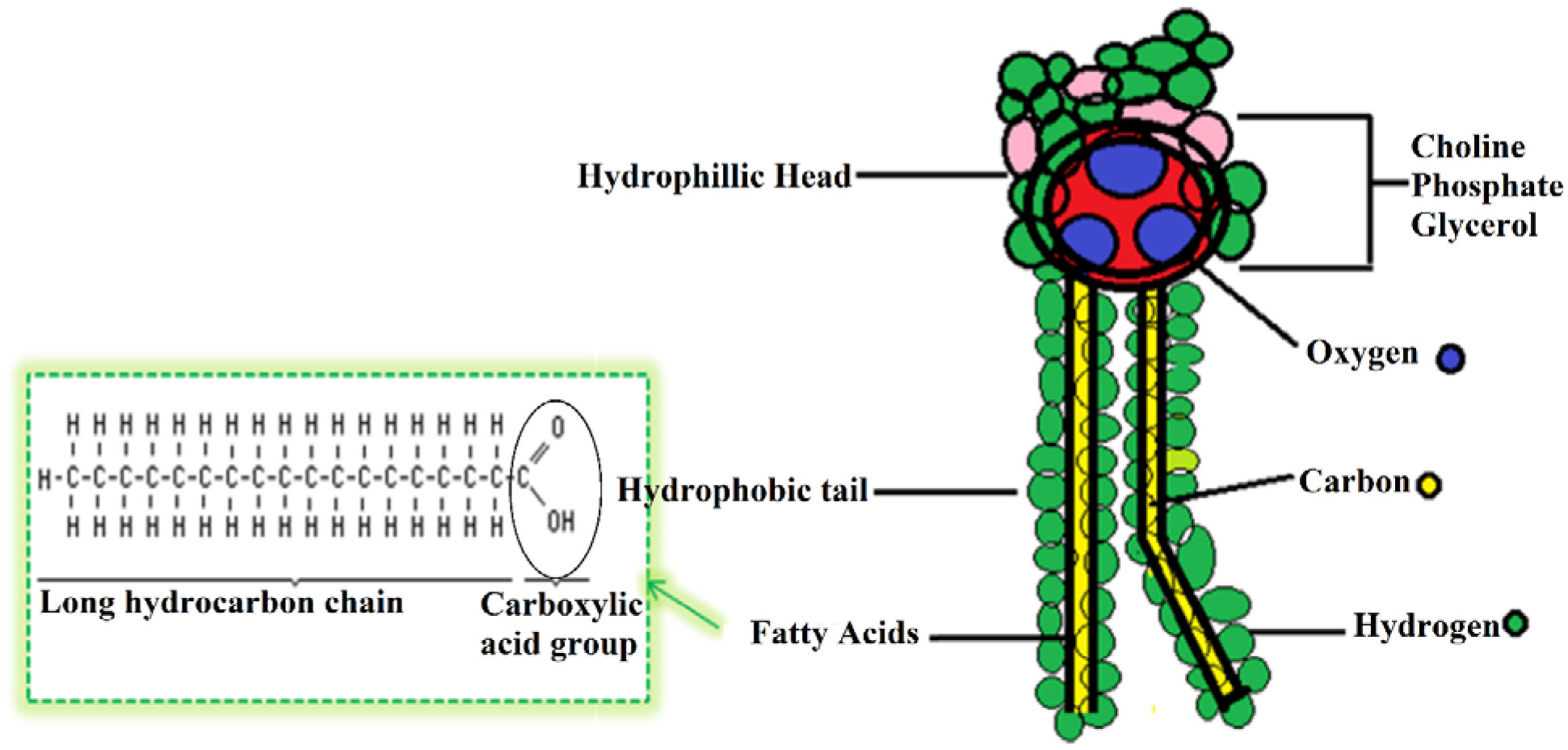
Citation: Manikandan Alagumuthu, Divakar Dahiya, Poonam Singh Nigam. Phospholipid—the dynamic structure between living and non-living world; a much obligatory supramolecule for present and future[J]. AIMS Molecular Science, 2019, 6(1): 1-19. doi: 10.3934/molsci.2019.1.1
[1] | Teresa M. Fonovich . Phospholipid synthetic and turnover pathways elicited upon exposure to different xenobiotics. AIMS Molecular Science, 2020, 7(3): 211-228. doi: 10.3934/molsci.2020010 |
[2] | Christian Carpéné, Jean Galitzky, Jean Sébastien Saulnier-Blache . Short-term and rapid effects of lysophosphatidic acid on human adipose cell lipolytic and glucose uptake activities. AIMS Molecular Science, 2016, 3(2): 222-237. doi: 10.3934/molsci.2016.2.222 |
[3] | Kenji Chiba, Yasuhiro Maeda, Noriyasu Seki, Hirotoshi Kataoka, Kunio Sugahara . Role of sphingosine 1-phosphate (S1P) and effects of fingolimod, an S1P receptor 1 functional antagonist in lymphocyte circulation and autoimmune diseases. AIMS Molecular Science, 2014, 1(4): 162-182. doi: 10.3934/molsci.2014.4.162 |
[4] | Mohamed Amine Ben Mlouka, Thomas Cousseau, Patrick Di Martino . Application of fluorescently labelled lectins for the study of polysaccharides in biofilms with a focus on biofouling of nanofiltration membranes. AIMS Molecular Science, 2016, 3(3): 338-356. doi: 10.3934/molsci.2016.3.338 |
[5] | Marcus Vinícius Dias-Souza, Arthur Azevedo Perpétuo, Gabriel Souza dos Santos, Luiz Felipe Carreiro Machado, Renan Martins dos Santos . Natural products in drug discovery: meeting the urgency for new antimicrobials for human and veterinary use. AIMS Molecular Science, 2023, 10(1): 11-21. doi: 10.3934/molsci.2023002 |
[6] | Sharmil Suganya R, Stanelybritto Maria Arul Francis, T Venugopal . Investigation of biocorrosion on mild steel in cooling tower water and its inhibition by C. sativum. AIMS Molecular Science, 2024, 11(4): 395-414. doi: 10.3934/molsci.2024024 |
[7] | Nataša Čerekovic, Palmiro Poltronieri . Plant signaling pathways activating defence response and interfering mechanisms by pathogen effectors, protein decoys and bodyguards. AIMS Molecular Science, 2017, 4(3): 370-388. doi: 10.3934/molsci.2017.3.370 |
[8] | Fohona S. Coulibaly, Danielle N. Thomas, Bi-Botti C. Youan . Anti-HIV lectins and current delivery strategies. AIMS Molecular Science, 2018, 5(1): 96-116. doi: 10.3934/molsci.2018.1.96 |
[9] | Hakuto Kageyama, Rungaroon Waditee-Sirisattha . Bioactive ultraviolet-absorbing compounds derived from cyanobacteria and microalgae. AIMS Molecular Science, 2025, 12(1): 26-31. doi: 10.3934/molsci.2025002 |
[10] | Priscilla B.S. Albuquerque, Luana C.B.B. Coelho, José A. Teixeira, Maria G. Carneiro-da-Cunha . Approaches in biotechnological applications of natural polymers. AIMS Molecular Science, 2016, 3(3): 386-425. doi: 10.3934/molsci.2016.3.386 |
As for now, the blue planet earth is unique in the universe as it harbors life. The existence of life in any other celestial object is the biggest unresolved question till-date. Whatever it may be the answer, no life exists without a boundary (i.e., the cell wall) which demarcates the surrounding environment. Ever since the first microbe appeared 4 billion years ago, bacterial population alone accounts for 5 × 1030, i.e., five million trillion. Universal rule in chemistry “Like dissolves like” is also applicable to living organisms. It refers to “polar” and “nonpolar” solvents and solutes. Organic compounds will dissolve in the organic solvent and inorganic compound in inorganic solvents. All organisms are roughly made-up of 80 percent of water, which is a universal solvent. The extracellular environment and the cytoplasm are both aqueous in nature, whereas, the membrane separating environment and cytoplasm is made-up of lipid [1],[2]. The evolutionary challenge is that this unique membrane constituted of phospholipids also acts as a regulatory membrane [3],[4]. This boundary membrane also denoted as phospholipid bilayer, plasmalemma, or plasma membrane, and cell membrane.
Phospholipid asymmetry is clearly seen in plasma membrane and membranes of cell organelles like Golgi complex, and endoplasmic reticulum. Fatty acids are one of the ubiquitous entities in eukaryotic and prokaryotic cells. They are the precursors of major building blocks such as phospholipids, sphingolipids, sterols, as secondary metabolites and signaling molecules. Due to changes in the grade of saturation of phospholipids, alterations on membrane fluidity may occur and help in the adaptation to physical changes like temperature. The degradation of fatty acids yields a high amount of ATP as well as it represents a suitable storage for energy and carbon [5]. Every single living cell has plasma membrane, which comprises of two classes of molecules, lipids and proteins. Phospholipids are the major part of lipid molecules present in the membrane and they comprise of saturated and unsaturated fatty acids. Any adjustment in the segment of cell membrane individual fatty acids may result in changes on membrane functions and properties [6].
Membranes not only help to maintain the integrity of the cell but also play a major role in survival and other physiological activities of the cell. Phospholipids have an important participation in all living cell membranes, which act as the building block of cell membranes in all organisms [7]. Lipids act as one of the most important components of the biological system [8]. They protect organisms from harsh environments by giving them specific structure and by contributing in cellular functions, through participation both in cellular and intercellular signals [9]. They participate in the transduction of biological signals across the membrane and act as signaling compounds to control vital biological processes [10].
Phospholipids can take part in crucial processes, which give structural integrity to membranes, assists in the functions of the cell to carry out metabolism-related processes. In higher organisms, all of their body organs and cells energetically retain a supply of phospholipids across the plasma membrane [11]. Phospholipids play a major role in growth and metabolism. They manage many membrane-associated activities like enzyme-catalysis, transport of solutes and receptor-mediated signaling [12]. They act as the precursor for the production of other lipid molecules [13] or saccharide macromolecules containing lipids (lipo-polysaccharides). Phospholipids help in protein alteration for membrane association and activation of molecular chaperones [14]. In recent years, phospholipids have been recognized as the intracellular messengers, which ensure that they have roles other than being structural components of membranes. Phospholipids become intriguing as they can offer the different options such as drug carriers, bio-emulsifiers, and surface-active wetting agents [15]. This review is an effort to extract out important characteristics of phospholipids, their role in microbes, plants and animals along with lipid profiling. This communication will bridge the gap in phospholipid studies.
Study of the structure of phospholipid is important because of their relevance in health sciences, development of drug delivery, polymer science, nourishment and beautifying agent and biomedical engineering etc. [16]. Phospholipids keep up the integrity of the cell or organelles by forming a semipermeable barrier that separates them from their outside surroundings [17]. The cell membrane with phospholipids is generally made-up of saturated fatty acids. These fatty acids are having dissimilar structures and less fluidity that enables them to integrate with the essential fatty acids. Phospholipid contains one saturated and one unsaturated fatty acids connected to glycerol head (Figure 1). The alcohol group present in glycerol is attached to the molecule of phosphate, which is also attached to a small molecule also containing an alcohol group, as for example the one known as choline. Phosphate group combined with glycerol make the head of phospholipid hydrophilic, while the fatty acid moieties confer hydrophobicity to the molecule [18].
Fatty acids are hydrocarbon chains of varying lengths and degrees of unsaturation (defined as the presence of double bonds), with a carboxyl group at one end and a methyl group at the other. Most naturally occurring fatty acids have an even number of carbon atoms, typically 12 to 28 [19]. Fatty acids are important sources of energy because, when metabolized, they yield large quantities of ATP [20]. Long-chain FA cannot cross the blood-brain barrier (BBB), while the short- and medium-chain FA do, which makes the latter groups a potential source of energy for the brain along with glucose and ketone bodies [21]. Fatty acids are one of the major components of glycerolipid and thus are major structural constituents of living cells [22]. Besides being major structural constituents of the cell, fatty acids and fatty acid metabolites also play roles as modulators of many signal transduction pathways [23]. Phospholipids are recognized to have amphiphilic nature as they have a polar head bunch and non-polar saturated and/or unsaturated fat tails agammaegated as a bilayer [24]. These particular properties permit phospholipids to assume an important part in the formation of phospholipids bilayer.
The head of a phospholipid is hydrophilic, while the tail is hydrophobic. In the membrane bilayer, the hydrophobic parts of the molecules are sandwiched in the middle, while the hydrophilic parts form the surface of the bilayer. Phospholipid's hydrophilic heads are present on both outer sides of the bilayer, thus attracting water present inside the cell and outside the cell. On the other hand, the hydrophobic parts of phospholipid molecules sandwich themselves inside the bilayer [25],[26]. These self-organized bilayers give shape to cells, cell organelles and maintain physiochemical properties, generating a domain inside a cell that is essential for protein interaction and functioning [27]. The differentiation in head group, aliphatic chain and alcohol groups leads to the existence of a wide variety of phospholipids. The different types of phospholipids such as soybean phosphatidyl-choline, synthetic phosphatidylcholine, and hydrogenated phosphatidylcholine are usually used in various types of formulations [28].
Phospholipid classification depends on the polar group, namely for instance serine glycerophospholipid, glycerol glycerophospholipid, ethanolamine glycerophospholipid and choline glycerophospholipid [29],[30]. Lipid head groups are substituents that join to the glycerol (Figure 2), sphingosine, or cholesterol spines of polar layer lipids. Phospholipids and glycolipids have head groups connected to the backbone through phosphodiester bond [31],[32]. The stereospecific classification of glycerol phospholipids locates the phosphate at the stereospecific numbering (Sn)-3 positions (Figure 3). Glycerol acts as the backbone for hydrophobic tail and hydrophilic head. Based on their backbone, these phospholipids are grouped into four major types as glycerophospholipid (GPL), sphingolipids (SL), phospholipids and ether phospholipids (EPL) [33],[34]. The structure of glycerophospholipid comprises of two chains (saturated and/or unsaturated) as well as a phosphorylated alcohol molecule and a glycerol backbone [35].
The hydroxyl cluster at the third carbon atom of the glycerol backbone is esterified to phosphoric functional group, which can be further esterified to the hydroxyl group of various alcohol moieties like ethanolamine, inositol, choline and serine. Phospholipids like phosphatidyl ethanolamine (PE), phosphatidylinositol (PI), phosphatidylcholine (PC), and phosphatidylserine (PS) are dominant in the cell membrane [36],[37]. Sphingolipids consist of a long-chain amino alcohol, commonly trans-1,3-dihydroxy-2-amino-4-octadecene [38]–[40]. Depending on the diversity of head groups, sphingolipids are classified into three types—sphingomyelin, cerebro sulfatide, and cerebroside [41],[42]. Phospholipids comprise polar head groups bound to ceramide or diacylglycerol. PLs are used as surface-active wetting agents and also as the coating agents on the surface of crystals to improve the hydrophilicity of hydrophobic drugs [28],[43]. Ether phospholipid contains an alkyl group linked to oxygen atoms of glycerol. These alkyl groups present in phospholipids occur naturally in O-alkyl group [44]. Ether phospholipids contain an alkyl group linked to an oxygen atom of glycerol and are mostly present in plasma and nervous tissue [45].
Asymmetrical circulation of phospholipids is generally found in the cytoplasmic layer (Figure 4). In typical cells, phospholipids are unevenly distributed in internal and external leaflets of the plasma membrane [46]–[48]. PC and sphingomyelin (SM) are present in the external leaflets, PS, and PE in the internal leaflets of the plasma membrane [49],[50]. A number of studies have revealed that the PL asymmetry plays a significant role in the cellular and biological processes. Generally, PL asymmetry plays a role in subcellular distribution of proteins (e.g., apoptosis/fusion of organelle), preserve biophysical properties of particular membrane, maintain membrane trafficking/maturing/vehicle, support cell shape and alteration of PL asymmetry can also play prominent role in initiating biological processes, for example, blood coagulation, cytokinesis, cell fusion, identifying and discarding of apoptotic cells [51]–[53]. The PL asymmetry can have other essential cellular functions that are still to be found and analyzed. Membrane lipids in eukaryotic cells also show irregular distribution between subcellular organelles and between two membrane leaflets of specific organelles [54]–[57].
Flippases are transmembrane lipid transporter proteins found in the membrane which belong to ATP-binding cassette transporters (ABC transporters) family. Figure 4 explains about how flippases hydrolyze ATP to form asymmetric phospholipid distribution among the bilayer [58]–[63]. In endoplasmic reticulum, membrane proteins make it easier the instantaneous flip-flop of lipids and permit them to equilibrate between the two membrane leaflets not depending on ATP hydrolysis [64]. This method can agammaegate given lipids in one leaflet, consequently causing an asymmetric lipid distribution over the layer. The characteristically dissimilar phospholipid arrangement of the two leaflets of a membrane bilayer is generated and maintained by bi-directional transport (flip-flop) of lipids between the leaflets [65]. Therefore, ATP-dependent flippases can preserve asymmetric lipid dispersion by moving particular lipid pumps (P-type ATPases/E1-E2 ATPases) towards or far from the cytosolic leaflet (ABC transporters). Cell apoptosis initiation activated by cytosolic calcium can break down the lipid asymmetry by the transient movement of an ATP-independent scramblase [1],[2],[66].
Phospholipids in microorganisms work as a wetting agent and thus affect the membrane fluidity in several ways [16],[68],[69]. The Corynebacterium is a genus of Gram-positive, rod-shaped bacteria, which contains phospholipids like glycerophospholipid, diphosphatidylglycerol, and phosphatidylinositol [70]. Its cell layer contains small quantities of phosphatidylethanolamine and its methylated subsidiaries (<10%) (Table 1). Corynebacterium species contain Acyl phosphatidylglycerol (APG), a rare phospholipid. APG releases three different fatty acids subsequently to the methanolysis or saponification [71]. Furthermore, a few species such as Corynebacterium equi, C. bovis, C. diphtheria, and C. xerosis, contain mannophosphoinositides. The quantitation of Corynebacterium lipid species showed that the presence of diphosphatidyl glycerol (DPG) was 1%, whereas phosphatidylglycerol was 91% [71],[72]. Corynebacterium phospholipids were recognized by the occurrence of extended chain isomer of medium chain length [73].
S.No. | Bacteria | Lipid | Amount | References |
1 | Corneybacterium (Gram-positive) | Phosphatidylethanolamine | <10% | [71] |
Diacylphosphoglycerol | 1% | |||
Phosphoglycerides | 91% | |||
2 | E. coli (Gram-negative) | Phosphatidylethanolamine | 80% | [74] |
Phosphatidylglycerol | 15% | |||
Cardiolipin | 5% | |||
3 | Pseudomonas aeruginosa (Gram-negative) | Phosphatidylethanolamine | 71.6 ± 1.4% | [75] |
Phosphatidyl glycerol | 11.8 ± 0.4% | |||
Diphosphatidyl glycerol | 0.8 ± 0.4% | |||
Phosphatidic acid | 2.4 ± 0.6% | |||
Lysophosphatidyl ethanolamine | 1.6 ± 0.3% | |||
Phosphatidylcholine | 7.9 ± 0.3% | |||
Lysophosphatidylcholine | 3.9 ± 0.7% | |||
4 | Borrelia burgdofer (Gram-positive/negative) | Phosphatidylglycerol | Nil | [77] |
Phosphatidylcholine | ||||
5 | Alcanivorax borkumensis (Gram-negative) | Ester links fatty acids | Nil | [79] |
Escherichia coli is a Gram-negative bacteria. The membrane lipids of E. coli consist of glycerophospholipids, principally phosphatidylethanolamine (80%), phosphatidylglycerol and cardiolipin. The culture in exponential phase has 15% phosphatidylglycerol and 5% cardiolipin. In the stationary phase, the cardiolipin content increases at the expense of phosphatidylglycerol [74]. Pseudomonas is a genus of Gram-negative bacteria. Phospholipids present in these bacteria are Phosphatidylethanolamine (PE), phosphatidylglycerol (PG), diphosphatidyl glycerol (DPG), phosphatidic acid (PA), lysophosphatidyl ethanolamine (LPE), phosphatidylcholine (PC), and lysophosphatidylcholine (LPC). The molar proportion of acidic and neutral phospholipid is 0.18 [75].
Borrelia burgdofer differs from numerous microbes in phospholipids content. It contains just two primary phospholipids, phosphatidylglycerol and phosphatidylcholine [76]. By default, it is Gram-negative since the saffron was used as a dye in the test, however in general rule spirochetes are not classified as either Gram-positive or Gram-negative [77]. Lysophosphatidyl ethanolamine occurs in considerable amount among a number of the marine bacteria. Some of them contain significant quantities of poly-β-hydroxybutyrate. Alcanivorax borkumensis: A marine bacterium (Gram-negative) contains phosphatidylethanolamine, phosphatidylglycerol and diphosphatidylglycerol, predominantly [78]. A. borkumensis is a hydrocarbon-degrading marine bacterium, which contains phospholipid ester-linked fatty acids [79].
Plants are one of the richest sources of lipids and phospholipids. As shown in Table 2, there are four types of major phospholipids in plants. The phospholipids play a vital role in major life activities of plants, like photosynthesis, signal transduction, membrane trafficking and growth regulation. In plants, the photosynthetic membrane contains many galactolipids such as monogalactosyl diacylglycerol (MGDG) and digalactosyl diacylglycerol (DGDG) [80]. In chloroplast, these derivatives are essential for the photosynthetic light responses. About 80% of lipids are similar to monogalactosyl diacylglycerol and digalactosyl diacylglycerol [81]. Remarkable lipid remodeling occurs and saves phosphates, which are limiting for other cellular processes. The part of galactolipids for cellular membrane remodeling under phosphate limiting deprivation leads to galactolipid synthesis, which has essential significance for the occurrence of this process in plants [82]. Phosphate limiting deprivation was a response to lipid remodeling in Arabidopsis. The plasma film is drawn as one of a few conceivable extra plastidic layers that are redesigned taking after phosphate limiting deprivation.
S. No. | Plants | Lipid% |
||||
A | B | C | D | E | ||
1 | Arabidopsis thaliana seeds | 4 | 19 | 27 | 20 | 21 |
2 | Coconut | 3 | 6 | 2 | <1 | 80 |
3 | Cocoa butter | 35 | 35 | 3 | <1 | <1 |
4 | Palm | 4 | 38 | 10 | <1 | 2 |
5 | Olive | 2 | 76 | 8 | 1 | 2 |
6 | Canola | 2 | 59 | 21 | 10 | 3 |
7 | Peanut | 2 | 48 | 34 | <1 | 6 |
8 | Sesame | 4 | 41 | 43 | <1 | <1 |
9 | Corn | 2 | 25 | 61 | 1 | <1 |
10 | Soya bean | 4 | 24 | 54 | 7 | 1 |
11 | Sunflower | 3 | 21 | 69 | <1 | <1 |
12 | Cotton seed | 2 | 18 | 54 | <1 | 2 |
13 | Safflower | 2 | 12 | 78 | <1 | 1 |
14 | Flax | 5 | 26 | 18 | 46 | <1 |
15 | Chia | 3 | 7 | 21 | 61 | <1 |
A: Monogalactosyl diacylglycerol; B: Digalactosyl diacylglycerol; C: Sulfoquinovosyl diacylglycerol; D: α-Linolenic acid; E: Others.
MGD2/MGD3 and DGD2 are the other UDP-GAL subordinate galactosyltransferases of Arabidopsis prompted taking place after phosphate limiting deprivation (Figure 5) [83]. There are two proposed pathways for the turnover of phospholipids, one of it includes PLC and the other one PLD and PAP. These two pathways does not take place simultaneously in the same cell, but rather may be enacted relying upon the tissue of the plant. Phospholipase D (PLD)-mediated phosphatidylcholine (PtdCho) hydrolysis is the major source of both DG and PtdOH during the late signaling phase [84].
Sulfolipids are a class of lipids that have sulfur as the functional group. The most widely recognized constituents of sulfolipids are sulfoquinovose, it helps to form sulfoquinovosyl diacylglycerol. In plants, sulfolipids are essential intermediates in the sulfur cycle [85]. Sulfoquinovosyl diacylglycerol is the main sulfur-containing anionic glycerolipid and it is the low-dominant segment of the lipid membrane. Around 8%–34% of polar lipids in chloroplast are characterized by sulfoquinovosyl-diacylglycerol [86].
In the plant cell, phospholipids act as second messengers. PLs are generated due to stimuli through the activation of lipid kinase. These lipid kinases are involved in membrane docking proteins, which contain the suitable lipid-binding domain i.e., throughout the signaling of lipid, a protein downstream involved in signaling cascade can move from the cytosol to membrane sites [87]. By combining signaling protein at micro-location interaction is elevated and is helpful for activating further transmission of signals [88],[89]. For example, a protein kinase could phosphorylate another kinase in the pathway or phospholipases could hydrolyze membrane lipids to deliver another round of lipid signaling. Signal transduction may inertly stimulate by expanding enzyme concentration at the docking sites yet the lipid can assume a more dynamic part by affecting a change in conformation that upgrades enzyme activity [90]. PL and their catabolites [91],[92] induce the changes in physical properties of the membrane such as raising the rate of ion flux or else decreasing the amount of ion flux, vesicle development, and membrane transportation. The PL act as plant growth regulators. There is evidence that in plant development, individual phospholipids play a major role and the most prominent of them is phosphatidic acid stress lipid signaling [93],[94].
The animal cell membrane consists of plasma membrane along with various intracellular membranes that characterize compartments with specific activation, and variation in both membrane lipids content (Table 3) [95]. Few lipids are dispersed unevenly in the plasma membrane; for example, in the red blood cell, internal membranes are enriched in phosphatidylethanolamine and phosphatidylserine, whereas external membrane mostly contain phosphatidylcholine and sphingomyelin [96]. The foremost part of an animal cell is surrounded by phospholipid bilayer with embedded cholesterol and protein molecules [97]. Cholesterol was typically discovered as scattered in differing degrees in entire cell membrane, in the unpredictable spaces between the hydrophobic tail of the membrane lipids, where it contributes to hardening and reinforcing of the membrane [98]. The fuse ring arrangement of cholesterol implies that it is more inflexible than another layer of lipids [99]. It also acts as an essential part of membrane lipids, and is the metabolic precursor of steroid hormones. During moderate temperature, cholesterol decreases the fluidity level of the membrane by diminishing the movement of phospholipid. While at low temperature, it prevents disrupting to harden the regular packing of phospholipids [100].
The protein to lipid proportion additionally varies from different bio-membranes, 80% of lipids is present in the myelin sheath that protects nerve cells, and 20% of lipids in the internal membrane of mitochondria [101]. The structure of the membrane plays a role in signal transduction and cellular recognition. For example, erythrocytes contain phosphatidylserine in the outer leaflet of the plasma membrane, which increases the cell adherence to the vascular cell wall and acts as a signal for recognition of macrophage and phagocytosis [102]. These recognition methods are generally involved in the normal process of red cell turnover in the spleen [62],[100]. A free hydroxyl (three of five) group of phosphatidylinositol can be phosphorylated in cells [103],[104]. Altogether, among distinguished phosphorylated subsidiaries of phosphatidylinositol, one of derivatives phosphatidylinositol 3,4,5-triphosphate has not been found in plant cells [36],[37]. Phosphoinositide is agammaegately denoted as inositol phospholipids. They mainly participate in signal transduction pathway, which is activated by stimuli such as growth factors, hormones, and neurotransmitters [105].
S. No. | Animal source | Lipid% [121] |
|||
A | B | C | D | ||
1 | Human erythrocyte | 80 | 77 | 20 | <4 |
2 | Mouse erythrocyte | 85 | 50 | 20 | 0 |
3 | Rat erythrocyte | 100 | 62 | 20 | 6 |
4 | Monkey erythrocyte | 82 | 67 | 13 | 0 |
5 | Human platelet | 93 | 45 | 20 | 9 |
6 | Pig platelet | 91 | 40 | 34 | 6 |
7 | Mouse erythroleukemia cell | 80 | 45 | 50 | 15 |
8 | Rabbit intestinal brush border | 63 | 32 | 34 | 44 |
9 | Rabbit kidney brush border | 80 | 35 | 23 | 15 |
10 | Rat cardiac sarcolemma | 93 | 43 | 25 | 0 |
11 | Krebs ascites cell | 47 | 52 | 45 | 19 |
12 | Bile canalicular surface | 65 | 85 | 50 | 0 |
13 | Sinusoidal surface | 65 | 85 | 55 | 0 |
A: Sphingomyelin; B: Phosphatidylcholine; C: Phosphatidylethanolamine; D: Phosphatidylserine.
Some extremophiles have the capacity to oxidize and reduce the heavy metals that are found in the environment and make utilization of them as an electron acceptor in their energy production process, which is referred as bioleaching [106]. Bioleaching is usually done under a condition where the aseptic environment is not well-maintained because of the occurrence of extremophiles usually in one or more species groups, and these associations expand their productivity during the bioleaching of mines [107]. Being an oxidizing agent, PLs play a key role in bioleaching too. In the understanding of mechanism of action of bioleaching process, it is mandatory to learn/study the membrane fluidity and its relevant structural components such as fatty acids, lipids, and protein associated structures of a bacterial species [108]. Membrane kinetic analysis largely supports in increasing the biological products, where appropriate engineering of such will favor the large yield with minimal substrate level.
Bacteria present in the soil are unimaginably delicate even to slight ecological changes. Expanded globalization has led to the diminished horticultural efficiency, adverse changes in the atmosphere, hazardous pollution and declined condition of the environment and ecosystems [109]. Soil microflora has defeated these impacts by corroding the toxins and preserving the cyclic change of the environmental substance [110]. Phospholipid fatty acid (PLFA) profiles provide sensitive reproducible measurements for characterizing the numerically prevailing part of soil microbial communities, without cultivating the organisms. The technique gives estimates of both microbial community composition and its biomass size, and results represent in situ conditions in the soil. PLFA analysis has been used to detect various environmental stresses in the soil and was found to be more discriminatory than other methods [111].
Lipids are used as carrier molecule of anticancer drugs namely paclitaxel and doxorubicin; hence, lipid components are in large demand in pharmaceutical industry. These solid lipid nanoparticles (lipid components) help in the controlled arrival of the particular drug [4]. Docosahexanoic acid (DHA) containing phospholipids find application in medicine, for example in promoting cell differentiation in leukemia [112]. The immune function was improved by using altered PL fatty acid, and the phospholipids play an important role in apoptosis [113]. Structured triacylglycerols containing medium-chain fatty acids play an important role in nitrogen balance, promoting immune function and better lipid clearance from the bloodstream [114]. Different fatty acids incorporated in PLs structure can offer intense and balanced nutrition for human beings as functional foods or pharmaceuticals.
Oxidized phospholipids are mainly utilized as biomarkers for the finding of cardiovascular illness [115]. They also agammaegate throughout the arteriosclerotic or thrombotic condition that produces an inflammatory signal. These signal transduction processes are useful tools for cardiovascular disease diagnosis [116]. Wide-range of phospholipids are worldwide being utilized as the enrolled pharmaceutical excipient, due to their physiological process, very much endured, toxicology safe and suitable for different organization of course. The composition of fatty acid and polar head group nature defines the physico-chemical properties of phospholipids. In pharmaceutical innovation, phospholipids are utilized as emulsifiers, carriers, for enhanced permeation, solubilizers, wetting and coating agents [117]. Finding the best phospholipid excipient for any medication substance, to suit its expected use and properties, requires an adequate comprehension of the properties of the phospholipids [15].
The mixtures called lecithin consist of different phospholipids extracted and purified from different sources. The phospholipids present in liquid lecithin are phosphatidic acid (PA, 5–12%), phosphatidylethanolamine (PE, 10–15%), phosphatidylinositol (PI, 10–15%) and phosphatidylcholine (PC, 14–16%). Lecithin is commonly used as a preservative in food, cosmetics, and pharmaceuticals [118],[119]. It acts as emulsifier, wetting, viscosity modifier, extrusion aid, separating agent, anti-dusting agent, and nutritional supplement. It is also utilized for assembling the biodegradable material [120]. Lecithin is also being used in textile and leather industries as calming and softening of the textile and leather fabrics. Furthermore use of lecithin helps in dispersing dyes used in coloring throughout the fabric, by immobilizing dyes within material, which encourages a controlled discharge and avoids the wastage of dyes [121].
The new era of phospholipids are yet to come because it holds the secret key to unlock novel drug delivery system, medicines, food industry, emulsifiers etc. All biological processes are directly or indirectly linked to phospholipids. Fatty acid synthesis is the most energy-intensive process in phospholipids synthesis, and these biochemical mechanisms are key elements of bacterial physiology. The research focus on fatty acid has led to introduce modifications on the chain-length and unsaturation-degree of acyl groups. Structured PLs with a defined fatty acid profile and polar head groups can be produced by enzyme-catalyzed reactions, including hydrolysis, trans-esterification, and trans-phosphatidylation that employ according to the specificities of different phospholipases and lipases. PL, in addition, has their significance in membrane fluidity kinetic studies. Creation of a new database will certainly help to study all types of phospholipids in the biological systems. Profiling of phospholipid certainly contributes to different applications in many fields, such as cosmetics, food, nutrients, and pharmaceuticals. Further research in Phospholipids would pave the way for new advancements and applications in various other fields.
[1] | López-Marqués RL, Poulsen LR, Bailly A, et al. (2014) Structure and mechanism of ATP-dependent phospholipid transporters. Biochim biophys Acta 1850: 461–475. |
[2] |
Lopez-Marques RL, Theorin L, Palmgren MG, et al. (2014) P4-ATPases: lipid flippases in the cell membrane. Pflug Arch Eur J Physiol 466: 1227–1240. doi: 10.1007/s00424-013-1363-4
![]() |
[3] |
Li J, Wang XL, Zhang T, et al. (2015) A review on phospholipids and their main applications in drug delivery system. Asian J Pharm Sci 10: 81–98. doi: 10.1016/j.ajps.2014.09.004
![]() |
[4] |
Miao J, Du YZ, Yuan H, et al. (2013) Drug resistance reversal activity of anticancer drug loaded solid lipid nanoparticles in multidrug resistant cancer cells. Colloids Surf B 110: 74–80. doi: 10.1016/j.colsurfb.2013.03.037
![]() |
[5] | Janben HJ, Steinbuchel A (2014) Fatty acid synthesis in E. coli and its applications towards the production of fatty acid-based biofuels. Biotechnol Biofuels 7: 7. |
[6] | Hussein J (2013) Cell membrane fatty acids and health. Int J Pharm Pharm Sci 5: 38–46. |
[7] |
Caforio A, Driessen AJM (2017) Archaeal phospholipids: Structural properties and biosynthesis. Biochim Biophys Acta-Mol Cell Biol Lipids 1862: 1325–1339. doi: 10.1016/j.bbalip.2016.12.006
![]() |
[8] |
Schiller J, Suss R, Arnhold J, et al. (2004) Matrix-assisted laser desorption and ionization time-of-flight (MALDI-TOF) mass spectrometry in lipid and phospholipid research. Prog Lipid Res 43: 449–488. doi: 10.1016/j.plipres.2004.08.001
![]() |
[9] |
Irvine RF, Schell MJ (2001) Back in the water: the return of the inositol phosphates. Nat Rev Mol Cell Biol 2: 327–338. doi: 10.1038/35073015
![]() |
[10] |
Hannun YA, Luberto C, Argraves KM (2001) Enzymes of sphingolipid metabolism: from modular to integrative signaling. Biochemistry 40: 4893–4903. doi: 10.1021/bi002836k
![]() |
[11] | Cooper GM (2000) The Cell: A Molecular Approach. 2Eds., Sunderland (MA): Sinauer Associates. Available from: https://www.ncbi.nlm.nih.gov/books/NBK9928/. |
[12] |
Dowhan W, Mileykovskaya E, Bogdanov M (2004) Diversity and versatility of lipid-protein interactions revealed by molecular genetic approaches. Biochim Biophys Acta 1666: 19–39. doi: 10.1016/j.bbamem.2004.04.010
![]() |
[13] | Adlercreutz P (2000) Enzymatic conversions of glycerophospholipids. In: Bornscheuer, U.T. Editor, Enzymes in Lipid Modification, Weinheim: Wiley, 292–306. |
[14] |
George CM, Gil-Soo H (2007) Regulation of phospholipid synthesis in Saccharomyces cerevisiae by zinc depletion. Biochim Biophys Acta 1771: 322–330. doi: 10.1016/j.bbalip.2006.05.006
![]() |
[15] | Perumal Chandran S, Natarajan SB, Senthil Rajan D, et al. (2014) Phospholipids as versatile polymer in drug delivery system. Int J Pharm Pharm Sci 6: 8–11. |
[16] |
Yang S, Qiao B, Lu SH, et al. (2007) Comparative lipidomics analysis of cellular development and apoptosis in two Taxus cell lines. Biochim Biophys Acta 1771: 600–612. doi: 10.1016/j.bbalip.2007.02.011
![]() |
[17] |
Mashaghi S, Jadidi T, Koenderink G, et al. (2013) Lipid nanotechnology. Int J Mol Sci 14: 4242–4282. doi: 10.3390/ijms14024242
![]() |
[18] |
van Hoogevest P, Wendel A (2014) The use of natural and synthetic phospholipids as pharmaceutical excipients. Eur J Lipid Sci Technol 116: 1088–1107. doi: 10.1002/ejlt.201400219
![]() |
[19] | Burdge GC, Calder PC (2015) Introduction to fatty acids and lipids. World Rev Nutr Diet 112: 1–16. |
[20] | Berg JM, Tymoczko JL, Stryer L (2002) Biochemistry. 5Eds. New York: W H Freeman. Available from: https://www.ncbi.nlm.nih.gov/books/NBK22581. |
[21] | Schönfeld P, Reiser G (2013) Why does brain metabolism not favor burning of fatty acids to provide energy? - Reflections on disadvantages of the use of free fatty acids as fuel for brain. J Cerebr Blood F Met 33: 1493–1499. |
[22] |
Boudière L, Michaud M, Petroutsos D, et al. (2014) Glycerolipids in photosynthesis: composition, synthesis and trafficking. Biochim Biophys Acta-Bioenerg 1837: 470–480. doi: 10.1016/j.bbabio.2013.09.007
![]() |
[23] |
Siebers M, Brands M, Wewer V, et al. (2016) Lipids in plant-microbe interactions. Biochim Biophys Acta-Mol Cell Biol Lipids 1861: 1379–1395. doi: 10.1016/j.bbalip.2016.02.021
![]() |
[24] |
Baccile N, Cuvier AS, Prévost S, et al. (2016) Self-assembly mechanism of pH-responsive glycolipids: micelles, fibers, vesicles, and bilayers. Langmuir 32: 10881–10894. doi: 10.1021/acs.langmuir.6b02337
![]() |
[25] |
Harroun TA, Heller WT, Weiss TM et al. (1999) Theoretical analysis of hydrophobic matching and membrane-mediated interactions in lipid bilayers containing gramicidin. Biophys J 76: 3176–3185. doi: 10.1016/S0006-3495(99)77469-2
![]() |
[26] |
Peterson BL, Cummings BS (2006) A review of chromatographic methods for the assessment of phospholipids in biological samples. Biomed Chromatogr 20: 227–243. doi: 10.1002/bmc.563
![]() |
[27] |
Camera E, Picardo M, Presutti C, et al. (2004) Separation and characterization of sphingoceramides by high-performance liquid chromatography–electrospray Ionization mass spectrometry. J Sep Sci 27: 971–976. doi: 10.1002/jssc.200301712
![]() |
[28] | Chung SY, Moriyama T, Uezu E, et al. (1995) Administration of phosphatidylcholine increases brain acetylcholine concentration and improves memory in mice with dementia. J Nutr 125: 1484–1489. |
[29] |
Nakamura Y (2017) Plant phospholipid diversity: emerging functions in metabolism and protein-lipid interactions. Trends Plant Sci 22: 1027–1040. doi: 10.1016/j.tplants.2017.09.002
![]() |
[30] | Bevers EM, Comfurius P, Dekkers DW, et al. (1998) Transmembrane phospholipid distribution in blood cells: control mechanisms and pathophysiological significance. Bio Chem 379: 973–986. |
[31] |
Sodt AJ, Pastor RW (2014) Molecular modeling of lipid membrane curvature induction by a peptide: more than simply shape. Biophys J 106: 1958–1969. doi: 10.1016/j.bpj.2014.02.037
![]() |
[32] |
Hama S, Ogino C, Kondo A (2015) Enzymatic synthesis and modification of structures phospholipids: recent advances in enzyme preparation and biocatalytic process. Appl Microbiol Biot 99: 7879–7891. doi: 10.1007/s00253-015-6845-1
![]() |
[33] |
Fahy E, Subramaniam S, Brown HA, et al. (2005) A comprehensive classification system for lipids. J Lipid Res 46: 839–862. doi: 10.1194/jlr.E400004-JLR200
![]() |
[34] | Hawthorne JN, Ansell GB (1982) Phospholipids: New Comprehensive Biochemistry. Amsterdam: Elsevier Biomedical Press. |
[35] | Berg JM, Tymoczko JL, Stryer L (2002) Biochemistry. 5Eds., New York: W H Freeman. Available from: https://www.ncbi.nlm.nih.gov/books/NBK22361/ |
[36] | Vance DE, Vance JE (2002) Biochemistry of Lipids, Lipoproteins, and Membranes. 4Eds., Amsterdam: Elsevier, 505–526. |
[37] |
Vance JE (2015) Phospholipid synthesis and transport in mammalian cells. Traffic 16: 1–18. doi: 10.1111/tra.12230
![]() |
[38] |
Lorant J, Alemayehu G (2010) Importance of the sphingosine base double-bond geometry for the structural and thermodynamic properties of sphingomyelin bilayers. Biophys J 99: 2957–2966. doi: 10.1016/j.bpj.2010.09.020
![]() |
[39] |
Ramstedt B, Slotte JP (2006) Sphingolipids and the formation of sterol-enriched ordered membrane domains. Biochim Biophys Acta-Biomembr 1758: 1945–1956. doi: 10.1016/j.bbamem.2006.05.020
![]() |
[40] |
Olsen I, Janzen E (2001) Sphingolipids in bacteria and fungi. Anaerobe 7: 103–112. doi: 10.1006/anae.2001.0376
![]() |
[41] |
Moskot M, Bocheńska K, Jakóbkiewicz-Banecka J, et al. (2018) Abnormal Sphingolipid World in Inflammation Specific for Lysosomal Storage Diseases and Skin Disorders. Int J Mol Sci 19: 247. doi: 10.3390/ijms19010247
![]() |
[42] | Helen JS, Sofia KM, Vassilios MK (1989) Lipid composition and structural studies on lipids from the land snail Eobania vermiculata. Naturforsch 44c: 597-608. |
[43] |
van Hoogevest P, Wendel A (2014) The use of natural and synthetic phospholipids as pharmaceutical excipients. Eur J Lipid Sci Technol 116: 1088–1107. doi: 10.1002/ejlt.201400219
![]() |
[44] |
Brites P, Waterham HR, Wander, RJA (2004) Functions and biosynthesis of plasmalogens in health and disease. Biochim Biophys Acta 1636: 219–231. doi: 10.1016/j.bbalip.2003.12.010
![]() |
[45] | Snyder F, Lee TC, Wykle RL (2002) Ether-linked lipids and their bioactive species in biochemistry of lipids, lipoproteins, and membranes. In: Vance, D.E., Vance, J.E. Editors, Biochemistry of Lipids, Lipoproteins and Membranes, 4Eds., Amsterdam: Elsevier, 233–262. |
[46] |
Li G, Kim J, Huang Z, et al. (2016) Efficient replacement of plasma membrane outer leaflet phospholipids and sphingolipids in cells with exogenous lipids. P Natl Acad Sci USA 113: 14025–14030. doi: 10.1073/pnas.1610705113
![]() |
[47] |
Montigny C, Lyons J, Champeil P, et al. (2016) On the molecular mechanism of flippase- and scramblase-mediated phospholipid transport. Biochim Biophys Acta 1861: 767–783. doi: 10.1016/j.bbalip.2015.12.020
![]() |
[48] |
Zhou Y, Wang CO, Cho K, et al. (2015) Signal transduction membrane potential modulates plasma membrane phospholipid dynamics and K-Ras signaling. Science 349: 873–876. doi: 10.1126/science.aaa5619
![]() |
[49] |
Vance JE (2008) Phosphatidylserine and phosphatidylethanolamine in mammalian cells: two metabolically related amino phospholipids. J Lipid Res 49: 1377–1387. doi: 10.1194/jlr.R700020-JLR200
![]() |
[50] |
Fadeel B, Xue D (2009) The ins and outs of phospholipid asymmetry in the plasma membrane: roles in health and disease. Criti Rev Biochem Mol Biol 44: 264–277. doi: 10.1080/10409230903193307
![]() |
[51] |
Suetsugu S, Kurisu S, Takenawa T (2014) Dynamic shaping of cellular membranes by phospholipids and membrane-deforming proteins. Physiol Rev 94: 1219–1248. doi: 10.1152/physrev.00040.2013
![]() |
[52] | Darland-Ransom M, Wang XC, Sun CL, et al. (2008) Role of C. elegans TAT-1 protein in maintaining plasma membrane phosphatidylserine asymmetry. Science 320: 528–531. |
[53] |
Mapes J, Chen YZ, Kim A, et al. (2012) CED-1, CED-7, and TTR-52 regulate surface phosphatidylserine expression on apoptotic and phagocytic cells. Curr Biol 22: 1267–1275. doi: 10.1016/j.cub.2012.05.052
![]() |
[54] |
Helen W (2015) Biological membranes. Essays Biochem 59: 43–69. doi: 10.1042/bse0590043
![]() |
[55] |
Van Meer G, Voelker DR, Feigenson GW (2008) Membrane lipids: Where they are and how they behave. Nat Rev Mol Cell Biol 9: 112–124. doi: 10.1038/nrm2330
![]() |
[56] |
Giordano F (2018) Non-vesicular lipid trafficking at the endoplasmic reticulum-mitochondria interface. Biochem Soc Trans 46: 437–452. doi: 10.1042/BST20160185
![]() |
[57] |
Panatala R, Hennrich H, Holthuis JCM (2015) Inner workings and biological impact of phospholipid flippases. J Cell Sci 128: 2021–2032. doi: 10.1242/jcs.102715
![]() |
[58] |
Sebastian TT, Baldridge RD, Xu P, et al. (2012) Phospholipid flippases: building asymmetric membranes and transport vesicles. Biochim Biophys Acta 1821: 1068–1077. doi: 10.1016/j.bbalip.2011.12.007
![]() |
[59] |
Tanaka K, Fujimura KK, Yamamoto T (2011) Functions of phospholipid flippases. J Biochem 149: 131–143. doi: 10.1093/jb/mvq140
![]() |
[60] |
Contreras FX, Sánchez-Magraner L, Alonso A, et al. (2010) Transbilayer (flip-flop) lipid motion and lipid scrambling in membranes. FEBS Lett 584: 1779–1786. doi: 10.1016/j.febslet.2009.12.049
![]() |
[61] |
Hankins HM, Baldridge RD, Xu P, et al. (2015) Role of flippases, scramblases and transfer proteins in phosphatidylserine subcellular distribution. Traffic 16: 35–47. doi: 10.1111/tra.12233
![]() |
[62] |
Lagace TA, Ridgway ND (2013) The role of phospholipids in the biological activity and structure of the endoplasmic reticulum. Biochim Biophys Acta-Mol cell Res 1833: 2499–2510. doi: 10.1016/j.bbamcr.2013.05.018
![]() |
[63] |
Pomorski TG, Holthuis JCM, Herrmann A, et al. (2004) Tracking down lipid flippases and their biological functions. J Cell Sci 117: 805–813. doi: 10.1242/jcs.01055
![]() |
[64] |
Nakao H, Ikeda K, Ishihama Y, et al. (2016) Membrane-spanning sequences in endoplasmic reticulum proteins promote phospholipid flip-flop. Biophys J 110: 2689–2697. doi: 10.1016/j.bpj.2016.05.023
![]() |
[65] |
Montigny C, Lyons J, Champeil P, et al. (2016) On the molecular mechanism of flippase- and scramblase-mediated phospholipid transport. Biochim Biophys Acta-Mol Cell Biol Lipids 1861: 767–783. doi: 10.1016/j.bbalip.2015.12.020
![]() |
[66] |
Siarheyeva A, Sharom FJ (2009) The ABC transporter MsbA interacts with lipid A and amphipathic drugs at different. Biochem J 419: 317–328. doi: 10.1042/BJ20081364
![]() |
[67] |
Casadei MA, Mañas P, Niven G, et al. (2002) Role of membrane fluidity in pressure resistance of Escherichia coli NCTC 8164. Appl Environ Microbiol 68: 5965–5972. doi: 10.1128/AEM.68.12.5965-5972.2002
![]() |
[68] |
Sajbidor J (1997) Effect of some environmental factors on the content and composition of microbial membrane lipids. Crit Rev Biotechnol 17: 87–103. doi: 10.3109/07388559709146608
![]() |
[69] |
Goldstein DB (1984) The effects of drugs on membrane fluidity. Annu Rev Pharmacol Toxicol 24: 43–64. doi: 10.1146/annurev.pa.24.040184.000355
![]() |
[70] | Begley TP, Guschina IA, Harwood JL (2008) Lipids: chemical diversity. In: Begley, T.P. Editor, Wiley Encyclopedia of Chemical Biology. |
[71] |
Yagüe G, Segovia M, Valero-Guillén PL (1997) Acyl phosphatidylglycerol: a major phospholipid of Corynebacterium amycolatum. FEMS Microbiol Lett 151: 125–130. doi: 10.1016/S0378-1097(97)00137-7
![]() |
[72] |
Mazzella N, Molinet J, Syakti AD, et al. (2004) Bacterial phospholipid molecular species analysis by ion-pair reversed-phase HPLC/ESI/MS. J Lipid Res 45: 1355–1363. doi: 10.1194/jlr.D300040-JLR200
![]() |
[73] | Niepel MTH, Wray V, Abraham WR (2006) Intraspecific variation of unusual phospholipids from Corynebacterium spp. containing a Novel Fatty acid. J Bacteriol 180: 4650–4657. |
[74] | Pluschke G, Hirota Y, Overath P (1978) Function of phospholipids in Escherichia coli. characterization of a mutant deficient in cardiolipin synthesis. J Biol Chem 253: 5048–5055. |
[75] |
Albelo ST, Domenech CE (1997) Carbons from choline present in the phospholipids of Pseudomonas aeruginosa. FEMS microbiol lett 156: 271–274. doi: 10.1111/j.1574-6968.1997.tb12739.x
![]() |
[76] |
Wang XG, Scagliotti JP, Hu LT (2004) Phospholipid synthesis in Borrelia burgdorferi BB0249 and BB0721 encode functional phosphatidylcholine synthase phosphatidylglycerol phosphate synthase proteins. Microbiol 150: 391–397. doi: 10.1099/mic.0.26752-0
![]() |
[77] |
Burgdorfer W, Barbour AG, Hayes SF, et al. (1982) Lyme disease-a tick-borne spirochetosis? Science 216: 1317–1319. doi: 10.1126/science.7043737
![]() |
[78] | Oliver JD, Colwell R (1973) Extractable lipids of Gram-negative marine bacteria: phospholipid composition. J Bacteriol 114: 897–908. |
[79] | Yakimov MM, Golyshin PN, Lang S, et al. (1998) Alcanivorax borkurnensis gen. now., sp. nov., a new, hydrocarbon-degrading and surfactant-producing marine bacterium. Int J Sys Bacteriol 48: 339–348. |
[80] |
Rottet S, Besagni C, Kessler F (2015) The role of plastoglobules in thylakoid lipid remodeling during plant development. Biochim Biophys Acta-Bioenerg 1847: 889–899. doi: 10.1016/j.bbabio.2015.02.002
![]() |
[81] |
Gao QM, Yu K, Xia Y, Shine MB, et al. (2014) Mono- and digalactosyldiacylglycerol lipids function non-redundantly to regulate systemic acquired resistance in plants. Cell Rep 9: 1681–1691. doi: 10.1016/j.celrep.2014.10.069
![]() |
[82] |
Dubots E, Botté C, Boudière L, et al. (2012) Role of phosphatidic acid in plant galactolipid synthesis. Biochimie 94: 86–93. doi: 10.1016/j.biochi.2011.03.012
![]() |
[83] |
Kobayashi K, Awai K, Nakamura M, et al. (2009) Type-B Monogalactosyl diacylglycerol synthases are involved in phosphate starvation-induced lipid remodeling, and are crucial for low-phosphate adaptation. Plant J 57: 322–331. doi: 10.1111/j.1365-313X.2008.03692.x
![]() |
[84] |
Lassègue B, Alexander RW, Clark M, et al. (1993) Phosphatidylcholine is a major source of phosphatidic acid and diacylglycerol in angiotensin II-stimulated vascular smooth-muscle cells. Biochem J 292: 509–517. doi: 10.1042/bj2920509
![]() |
[85] |
Harwood JL, Nicholls RG (1979) The plant sulpholipid-a major component of the sulphur cycle. Biochem Soc T 7: 440–447. doi: 10.1042/bst0070440
![]() |
[86] |
Joyard J, Teyssier E, Miege C, et al. (1998) The biochemical machinery of plastid envelope membranes. Plant Physiol 118: 715–723. doi: 10.1104/pp.118.3.715
![]() |
[87] |
Damnjanovic J, Iwasaki Y (2013) Phospholipase D as a catalyst: application in phospholipid synthesis, molecular structure, and protein engineering. J Biosci Bioeng 116: 271–280. doi: 10.1016/j.jbiosc.2013.03.008
![]() |
[88] |
Zhao P (2015) Phospholipase D and phosphatidic acid in plant defense response: from protein-protein and lipid-protein interaction to hormone signaling. J Exp Bot 66: 1721–1736. doi: 10.1093/jxb/eru540
![]() |
[89] | Bodin S, Giuriato S, Ragab J, et al. (2001) Production of phosphatidylinositol 3,4,5-trisphosphate and phosphatidic acid in platelet rafts: evidence for a critical role of cholesterol enriched domains in human platelet activation. Biochemistry 40, 50: 15290–15299. |
[90] |
Meijer HG, Munnik T (2003) Phospholipid-based signaling in plants. Annu Rev Plant Biol 54: 265–306. doi: 10.1146/annurev.arplant.54.031902.134748
![]() |
[91] |
Takashi T, Thomas L (2017) Intra-mitochondrial phospholipid trafficking. Biochim Biophys Acta-Mol Cell Biol Lipids 1862: 81–89. doi: 10.1016/j.bbalip.2016.08.006
![]() |
[92] |
Cowan KA (2006) Phospholipids as plant growth regulators. J Plant Growth Regul 48: 97–109. doi: 10.1007/s10725-005-5481-7
![]() |
[93] |
Munnik T (2001) Phosphatidic acid: an emerging plant lipid second messenger. Trends Plant Sci 6: 227–233. doi: 10.1016/S1360-1385(01)01918-5
![]() |
[94] |
Testerink C, Munnik T (2005) Phosphatidic acid: a multifunctional stress signaling lipid in plants. Trends Plant Sci 10: 368–375. doi: 10.1016/j.tplants.2005.06.002
![]() |
[95] | Lodish H, Berk A, Zipursky SL, et al. (2000) Molecular cell Biology. 4Eds., New York: W. H. Freeman. Available from: https://www.ncbi.nlm.nih.gov/books/NBK21583/. |
[96] |
Penno A, Hackenbroich G, Thiele C (2013) Phospholipids and lipid droplets. Biochim Biophys Acta-Mol Cell Biol Lipids 1831: 589–594. doi: 10.1016/j.bbalip.2012.12.001
![]() |
[97] |
Gupta G, Surolia A (2010) Glycosphingolipids in microdomain formation and their spatial organization. FEBS Lett 584: 1634–1641. doi: 10.1016/j.febslet.2009.11.070
![]() |
[98] | Alberts B, Johnson A, Lewis J (2002) Molecular biology of the cell. 4Eds., NewYork: Garland Science. |
[99] |
Dufourc EJ (2008) Sterols and membrane dynamics. J Chem Biol 1: 63–77. doi: 10.1007/s12154-008-0010-6
![]() |
[100] |
Singer SJ (2004) Some early history of membrane molecular biology. Annu Rev Physiol 66: 1–27. doi: 10.1146/annurev.physiol.66.032902.131835
![]() |
[101] |
Stoeckenius W, Engelman DM (1969) Current models for the structure of biological membranes. J Cell Biol 42: 613–646. doi: 10.1083/jcb.42.3.613
![]() |
[102] | Simons K, Sampaio JL (2011) Membrane organization and lipid rafts. Cold Spring Harb Perspect Biol 3: a004697. |
[103] |
Kim SH, Song HE, Kim SJ, et al. (2017) Quantitative structural characterization of phosphatidylinositol phosphates from biological samples. J Lipid Res 58: 469–478. doi: 10.1194/jlr.D069989
![]() |
[104] |
Kim Y, Shanta SR, Zhou LH, et al. (2010) Mass spectrometry-based cellular phosphoinositides profiling and phospholipid analysis: a brief review. Exp Mol Med 42: 1–11. doi: 10.3858/emm.2010.42.1.001
![]() |
[105] |
Berridge MJ (1993) Inositol trisphosphate and calcium signaling. Nature 361: 315–325. doi: 10.1038/361315a0
![]() |
[106] | Pronk JT, De BJC, Bos P (1992) Anaerobic growth of Thiobacillus ferrooxidans. Appl Environ Microbiol 58: 2227–2230. |
[107] |
Rawlings DE (2002) Heavy metal mining using microbes. Annu Rev Microbiol 56: 65–91. doi: 10.1146/annurev.micro.56.012302.161052
![]() |
[108] |
Rampelotto PH (2010) Resistance of microorganisms to extreme environmental conditions and its contribution to astrobiology. Sustainability 2: 1602–1623. doi: 10.3390/su2061602
![]() |
[109] |
Haruta S, Kanno N (2015) Survivability of microbes in natural environments and their ecological impacts. Microbes Environ 30: 123–125. doi: 10.1264/jsme2.ME3002rh
![]() |
[110] | Bloem J, de Ruiter P, Bouwman LA (1997) Soil Food webs and nutrient cycling in agroecosystems. In: van Elsas, JD., Trevors, J.T., Willington, E. Editors, Modern Soil Microbiology. New York: Marcel Dekker, 245–278. |
[111] | Kaur A, Kaur A, Choudhary R, et al. (2005) Phospholipid fatty acid-a bioindicator of environment monitoring and assessment in soil ecosystem. Curr Sci 89: 1103–1112. |
[112] | Hosokawa M, Minami K, Kohno H, et al. (1999) Differentiation- and apoptosis-inducing activities of phospholipids containing docosahexaenoic acid for mouse myeloid leukemia M1 cells. Fish Sci 65: 789–799. |
[113] |
Chaurio RA, Janko C, Muñoz LE, et al. (2009) Phospholipids: key players in apoptosis and immune regulation. Molecules 14: 4892–4914. doi: 10.3390/molecules14124892
![]() |
[114] | Hoy CE, Xu X (2001) Structured triacylglycerols. In: Gunstone FD. Editor, Structured and Modified Lipid. New York: Marcel Dekker, 209–240. |
[115] |
Willers C, Jansen VRPJ, Claassens S (2015) Phospholipid fatty acid profiling of microbial communities-a review of interpretations and recent applications. J App Microbiol 119: 1207–1218. doi: 10.1111/jam.12902
![]() |
[116] | Ashraf MZ, Kar NS, Podrez EA (2009) Oxidized phospholipids: biomarker for cardiovascular diseases. Int J Biochem Cell B 4: 1241–1244. |
[117] |
van Hoogevest P, Wendel A (2014) The use of natural and synthetic phospholipids as pharmaceutical excipients. Eur J Lipid Sci Tech 116: 1088–1107. doi: 10.1002/ejlt.201400219
![]() |
[118] | Diehl BWK, Ockels W (1995) Phospholipids: characterization, metabolism, and novel biological applications. In: Cevc, G., Paltauf, F. Editors, Proceedings of the 6th International Colloquium, USA: AOCS Press, 29–32. |
[119] |
Descalzo AM, Insani EM, Pense NA (2003) Light-scattering detection of phospholipids resolved by HPLC. Lipids 38: 999–1003. doi: 10.1007/s11745-003-1154-1
![]() |
[120] | Campbell NA, Williamson B, Heyden RJ (2015) Biology Exploring Life. Boston, Massachusetts: Pearson Prentice Hall. |
[121] |
Zachowski A (1993) Phospholipids in animal eukaryotic membranes: transverse asymmetry and movement. Biochem J 294: 1–14. doi: 10.1042/bj2940001
![]() |
1. | Caroline H. Knittel, Neal K. Devaraj, Bioconjugation Strategies for Revealing the Roles of Lipids in Living Cells, 2022, 55, 0001-4842, 3099, 10.1021/acs.accounts.2c00511 | |
2. | Kira L. F. Hilton, Chandni Manwani, Jessica E. Boles, Lisa J. White, Sena Ozturk, Michelle D. Garrett, Jennifer R. Hiscock, The phospholipid membrane compositions of bacterial cells, cancer cell lines and biological samples from cancer patients, 2021, 12, 2041-6520, 13273, 10.1039/D1SC03597E | |
3. | Dalia Santos-Luna, Yudibeth Sixto-López, Diego Bravo-Alfaro, Cynthia Cano-Sarmiento, Hugo García, José Correa-Basurto, Design and simulation of a caprylic acid enzymatically modified phosphatidylcholine micelle using a coarse-grained molecular dynamics simulations approach, 2023, 0739-1102, 1, 10.1080/07391102.2023.2180434 | |
4. | Dominik Wupperfeld, Gert Fricker, Béatrice Bois De Fer, Larissa Frank, Annika Wehrle, Branko Popovic, Essential phospholipids decrease apoptosis and increase membrane transport in human hepatocyte cell lines, 2022, 21, 1476-511X, 10.1186/s12944-022-01698-8 | |
5. | Souvik Mukerjee, Abdulaziz S. Saeedan, Mohd. Nazam Ansari, Manjari Singh, Polyunsaturated Fatty Acids Mediated Regulation of Membrane Biochemistry and Tumor Cell Membrane Integrity, 2021, 11, 2077-0375, 479, 10.3390/membranes11070479 | |
6. | Neven Žarković, Biserka Orehovec, Bruno Baršić, Marko Tarle, Marta Kmet, Ivica Lukšić, Franz Tatzber, Willibald Wonisch, Elżbieta Skrzydlewska, Wojciech Łuczaj, Lipidomics Revealed Plasma Phospholipid Profile Differences between Deceased and Recovered COVID-19 Patients, 2022, 12, 2218-273X, 1488, 10.3390/biom12101488 | |
7. | Ashley B. Carey, Alex Ashenden, Ingo Köper, Model architectures for bacterial membranes, 2022, 14, 1867-2450, 111, 10.1007/s12551-021-00913-7 | |
8. | Elias Gizaw Mernie, Mei‐Chun Tseng, Wen‐Ti Wu, Tzu‐Ming Liu, Yu‐Ju Chen, Nanoprobe‐based mass spectrometry and Fourier transform infrared spectroscopy for rapid phospholipid profiling, 2022, 69, 0009-4536, 94, 10.1002/jccs.202100284 | |
9. | N. A. Ishutina, I. A. Аndrievskaya, I. A. Sinyakin, Peculiarities of phospholipiid changes in erythrocyte membranes in parturient women with COVID-19-associated community-acquired pneumonia, 2023, 1998-5029, 83, 10.36604/1998-5029-2023-87-83-89 | |
10. | Telli Alia, 2023, 9789815123494, 118, 10.2174/9789815123494123010007 | |
11. | Jyoti Sankar Prusty, Ashwini Kumar, Awanish Kumar, Anti-fungal peptides: an emerging category with enthralling therapeutic prospects in the treatment of candidiasis, 2024, 1040-841X, 1, 10.1080/1040841X.2024.2418125 | |
12. | Wojciech Łuczaj, Anna Moniuszko-Malinowska, Monika Groth, Elżbieta Skrzydlewska, Changes in the serum phospholipid profile of neuroborreliosis patients, foresters, and patients subjected to long-term therapy according to ILADS methods, 2025, 10988823, 106966, 10.1016/j.prostaglandins.2025.106966 |
S.No. | Bacteria | Lipid | Amount | References |
1 | Corneybacterium (Gram-positive) | Phosphatidylethanolamine | <10% | [71] |
Diacylphosphoglycerol | 1% | |||
Phosphoglycerides | 91% | |||
2 | E. coli (Gram-negative) | Phosphatidylethanolamine | 80% | [74] |
Phosphatidylglycerol | 15% | |||
Cardiolipin | 5% | |||
3 | Pseudomonas aeruginosa (Gram-negative) | Phosphatidylethanolamine | 71.6 ± 1.4% | [75] |
Phosphatidyl glycerol | 11.8 ± 0.4% | |||
Diphosphatidyl glycerol | 0.8 ± 0.4% | |||
Phosphatidic acid | 2.4 ± 0.6% | |||
Lysophosphatidyl ethanolamine | 1.6 ± 0.3% | |||
Phosphatidylcholine | 7.9 ± 0.3% | |||
Lysophosphatidylcholine | 3.9 ± 0.7% | |||
4 | Borrelia burgdofer (Gram-positive/negative) | Phosphatidylglycerol | Nil | [77] |
Phosphatidylcholine | ||||
5 | Alcanivorax borkumensis (Gram-negative) | Ester links fatty acids | Nil | [79] |
S. No. | Plants | Lipid% |
||||
A | B | C | D | E | ||
1 | Arabidopsis thaliana seeds | 4 | 19 | 27 | 20 | 21 |
2 | Coconut | 3 | 6 | 2 | <1 | 80 |
3 | Cocoa butter | 35 | 35 | 3 | <1 | <1 |
4 | Palm | 4 | 38 | 10 | <1 | 2 |
5 | Olive | 2 | 76 | 8 | 1 | 2 |
6 | Canola | 2 | 59 | 21 | 10 | 3 |
7 | Peanut | 2 | 48 | 34 | <1 | 6 |
8 | Sesame | 4 | 41 | 43 | <1 | <1 |
9 | Corn | 2 | 25 | 61 | 1 | <1 |
10 | Soya bean | 4 | 24 | 54 | 7 | 1 |
11 | Sunflower | 3 | 21 | 69 | <1 | <1 |
12 | Cotton seed | 2 | 18 | 54 | <1 | 2 |
13 | Safflower | 2 | 12 | 78 | <1 | 1 |
14 | Flax | 5 | 26 | 18 | 46 | <1 |
15 | Chia | 3 | 7 | 21 | 61 | <1 |
A: Monogalactosyl diacylglycerol; B: Digalactosyl diacylglycerol; C: Sulfoquinovosyl diacylglycerol; D: α-Linolenic acid; E: Others.
S. No. | Animal source | Lipid% [121] |
|||
A | B | C | D | ||
1 | Human erythrocyte | 80 | 77 | 20 | <4 |
2 | Mouse erythrocyte | 85 | 50 | 20 | 0 |
3 | Rat erythrocyte | 100 | 62 | 20 | 6 |
4 | Monkey erythrocyte | 82 | 67 | 13 | 0 |
5 | Human platelet | 93 | 45 | 20 | 9 |
6 | Pig platelet | 91 | 40 | 34 | 6 |
7 | Mouse erythroleukemia cell | 80 | 45 | 50 | 15 |
8 | Rabbit intestinal brush border | 63 | 32 | 34 | 44 |
9 | Rabbit kidney brush border | 80 | 35 | 23 | 15 |
10 | Rat cardiac sarcolemma | 93 | 43 | 25 | 0 |
11 | Krebs ascites cell | 47 | 52 | 45 | 19 |
12 | Bile canalicular surface | 65 | 85 | 50 | 0 |
13 | Sinusoidal surface | 65 | 85 | 55 | 0 |
A: Sphingomyelin; B: Phosphatidylcholine; C: Phosphatidylethanolamine; D: Phosphatidylserine.
S.No. | Bacteria | Lipid | Amount | References |
1 | Corneybacterium (Gram-positive) | Phosphatidylethanolamine | <10% | [71] |
Diacylphosphoglycerol | 1% | |||
Phosphoglycerides | 91% | |||
2 | E. coli (Gram-negative) | Phosphatidylethanolamine | 80% | [74] |
Phosphatidylglycerol | 15% | |||
Cardiolipin | 5% | |||
3 | Pseudomonas aeruginosa (Gram-negative) | Phosphatidylethanolamine | 71.6 ± 1.4% | [75] |
Phosphatidyl glycerol | 11.8 ± 0.4% | |||
Diphosphatidyl glycerol | 0.8 ± 0.4% | |||
Phosphatidic acid | 2.4 ± 0.6% | |||
Lysophosphatidyl ethanolamine | 1.6 ± 0.3% | |||
Phosphatidylcholine | 7.9 ± 0.3% | |||
Lysophosphatidylcholine | 3.9 ± 0.7% | |||
4 | Borrelia burgdofer (Gram-positive/negative) | Phosphatidylglycerol | Nil | [77] |
Phosphatidylcholine | ||||
5 | Alcanivorax borkumensis (Gram-negative) | Ester links fatty acids | Nil | [79] |
S. No. | Plants | Lipid% |
||||
A | B | C | D | E | ||
1 | Arabidopsis thaliana seeds | 4 | 19 | 27 | 20 | 21 |
2 | Coconut | 3 | 6 | 2 | <1 | 80 |
3 | Cocoa butter | 35 | 35 | 3 | <1 | <1 |
4 | Palm | 4 | 38 | 10 | <1 | 2 |
5 | Olive | 2 | 76 | 8 | 1 | 2 |
6 | Canola | 2 | 59 | 21 | 10 | 3 |
7 | Peanut | 2 | 48 | 34 | <1 | 6 |
8 | Sesame | 4 | 41 | 43 | <1 | <1 |
9 | Corn | 2 | 25 | 61 | 1 | <1 |
10 | Soya bean | 4 | 24 | 54 | 7 | 1 |
11 | Sunflower | 3 | 21 | 69 | <1 | <1 |
12 | Cotton seed | 2 | 18 | 54 | <1 | 2 |
13 | Safflower | 2 | 12 | 78 | <1 | 1 |
14 | Flax | 5 | 26 | 18 | 46 | <1 |
15 | Chia | 3 | 7 | 21 | 61 | <1 |
S. No. | Animal source | Lipid% [121] |
|||
A | B | C | D | ||
1 | Human erythrocyte | 80 | 77 | 20 | <4 |
2 | Mouse erythrocyte | 85 | 50 | 20 | 0 |
3 | Rat erythrocyte | 100 | 62 | 20 | 6 |
4 | Monkey erythrocyte | 82 | 67 | 13 | 0 |
5 | Human platelet | 93 | 45 | 20 | 9 |
6 | Pig platelet | 91 | 40 | 34 | 6 |
7 | Mouse erythroleukemia cell | 80 | 45 | 50 | 15 |
8 | Rabbit intestinal brush border | 63 | 32 | 34 | 44 |
9 | Rabbit kidney brush border | 80 | 35 | 23 | 15 |
10 | Rat cardiac sarcolemma | 93 | 43 | 25 | 0 |
11 | Krebs ascites cell | 47 | 52 | 45 | 19 |
12 | Bile canalicular surface | 65 | 85 | 50 | 0 |
13 | Sinusoidal surface | 65 | 85 | 55 | 0 |