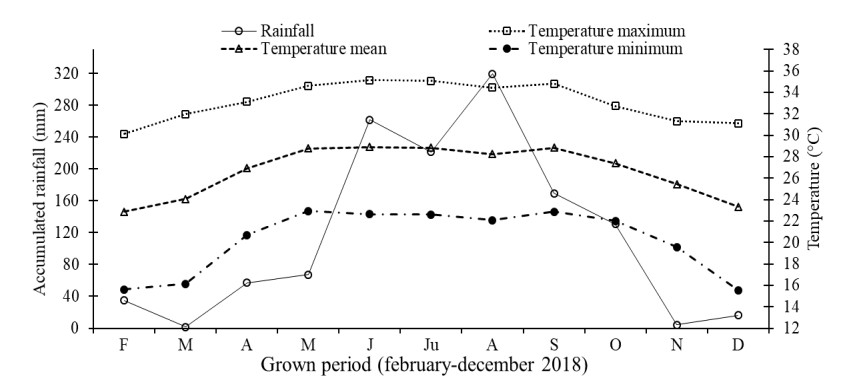
Citation: José Luis Del Rosario-Arellano, Isaac Meneses-Márquez, Otto Raúl Leyva-Ovalle, Noé Aguilar-Rivera, Gloria Ivette Bolio-López, Pablo Andrés-Meza. Morphoagronomic and industrial performance of cassava (Manihot esculenta Crantz) germplasm for the production of starch and solid byproducts[J]. AIMS Agriculture and Food, 2020, 5(4): 617-634. doi: 10.3934/agrfood.2020.4.617
[1] | Eric Tzyy Jiann Chong, Lucky Poh Wah Goh, Mariam Abd. Latip, Zaleha Abdul Aziz, Noumie Surugau, Ping-Chin Lee . Genetic diversity of upland traditional rice varieties in Malaysian Borneo based on mitochondrial cytochrome c oxidase 3 gene analysis. AIMS Agriculture and Food, 2021, 6(1): 235-246. doi: 10.3934/agrfood.2021015 |
[2] | Heru Irianto, Mujiyo Mujiyo, Aulia Qonita, Ato Sulistyo, Erlyna Wida Riptanti . The development of jarak towo cassava as a high economical raw material in sustainability-based food processing industry. AIMS Agriculture and Food, 2021, 6(1): 125-141. doi: 10.3934/agrfood.2021008 |
[3] | Avraam Koskosidis, Ebrahim M. Khah, Ourania I. Pavli, Dimitrios N. Vlachostergios . Effect of storage conditions on seed quality of soybean (Glycine max L.) germplasm. AIMS Agriculture and Food, 2022, 7(2): 387-402. doi: 10.3934/agrfood.2022025 |
[4] | Pattarabhorn Pakaweerachat, Worasaung Klinthong, Kazuhisa Ohtaguchi, Teerin Chysirichote . Simultaneous isoquercitin and gallic acid production of Aspergillus niger on Triphala byproduct under solid state fermentation in packed-bed bioreactor. AIMS Agriculture and Food, 2023, 8(2): 359-373. doi: 10.3934/agrfood.2023020 |
[5] | Anthony Temitope Idowu, Oluwakemi Osarumwense Igiehon, Ademola Ezekiel Adekoya, Solomon Idowu . Dates palm fruits: A review of their nutritional components, bioactivities and functional food applications. AIMS Agriculture and Food, 2020, 5(4): 734-755. doi: 10.3934/agrfood.2020.4.734 |
[6] | John Samelis, Athanasia Kakouri . Major technological differences between an industrial-type and five artisan-type Greek PDO Galotyri market cheeses as revealed by great variations in their lactic acid microbiota. AIMS Agriculture and Food, 2019, 4(3): 685-710. doi: 10.3934/agrfood.2019.3.685 |
[7] | Sangam Dahal, Basanta Kumar Rai, Anish Dangal, Kishor Rai, Prekshya Timsina, Ramesh Koirala, Sanjay Chaudhary, Pankaj Dahal, Tanka Bhattarai, Angelo Maria Giuffrè . Evaluation of storage stability of refrigerated buffalo meat coated with hydrothermally treated potato starch incorporated with thyme (Thymus vulgaris) and ginger (Zingiber officinale) essential oil. AIMS Agriculture and Food, 2024, 9(4): 1110-1133. doi: 10.3934/agrfood.2024058 |
[8] | Johan Sukweenadhi, Eloqui Viectorica Wiranata, Ida Bagus Made Artadana, Kang-Se Chang . Isolation and in vitro screening of plant growth promoting bacteria from rhizosphere and root tissues of potato tuber (Solanum tuberosum L.). AIMS Agriculture and Food, 2023, 8(4): 1028-1037. doi: 10.3934/agrfood.2023055 |
[9] | María Cámara-Ruiz, José María García Beltrán, Francisco Antonio Guardiola, María Ángeles Esteban . In vitro and in vivo effects of purslane (Portulaca oleracea L.) on gilthead seabream (Sparus aurata L.). AIMS Agriculture and Food, 2020, 5(4): 799-824. doi: 10.3934/agrfood.2020.4.799 |
[10] | Nelson Mpumi, Revocatus L. Machunda, Kelvin M. Mtei, Patrick A. Ndakidemi . Insecticidal Efficacy of Syzygium aromaticum, Tephrosia vogelii and Croton dichogamus Extracts against Plutella xylostella and Trichoplusia ni on Brassica oleracea crop in Northern Tanzania. AIMS Agriculture and Food, 2021, 6(1): 185-202. doi: 10.3934/agrfood.2021012 |
Cassava (Manihot esculenta Crantz) is one of 100 species of trees, shrubs, and weeds of the Manihot genus, distributed in tropical and subtropical zones [1]. In these areas, its starch rich storage roots [2,3,4] are considered the main source of calories and part of food sovereignty for more than 800 million people around the world [5].
Furthermore, cassava could contribute to rural industrial development [6,7], since its starch shows potential for the development of products with high added value in alimentary and non-alimentary industrial applications like pharmaceutical products, paper, fabrics, sweeteners, animal feed, biofuels [1,5], and even the manufacture of bioplastics [8,9,10,11]. Regarding the aforementioned, the preference for cassava starch is because it is an inexpensive and easy to extract raw material. Moreover, its properties are equal or superior to those of starch from maize, wheat, and rice; these factors make it a choice ingredient for industry, especially for small businesses with little capital [12]. Another of its advantages is that the plants can be grown under adverse climatic and soil conditions [13,14]. Its yield can be potentialized [15,16,17] in places with adequate rainfall conditions, good soil fertility levels, optimum crop management [18], and use of highly productive clones [19,20]. Regarding this last aspect, it is important to learn their agronomical, technological, and even culinary attributes, as these influence their selection and adoption by farmers [16,21,22]. In the evaluation of brazilian elite germplasm, clones less than 3 m high, height at the first branch > 0.6 m, high productivity of fresh roots (>30 t ha−1) and starch (24.17 to 30.94%) were found; these are considered important characteristics for the selection of outstanding materials [23]. Meanwhile, Peprah et al. [24], found a positive association between the fresh roots yield with the number of total roots, biomass, and harvest index in elite and local germplasm of Ghana; also, the dry matter content with starch yield. In this regard, the content of dry matter and starch are considered quality factors, which tend to affect the yield in the industrial manufacture of cassava [25,26].
Nevertheless, industries based on cassava generate a high amount of lignocellulosic byproducts [27,28,29] with incorrect disposal creates severe public health issues, while their disuse results in economic losses and depletion of natural resources [30]. For example, in the production of 1 t of cassava starch, 2.5 t of bagasse are produced [31], a lignocellulosic byproduct [32] with a high amount of carbohydrates (82.6%), essentially from residual starch [33,29]; in addition to 100 to 200 kg of peels per ton of processed cassava [34], and a high amount of wastewater [35]. Hence, the assessment of byproducts has raised a lot of interest [36], since thanks to advancements in industrial biotechnology, these agro-industrial byproducts have potential for economic use [37].
In Mexico, cassava has a yield of 12.58 t ha−1 [38], being mainly used as a food source. Moreover, it is underexploited, being grown in a traditional fashion and mostly in family orchards [39,40,41,42]. Also, given the great diversity of traditional clones in the country [43,44], with specific characteristics such as plant height, stem diameter, leaf dimensions, weight of roots per plant, field yield of fresh roots, and starch content [45], it is relevant to explore native germplasm to evidence its morphoagronomic and industrial performance. This work would help to protect, value, boost, and foster the incorporation of cassava into production systems.
This research was carried out in the Cotaxtla Experimental Field of the National Institute of Forestry, Agricultural, and Livestock Research (NIFALR), Veracruz, Mexico (18°56’11.28” N; 96°11’49.53” W, altitude 14 masl). Its historical (1986-2018) climatic data are mean annual maximum, mean, and minimum temperatures of 31.15 ℃, 25.11 ℃, and 19.07 ℃, respectively; rainfall of 1,453.91 mm [46]; and subhumid warm climate (Aw2), according to the Köppen climate classification modified by García [47]. The soil representing the experimental area has sandy loam texture, having moderately acidic pH (5.8), moderately low organic matter content (12.0 g kg−1), low N content (11.0 mg kg−1), high P and K contents available (132.8 and 139.0 mg kg−1, respectively), very high exchangeable Ca (1,800 mg kg−1) with suitable Mg content (191.0 mg kg−1), and small amounts of micronutrient.
The climatic conditions such as monthly mean temperature, maximum, minimum; and accumulated monthly rainfall of the cultivation cycle (300 days) were recorded through the El Tejar meteorological station, Medellin de Bravo, Veracruz (19°2’30.00” N; 96°8’26.99” W), located 15.45 km from the experimental site [46].
Three clones were evaluated: MMEXV5, MMEXV40 and MMEXCH23, safeguarded in the Cassava Germplasm Bank of NIFALR, based on their high starch and dry matter content, according to previous characterization data [44,49] obtained through the gravimetric method [50]. According to the phenotype of the clones, they showed a mean of seven lobes per leaf and two main stems; two branching levels in MMEXV40 (open shaped and tetrachotomic) and MMEXCH23 (umbrella shaped and trichotomic), while MMEXV5 showed erect growth (cylindrical shaped).
To prepare the soil, weeds were removed by hand; it was ploughed once, crossed and rowed mechanically. Planting was done on February 13th, 2018; consisting of 20 cm long cuttings. Also, to ensure the phytosanity of the agronomic seed, the cuttings were submerged in a chemical treatment consisting of a Benomyl solution at a ratio of 2 g L−1 water ((Benomyl: Methyl-1-(butyl carbamoyl) benzimidazole-2-ylcarbamate)) and Cypermethrin with 4 mL L−1 water ((Cypermethrin: alpha-Cyano (3-phenoxybenzyl (+) Cis trans-3-(2, 2-dichlorovinyl)-2, 2-dimethyl cyclopropane carboxylate)). Field-trials were established in a randomized complete block design with three replicates, each consisting of five rows of six plants, giving a plot size of 30 plants. Planting was done at a spacing of 1 × 1 m. Weeds were controlled manually every month during the crop growth cycle. The plants were monitored every 15 days to detect the presence of pests and diseases. An amino acid, Ca, B, and Zn based foliar fertilizer was applied at a dose of 2.2 mL L−1 water at 30, 60, and 150 days after planting (dap), while soil fertilization was applied with 20-20-20 (NPK) fertilizer at 75 dap.
Morphoagronomic and industrial traits were recorded at 300 dap, time when the roots are usually harvested [1,26,51].
Three healthy plants were randomly selected from the central part of each experimental unit. For each plant, we registered: length and width of the central lobe of the leaf (LLL and LLW, cm), this located in the middle part of the plant; the diameter of the stem (STDIA, cm), at 2 cm from the soil surface; height, from the soil surface to the last leaf developed (PH, m), as well as the number of total roots (NTR) and commercial roots (NCR) were counted [50]. Lastly, fresh storage roots with no peduncle were washed, dried, and weighed (Torrey L-PCR 40 scale) to report root yield (RY, t ha−1).
The fresh storage roots were immediately transported to the Laboratory of General Uses of the School of Biological and Agriculture-Livestock Sciences, from the Veracruz University. To register the yield of starch extraction and related byproducts (completely randomized design), we used the method proposed by López et al. [52] and Vargas et al. [53] with some modifications. First, the storage roots were submerged in a NaClO solution (250 ppm L−1) for 10 minutes. To determine dry matter (DM), 100 g of thin slices were cut from the midlle section of two random storage roots per plant and dried at 60 ℃ for 72 hours in an oven (Ecoshell PCD 2000 serials), measuring until it reached a constant weight. The remaining storage roots were peeled and both the pulp (PUL) and peels (PE) were weighed. Subsequently, 1 kg of pulp was taken, cut into small pieces, and ground in a juice extractor (Haus, model: 74.20304) until a white viscous liquid was obtained, with a fibrous fraction or bagasse as byproduct. The liquid was filtered through cloth and 500 mL distilled water was added and shaken. Then, it was left to settle for three hours and finally, it was decanted to obtain a starch paste (this procedure was repeated three times). The fresh bagasse (FBAG) was weighed and then, to avoid starch loss, it was wrung to later be subjected to the previously described procedure. Both samples, having been decanted separately, were placed in an oven (Ecoshell PCD 2000 serials) at 50 ℃ for 24 h. Subsequently, we determined the starch extraction yield from the pulp (SPUL), the bagasse (SBAG), and total starch (STL = SPUL + SBAG). Finally, the fresh bagasse was dried at 50 ℃ for 72 h [54] to register its weight (DBAG). All the traits were weighed on a scale (Brainweigh B® 3000D). The values of traits DM, PUL, and PE were registered as a percentage of the fresh storage root weight; and the values of FBAG, DBAG, SPUL, SBAG, and STL were registered as percentages based on 1 kg of pulp.
The traits were subjected to verification of assumptions of normality and homogeneity through the Shapiro-Wills and Leven tests, respectively. An analysis of variance was done as well as a Tukey mean comparison test (p ≤ 0.05). To learn the correspondence between morphoagronomic and agro-industrial traits and the clones, a PCA was done through the correlation matrix of the original traits. The eigenvalues, eigenvectors, and total accumulated variance were considered. Subsequently, the values were graphed in a two-dimensional plane using the R Studio v3.5.2 software [55].
The climatic conditions were favorable for the development of the crop (Figure 1), since there was an average maximum, mean, and minimum temperature of 33.12, 26.68, and 20.24 ℃, respectively; and a total accumulated rainfall of 1283.20 mm [1,56]. The location was characterized for a dry period, with a constant increase in temperature and decrease in rainfall from the time of planting until May, followed by a wet period from June to October. It is worth mentioning that cassava plants subjected to water stress decrease their physiological activity, consequently decreasing yield [57], especially when the drought period coincides with root tuberization and thickening [58], particularly between 60 and 90 dap [26,59,60]. Therefore, surface irrigation was performed 60 and 75 dap. Although cassava can withstand dry periods [17], these foster the appearance of economically important pests [61,62]. With regard to this, we detected the presence of thrips (Frankliniella spp.); this pest was controlled through the application of Abamectin (0.55 mL L−1 water ((Abamectin (avermectin)) and Cypermethrin.
Statistical assumptions were fulfilled. On the other hand, the ANOVA showed differences in the morphoagronomic traits STDIA, PH, and RY (p ≤ 0.01) (Figure 2). STDIA values of 4.55 to 5.57 cm were recorded, with the largest dimension for the MMEXV40 clone (Figure 2a). These values are considered high, since stem diameters of between 2 and 6 cm have been reported [63]. The notable development of STDIA is attributed partly to the adecuate edaphoclimatic conditions present at the study site. In this regard, the optimal conditions for the development of cassava are between 20 and 30 ℃, while areas with < 500 mm cause drought stress. Cassava plants prefer soils with medium and light texture, features that facilitate drainage. They tolerate acidic soils, high levels of interchangeable Al and low levels of P [56,64]. In addition, they respond well to the application of external nutrients, as low soil fertility is one of the limitations in the production of cassava [15,65,66,67]. In turn, low rainfall (281.51 to 872 mm) negatively affects growth [68,69], as a result of stomatal closure, which decreases the CO2 concentration in leaves and consequently affects the photosynthesis process and translocation of photo-assimilates to growth organs [57]. In turn, the low availability of water decreases nutrient transportation and cell growth and multiplication [70].
In the case of PH, clone MMEXV5, with an erect branching habit, registered the highest value at 4.79 m, compared with the branching clones (Figure 2b). These plants of erect habit are preferred for their ease of agronomic management, seed production, ease of harvest, and the possibility to be associated with other crops [26,71]. One advantage to the use of erect branching clones is to increase planting density, decreasing the planting framework from 90 × 90 to 75 × 75 cm [16,72]; however, an efficient study of this agronomic practice is necessary, since an increase in density could decrease the number of storage roots due to competition between plants [73,74]. To this regard, a decrease of 67 and 73% in the total storage roots production was caused when the population density increased from 5,000 to 20,000 plants per ha−1 [51]. Furthermore, environmental conditions and crop management affect this feature. Tall plants have been observed in zones with a mean temperature of 27 ℃ [75] and optimum water management to supply water demand [76,77], as well as the use of fertilizers [18,78]. On the other hand, shorter plants, like MMEXV40 and MMEXCH23 (<3.00 m), are useful in regions with strong winds [73].
Fresh storage roots yield (Figure 2c) is considered the most important trait for selection, empowerment, and conservation of traditional clones by farmers [16,19,79]. In this regard, the MMEXV5 clone reached the greatest value with 41.24 t ha−1, while the MMEXCH23 clone achieved lower production (15.85 t ha−1). The MMEXV5 clone performance was similar to the Tambou clone with 46.23 t ha−1 under the same period of growth and timely weed management [80], even higher than the yield of 25 cassava clones (1.79 to 31.79 t ha−1) grown on the same soil texture, fertilized with low and high N levels (0 and 125 kg N ha−1, respectively) in two growth cycles [81]; likewise, to the yield of 8 to 24.8 t ha−1, reached by 12 genotypes (native, improved, and new genotypes originated by line crosses) subjected to a density of 10,000 plants per ha-1, and harvested at 270 dap [82]. Therefore, there is highly productive germplasm in Mexico, since the national average is 12.58 t ha −1 [39].
The analysis of variance showed differences (p ≤ 0.05) in the agro-industrial traits: PE, PUL, FBAG, DBAG, SPUL, SBAG, and STL (Table 1). In cassava crops, pulp is considered the most economically relevant issue. In this research work, high PUL percentages were found in the MMEXV5 and MMEXV40 clones, with 80.58 and 80.12%, respectively, which is considered a characteristic value for the species [63,83].
Source of variation | Clones | ||
MMEXV5 | MMEXV40 | MMEXCH23 | |
Pulp (%) | 80.58 ± 1.96a† | 80.12 ± 0.99a | 75.47 ± 3.31b |
Peel (%) | 19.41 ± 1.96b | 19.88 ± 0.99b | 24.52 ± 3.29a |
Pulp starch (%) | 8.96 ± 1.14b | 10.59 ± 1.04a | 9.53 ± 0.94ab |
Bagasse starch (%) | 1.91 ± 0.69b | 1.98 ± 0.49b | 2.79 ± 0.78a |
Total starch (%) | 10.87 ± 1.40b | 12.57 ± 1.27a | 12.32 ± 1.02ab |
Fresh bagasse yield (%) | 60.18 ± 2.32b | 57.36 ± 2.51b | 64.39 ± 3.00a |
Dry bagasse yield (%) | 20.19 ± 2.00ab | 19.06 ± 1.66b | 21.96 ± 2.62a |
Note: † Means with the same letter in a row are not significantly different (Tukey, 0.05). |
Notwithstanding both clones having equal percentages of pulp in their storage roots, the MMEXV40 clone stood out for its higher yield in pulp extraction starch (10.59%) and total starch (12.57%), compared against MMEXV5, which had a lower amount, even than MMEXCH23. Thus, the results show that the amount of pulp is not related with starch yield. Similar STL amounts were reached by the improved Mexican cassava varieties Esmeralda and Sabanera, with 10 and 12%, respectively [53], also by varieties from Trinidad and Tobago, Palo Rojo (11.8%), although lower than M Col22 (16.1%) and Maracas (20.3%) [12]. Furthermore, starch yields ranging from 8.4 to 34% have been reported for native and improved clones with high carotenoid content [3,4], which indicates great variation with respect to the yield of starch extraction. This response could be due to the genotype, harvest age, environmental conditions during growing [1,84] or harvest, plant density, growing season, soil type [3], even to the extraction conditions [84].
The greatest presence of the byproduct bagasse in the MMEXCH23 clone (64.39% fresh and 21.96% dry) was related with the high extraction of SBAG (2.79%), this suggests that a high number of starch granules (15.75 to 22.64%) could be caught among the bagasse fiber net [85]. Thus, good byproduct management should be considered during the industrial extraction process in order to minimize losses. Various authors believe that the presence of bagasse depends on factor such as age and the clone [12], even at ratios greater than 30% of dry bagasse [54].
On the other hand, although no significant differences were found for DM, it is reported that the MMEXCH23 clone reached 38% (6.02 t DM ha−1), followed by MMEXV40 (35.91% = 10.66) and MMEXV5 (34.22% = 14.11). Values of 4 to 45% of DM have been reported in the literature [86,87,88], while the values found can be considered high. In this regard, clones with a high dry matter content, a trait closely related to the starch content [24,26,89], are preferred by farmers, breeders, and processors; however, the low fresh storage roots yield of MMEXCH23 may limit its potential [82,88].
The general purpose of the PCA is to summarize the information from a series of response traits into a lower number of dimensions (Principal Components) whose graphs allow us to do an exploratory analysis [90,91,92]. The PCA evidences that with that, the general performance of the traits is explained with a mere two components (Table 2), where PC1 is related with components of storage roots yield and the generation of byproducts, while PC2 explains the yield of starch extraction. The PCA graph (Figure 3) shows the association between morphoagronomic and agro-industrial traits, as well as the differences between the cassava clones.
Feature | PC1 | PC2 |
Plant height | 0.55 | −0.84 |
Leaf lobe length | 0.99 | −0.13 |
Leaf lobe width | 0.83 | 0.56 |
Stem diameter | 0.18 | 0.98 |
Total number of storage roots | 1.00 | −0.06 |
Number of commercial storage roots | 0.77 | −0.63 |
Storage root yield | 0.97 | −0.25 |
Peel | −0.99 | −0.13 |
Pulp | 0.99 | 0.13 |
Pulp starch | −0.05 | 1.00 |
Bagasse starch | −1.00 | −0.06 |
Total starch | −0.41 | 0.91 |
Dry matter | −0.99 | 0.12 |
Fresh bagasse yield | −0.81 | −0.59 |
Dry bagasse yield | −0.82 | −0.58 |
Eigenvalues | 9.97 | 5.03 |
Explained variance (%) | 0.66 | 0.34 |
Accumulated variance (%) | 0.66 | 1.00 |
The particular traits of the MMEXV5 clone were related with high productivity in the field. A high fresh storage roots yield was correlated with a greater number of storage roots, plant height, leaf lobe dimensions, and pulp, similar to the reports by Agre et al. [21], Nadjiam et al. [71], Silva et al. [73], and Temegne et al. [93]. It is important to mention that there was a negative association between NTR and DM; PH with SPUL and STL; as well as RY with STL. Hence, the clones with a higher number of fresh roots and height, developed poor dry matter and yield of starch extraction. Because of this, MMEXV5 can be considered a multipurpose clone given its high productivity of storage roots, since besides its capacity to be grown for human consumption [94] or cattle feed [95,96], which requires a removal process to decrease or eliminate the presence of cyanogenic glucosides, precursors of cyanhydric acid [63,97], its leaves can also be harvested and used as a vegetable or forage (fresh or dehydrated) given their high amount of proteins, vitamins (B1, B2, C, and carotenoids), and minerals (K, P, Mg, and Ca) [7,22], given that their collection does not significantly affect storage roots yield [18].
The MMEXCH23 clone was characterized for its high percentage of solid byproducts (bagasse and peels) and total starch, factors which cause a better proportion of dry matter in the storage root [98]. On the other hand, although bagasse and peels are low-value resources, they can be used as organic fertilizer [99], animal feed [100,101], or cultivation medium for fungi [102,103]. Moreover, they have the potential to be adapted adapt to the biorefinery model, useful to obtain bioenergetic benefits and bioproducts [104], such as the conversion to biofuels of second generation: biobutanol, biodiesel, bioethanol, bio-oil, charcoal [28,105,106,107,108], biogas [31,109]; or bioproducts: cyclodextrins [110], organic acids, lactic acid, α-amylase, biodegradable packaging [111], and even fibers from cellulose [112], nanofibers [32,113], and nanocellulose [114] with potential to be used as reinforcement and improvement of the properties of biodegradable films. These alternatives of use can decrease the volume of bagasse and peels, on the whole, offer added value [115,116].
On the other hand, the traits of MMEXV40 characterize it for its high yield of starch extraction (SPUL and STL), which in turn was associated with STDIA; this morphoagronomic trait can be used as an indicator for the indirect selection of clones with high starch yield. In this regard, the identification of clones with high starch extraction is important for their processing [20], as this helps to decrease production costs, plan the extraction, and provide greater industrial yield [20,25]. Among the potential uses for the MMEXV40 clone stands out thickener for broths, baby food, sauces, cold meats, and processed meats, as well as making cookies, dextrose and glucose syrup as sweeteners in confectionery, monosodium glutamate as a flavor enhancer, adhesives, pills and tablets [5], bioethanol production [117,118,119], and even replace maize as an energy source in animal diets [120,121].
This study successfully determined several morphoagronomic and industrial traits that may be of value in production of byproducts to multiples purposes. The results suggested that the clones MMEXV5, MMEXV40 and MMEXCH23 were highly productive (15.85 to 41.24 t ha−1), with yields higher than the national average (12.58 t ha−1). During the process starch extraction, a greater presence of bagasse in MMEXCH23 was related to a high extraction of bagasse starch (2.79%), which suggested that a high amount of starch granules (15.75 to 22.64%) can be trapped between the fibrous network of bagasse. Based on the PCA, it was found that high productivity of the cassava clones in the field, presented low dry matter and starch extraction; these morphoagronomic traits, must be taken into account in cassava improvement programs. Likewise, this study reveals that the local cassava clones have not been fully exploited in tropics regions for its improvement and further uses.
Finally, the valorization of the evaluated germplasm could make cassava into the basic raw material in a great variety of products with high added value for the food and non-food industry, even obtain bioproducts and bioenergy through the conversion of bagasse and peel.
The National Council of Science and Technology is thanked for the scholarship granted for the development of research work in the area of bioplastics; also, to the National Institute of Forestry, Agricultural and Livestock Research, Cotaxtla Experimental Field for the establishment of cultivation, advice and human resources; and the Universidad Veracruzana, Faculty of Biological and Agricultural Sciences, Orizaba-Córdoba region for the processing and statistical analysis of cassava.
All authors declare no conflicts of interest in this paper.
[1] | Howeler R, Lutaladio N, Thomas G (2013) Save and grow: Cassava: A guide to sustainable production intensification. Rome, 129. |
[2] | Mtunguja MK, Thitisaksakul M, Muzanila YC, et al. (2016) Assessing variation in physicochemical, structural, and functional properties of root starches from novel Tanzanian cassava (Manihot esculenta Crantz.) landraces. Starch‐Stärke 68: 514-527. |
[3] | Buddhakulsomsiri J, Parthanadee P, Pannakkong W (2018) Prediction models of starch content in fresh cassava roots for a tapioca starch manufacturer in Thailand. Comput Electron Agric 154: 296-303. |
[4] | Atwijukire E, Hawumba JF, Baguma Y, et al. (2019) Starch quality traits of improved provitamin A cassava (Manihot esculenta Crantz). Heliyon 5: 1-18. |
[5] | FAO (Food and Agriculture Organization of the United Nations) (2018) Food outlook-biannual report on global food markets-november 2018. Roma, Italia, 100. |
[6] | Lebot V (2016) Tropical roots and tuber crops, cassava, sweet potate, yams and aroids. Cabi, 541. |
[7] | Parmar A, Sturm B, Hensel O (2017) Crops that feed the world: Production and improvement of cassava for food, feed, and industrial uses. Food Secur 9: 907-927. |
[8] | Papong S, Malakul P, Trungkavashirakun R, et al. (2014) Comparative assessment of the environmental profile of PLA and PET drinking water bottles from a life cycle perspective. J Cleaner Prod 65: 539-550. |
[9] | van den Oever M, Molenveld K, Zeevan van der M, et al. (2017) Bio-based and biodegradable plastics: Facts and figures: Focus on food packaging in the Netherlands. Wageningen Food & Biobased Res Report 1722: 1-67. |
[10] | European Bioplastics (2018) Bioplastics market data, 2018. Available from: https://www.european-bioplastics.org/market/. |
[11] | IFBB (Institute for Bioplastics and Biocomposites) (2019) Biopolymers facts and statistics, production capacities, processing routes, feedstock, land and water use. Available from: https://www.ifbb-hannover.de/en/facts-and-statistics.html. |
[12] | Olusola AJ, Adebiyi OB, Riyaad K (2015) Evaluation of new cassava varieties for adhesive properties. Starch/Staerke 67: 561-566. |
[13] | Onyenwoke CA, Simonyan KL (2014) Cassava post-harvest processing and storage in Nigeria: A review. Afr J Agric Res 9: 3853-3863. |
[14] | Veiga JPS, Valle TL, Feltran JC, et al. (2016) Characterization and productivity of cassava waste and its use as an energy source. Renewable Energy 93: 691-699. |
[15] | Byju G, Nedunchezhiyan M, Ravindran CS, et al. (2012) Modeling the response of cassava to fertilizers: A site-specific nutrient management approach for greater tuberous root yield. Commun Soil Sci Plant Anal 43: 1149-1162. |
[16] | Howeler R (2014) Sustainable soil and crop management of cassava in Asia: A reference manual. CIAT Publication No. 389. Cali, Colombia, Centro Internacional de Agricultura Tropical (CIAT), 280. |
[17] | Polthanee A, Srisutham M (2017) Supplementary irrigation for cassava planted in the late rainy season of northeastern Thailand. Asian J Crop Sci 9: 100-108. |
[18] | Munyahali W, Pypers P, Swennen R, et al. (2017) Responses of cassava growth and yield to leaf harvesting frequency and NPK fertilizer in South Kiv, Democratic Republic of Congo. Field Crops Res 214: 194-201. |
[19] | Amamgbo LEF, Akinpelu AO, Omodamiro R, et al. (2016) Promotion and popularization of some elite cassava varieties in IgbariamAnambra state: Implication for food security and empowerment. Global Adv Res J Agric Sci 5: 61-66. |
[20] | Saraiva LS, Ledo CDS, Santos VDS (2019) Effect of harvesting times on agronomic characteristics of industrial cassava genotypes. Revista Brasileira de Ciências Agrárias 14: 1-6. |
[21] | Agre AP, Bhattacharjee R, Dansi A, et al. (2017) Assessment of cassava (Manihot esculenta Crantz) diversity, loss of landraces and farmers preference criteria in southern Benin using farmers' participatory approach. Genet Resour Crop Evol 64: 307-320. |
[22] | Kombate K, Dansi D, Dossou-Aminon I, et al. (2017) Diversity of cassava (Manihot esculenta Crantz) cultivars in the traditional agriculture of Togo. Int J Curr Res Biosci Plant Biol 6: 3328-3240. |
[23] | Vieira E A, Fialho JDF, Carvalho LJCB, et al. (2015) Agronomic performance of sweet cassava germplasm accesses in Cerrados area of Unaí County, northeast region of Minas Gerais. Científica (Jaboticabal) 43: 371-377. |
[24] | Peprah BB, Parkes E, Manu-Aduening J, et al. (2020) Genetic variability, stability and heritability for quality and yield characteristics in provitamin A cassava varieties. Euphytica 216: 1-13. |
[25] | Buddhakulsomsiri J, Parthanadee P, Praneetpholkrang P (2015) Determining appropriate production and inbound logistics practices for a cassava supply chain in Thailand. Nat Sci 49: 937-950. |
[26] | Ceballos H, Hershey CH (2017) Cassava (Manihot esculenta Crantz). In: Genetic Improvement of Tropical Crops, 129-180. |
[27] | Chavalparit O, Ongwandee M (2009) Clean technology for the tapioca starch industry in Thailand. J Cleaner Prod 17: 105-110. |
[28] | Li S, Cui Y, Zhou Y, et al. (2017) The industrial applications of cassava: Current status, opportunities and prospects. J Sci Food Agric 97: 2282-2290. |
[29] | Santos SA, Lopes SY, Araújo KR, et al. (2017) Waste bio-refineries for the cassava starch industry: New trends and review of alternatives. Renewable Sustainable Energy Rev 73: 1265-1275. |
[30] | Tchobanoglous G, Theisen H, Vigil S (1993) Integrated solid waste management: Engineering principles and management issues. McGraw-Hill, New York, 3-22. |
[31] | Trakulvichean S, Chaiprasert P, Otmakhova J, et al. (2017) Integrated economic and environmental assessment of biogas and bioethanol production from cassava cellulosic waste. Waste Biomass Valorization 10: 691-700. |
[32] | Travalini AP, Lamsal B, Magalhães WLE, et al. (2019) Cassava starch films reinforced with lignocellulose nanofibers from cassava bagasse. Int J Biol Macromol 139: 1151-1161. |
[33] | Pothiraj C, Balaji P, Eyini M (2006) Raw starch degrading amylase production by various fungal cultures grown on cassava waste. Mycobiology 34: 128-130. |
[34] | Anyanwu CN, Ibeto CN, Ezeoha SL, et al. (2015) Sustainability of cassava (Manihot esculenta Crantz) as industrial feedstock, energy and food crop in Nigeria. Renewable Energy 81: 745-752. |
[35] | Tran T, Da G, Moreno-Santander MA, et al. (2015) A comparison of energy use, water use and carbon footprint of cassava starch production in Thailand, Vietnam and Colombia. Resour, Conserv Recycl 100: 31-40. |
[36] | Xiong X, Iris KM, Tsang DC, et al. (2019) Value-added chemicals from food supply chain wastes: State-of-the-art review and future prospects. Chem Eng J 375: 24. |
[37] | Pandey A, Soccol CR, Nigam P, et al. (2000) Biotechnological potential of agro-industrial residues. Ⅱ: cassava bagasse. Bioresour Technol 74: 81-87. |
[38] | FAOSTAT (Statistics Division Food and Agriculture Organization of the United Nations) (2019) Data production crops. Roma, Italia. Available from: http://www.fao.org/faostat/en/#data/QC. |
[39] | Góngora-Chin RE, Flores-Guido S, Ruenes-Morales MR, et al. (2016) Uso tradicional de la flora y fauna en los huertos familiares mayas en el municipio de Campeche, Campeche, México. Ecosistemas y Recursos Agropecuarios 3: 379-389. |
[40] | Mateos-Maces L, Castillo-González F, Chávez SJL, et al. (2016) Manejo y aprovechamiento de la agrobiodiversidad en el sistema milpa del sureste de México. Acta Agronómica 65: 413-421. |
[41] | Meléndez GL, Hirose LJ (2018) Patrones culinarios asociados al camote (Ipomoea batatas) y la yuca (Manihot esculenta) entre los mayas yucatecos, ch'oles y huastecos. Estudios de Cultura Maya LII 52: 193-226. |
[42] | Centurión-Hidalgo D, Espinosa-Moreno J, Cruz-Lázaro E, et al. (2019) Estacionalidad de los vegetales comercializados en los mercados públicos del estado de Tabasco del estado de Tabasco. Revista de Alimentación Contemporánea y Desarrollo Regional 29: 16. |
[43] | Ballesteros P, Rodríguez PG, Zavala HLA, et al. (2011) La yuca (Manihot esculenta Crantz), cultivo promisorio para Guerrero. In: Instituto Tecnológico Ciudad Altamirano & Fundación Produce de Guerreo A. C. (Eds.), manual técnico agricultura, 14-25. |
[44] | Meneses MI, Vázquez HA, Rosas GX, et al. (2014) Contenido de materia seca y almidón en clones de yuca (Manihot esculenta Crantz). Revista Biológico Agropecuaria Tuxpan 26: 271-274. |
[45] | SINAREFI (Sistema Nacional de Recursos Fitogenéticos para la Alimentación y la Agricultura) (2013) Manejo, evaluación y acciones que promueven la conservación de yuca (Manihot esculenta Crantz) en México. Resina, Macro Red Impulso, 30. |
[46] | SMN-CONAGUA (Servicio Meteorológico Nacional-Comisión Nacional del Agua) (2020) Información estadística climatológica. Estación climatológica El Tejar, Medellín, Veracruz, México. Datos de temperatura y precipitación. Available from: https://smn.conagua.gob.mx/es/climatologia/informacionclimatologica/informacion-estadistica-climatologica. |
[47] | García E (2004) Modificaciones al sistema de clasificación climática de Köppen. Instituto de Geografía, Universidad Nacional Autónoma de México, 5: 98. |
[48] | NOM-021-RECNAT-2000 (Norma Oficial Mexicana) (2002) NOM-021-RECNAT-2000, que establece las especificaciones de fertilidad, salinidad y clasificación de suelos. Estudios, muestreo y análisis. Diario Oficial. Available from: http://extwprlegs1.fao.org/docs/pdf/mex50674.pdf. |
[49] | Del Rosario-Arellano JL, Meneses-Márquez I, Andrés-Meza P, et al. (2017) Caracterización morfo-agronómica de accesiones de yuca (Manihot esculenta Crantz). In: Perez-Soto F, Figueroa-Hernández E, García-Nuñez RM, et al. Genética y fertilización en la producción agrícola, 60-72. |
[50] | Fukuda WMG, Guevara CL, Kawuki R, et al. (2010) Selected morphological and agronomic descriptors for the characterization of cassava. IITA, Ibadan, Nigeria, 19. |
[51] | Aguiar EB, Valle TL, Lorenzi JO, et al. (2011) Efeito da densidade populacional e época de colheita na produção de raízes de mandioca de mesa. Bragantia 70: 561-569. |
[52] | López OV, Viña SZ, Pachas ANA, et al. (2010) Composition and food properties of Pachyrhizus ahipa roots and starch. Int J Food Sci Technol 45: 223-233. |
[53] | Vargas ENA, Veleva L, Rodríguez CM, et al. (2017) Yuca (Manihot esculenta), alternativa para la producción de bioplásticos. In Martínez SR, González HRI (Ed.). La Ingeniería ambiental y química ante los problemas ambientales en el sureste mexicano, 159-174. |
[54] | Versino F, García MA (2014) Cassava (Manihot esculenta) starch films reinforced with natural fibrous filler. Ind Crops Prod 58: 305-314. |
[55] | Studio R (2018) RStudio: Integrated Development for R. RStudio, Inc., Boston, MA URL. Available from: http://www.rstudio.com/. |
[56] | ECOCROP (Crop Ecological Requirements Database) (2014) Data sheet. Available from: http://ecocrop.fao.org/ecocrop/srv/en/dataSheet?id=1420. |
[57] | El-Sharkawy MA (2006) International research on cassava photosynthesis, productivity, eco-physiology, and responses to environmental stresses in the tropics. Photosynthetica 44: 481-512. |
[58] | Daryanto S, Wang L, Jacinthe PA (2016) Drought effects on root and tuber production: A meta-analysis. Agric Water Manage 176: 122-131. |
[59] | Okogbenin E, Setter TL, Ferguson ME, et al. (2013) Phenotypic approaches to drought in cassava: Review. Front Physiol 4: 1-15. |
[60] | Gregory PJ, Wojciechowski T (2020) Root systems of major tropical root and tuber crops: Root architecture, size, and growth and initiation of storage organs. Adv Agron 161: 1-25. |
[61] | Bellotti A, Campo BVH, Hyman G (2012) Cassava production and pest management: Present and potential threats in a changing environment. Trop Plant Biol 5: 39-72. |
[62] | Parsa S, Medina C, Rodriguez V (2015) Sources of pest resistance in cassava. Crop Prot 68: 79-84. |
[63] | Ceballos H, de la Cruz AGA (2002) Taxonomía y morfología de la yuca. In: Ospina IAB, Ceballos H, (Eds.). La yuca en el tercer milenio. Sistemas modernos de producción procesamiento, utilización y comercialización, 15-32. |
[64] | Hershey C (2017) Achieving sustainable cultivation of cassava. Genetics, breeding, pests and diseases. Burleigh Dodds Science Publishing Limited, 2: 301. |
[65] | Omondi JO, Lazarovitch N, Rachmilevitch S, et al. (2018) Nutrient use efficiency and harvest index of cassava decline as fertilization solution concentration increases. J Plant Nutr Soil Sci 181: 644-654. |
[66] | Byju G, Suja G (2020) Mineral nutrition of cassava. In: Advances in Agronomy (1st ed., Vol. 159). Elsevier Inc. |
[67] | Adiele JG, Schut AGT, van den Beuken RPM, et al. (2020) Towards closing cassava yield gap in west Africa: Agronomic efficiency and storage root yield responses to NPK fertilizers. Field Crops Res 253: 107820. |
[68] | Odubanjo O, Olufayo A, Oguntunde P (2011) Water use, growth, and yield of drip irrigated cassava in a humid tropical environment. Soil Water Res 6: 10-20. |
[69] | Borgues VA, Perreira DR, Morgante CV, et al. (2019) Early prediction models for cassava root yield in different water regimes. Field Crops Res 239: 149-158. |
[70] | Payvandi S, Daly KR, Jones DL, et al. (2014) A mathematical model of water and nutrient transport in xylem vessels of a wheat plant. Bull Math Biol 76: 566-596. |
[71] | Nadjiam D, Sarr PS, Naïtormbaïdé M, et al. (2016) Agro-morphological characterization of cassava (Manihot esculenta Crantz) cultivars from Chad. Agric Sci 7: 14. |
[72] | Oliveira EC, de Almeida LHC, Zucareli C, et al. (2019) Analysis of cassava growth at different harvest times and planting densities. Semina: CiênciasAgrárias 40: 113-126. |
[73] | Silva GR, Freitas de Almeida C, da Silva CJR, et al. (2016) Genetic diversity in sweet cassava from the brazilian middle north region and selection of genotypes based on morpho-agronomical descriptors. Afr J Agric Res 11: 3710-3719. |
[74] | Zayas-Infante S, Boeckx P, Vargas-Rodríguez H (2019) Comportamiento productivo en agroecosistemas de intercalamiento yuca-frijol en el municipio "Calixto García", provincia Holguín. Cultivos Tropicales 40: a03-e03. |
[75] | Manrique LA (1990) Plant morphology of cassava during summer and winter. Agron J 82: 881-886. |
[76] | Salcedo MJ, Chavarria AB, Pérez BJ (2017) Arquitectura del dosel en yuca (Manihot esculenta Crantz) en el bosque seco tropical en Colombia. Revista Colombiana de Ciencia Animal 9: 271-280. |
[77] | Pipatsitee P, Eiumnoh A, Praseartkul P, et al. (2019) Non-destructive leaf area estimation model for overall growth performance in relation to yield attributes of cassava (Manihot esculenta Crantz) under water deficit conditions. Notulae Botanicae Horti Agrobotanici Cluj-Napoca 47: 580-591. |
[78] | Biratu GK, Elias E, Ntawuruhunga P, et al. (2018) Cassava response to the integrated use of manure and NPK fertilizer in Zambia. Heliyon 4: e00759. |
[79] | Dias CA, Silveira VAE, Freire MW, et al. (2014) Avaliação de variedades de mandioca tipo indústria. Magistra, Cruz Das Almas 26: 456-466. |
[80] | Dje BIR, Kouassi KI, Koffi KK, et al. (2018) Evaluation of cassava varieties for weed tolerance ability. Expl Agric 54: 443-451. |
[81] | Kang L, Liang Q, Jiang Q, et al. (2020) Screening of diverse cassava genotypes based on nitrogen uptake efficiency and yield. J Int Agric 19: 965-974. |
[82] | Tumuhimbise R, Melis R, Shanahan P, et al. (2014) Genotype×environment interaction effects on early fresh storage root yield and related traits in cassava. Crop J 2: 329-337. |
[83] | Alarcón MF, Dufour D (1998) Almidón agrio de yuca en Colombia: Tomo I. Producción y recomendaciones. Cali, Colombia: Centro Internacional de Agricultura Tropical. Montpellier Francia: Centro de Cooperación Internacional en recherche agronomique pour le dèveloppement, 35. |
[84] | León-Pacheco R, Pérez-Macías M, Fuenmayor-Campos F, et al. (2018) Root quality of four clones on cassava (Manihot esculenta Crantz) as affected by the irrigation regime. Bioagro 30: 87-91. |
[85] | García MC, Salcedo MJ, Alvis BA (2018) Condiciones óptimas de la etapa de lixiviación en la extracción de almidón de yuca. Biotecnología en el Sector Agropecuario y Agroindustrial 16: 62-67. |
[86] | Sriroth K, Chollakup R, Chotineeranat S, et al. (2000) Processing of cassava waste for improved biomass utilization. Bioresour Technol 71: 63-69. |
[87] | Kawano K, Fukuda WMG, Cenpukdee U (1987) Genetic and environmental effects on dry matter content of cassava root. Crop Sci 27: 69-74. |
[88] | Gonçalves GD, Nogueira PCJ, Silveira VAE, et al. (2017) Physiological and agronomic characteristics of cassava genotypes. Afr J Agric Res 12: 354-361. |
[89] | Kamanda I, Blay ET, Asante IK, et al. (2020) Genetic diversity of provitamin-A cassava (Manihot esculenta Crantz) in Sierra Leone. Genet Resour Crop Evol 67: 1193-1208. |
[90] | Karim KY, Ifie B, Dzidzienyo D, et al. (2020) Genetic characterization of cassava (Manihot esculenta Crantz) genotypes using agro-morphological and single nucleotide polymorphism markers. Physiol Mol Biol Plants 26: 317-330. |
[91] | Afifi AA, Clark V (1996) Computer Aided Multivariate Analysis. Los Angeles, California, EUA, 330-352. |
[92] | Johnson DE (2000) Métodos multivariados aplicados al análisis de datos. International Thomson editors, 556. |
[93] | Castillo MLE (2007) Introducción al SAS para Windows. Universidad Autónoma Chapingo (UACH), Estado de México, México, 295. |
[94] | Temegne NC, Mouafor BI, Ngome AF (2016) Agro-morphological characterization of cassava (Manihot esculenta Crantz) collected in the humid forest and Guinea savannah agro-ecological zones of Cameroon. Greener J Agric Sci 6: 209-225. |
[95] | Tokunaga H, Baba T, Ishitani M, et al. (2018) Sustainable management of invasive cassava pests in Vietnam, Cambodia, and Thailand. In: Kokubun M, Asanuma S (Eds). Crop Production under stressful conditions. Springer, Singapore, 131-157. |
[96] | Adetunji AR, Isadare DA, Akinluwade KJ, et al. (2015) Waste-to-wealth applications of cassava- a review study of industrial and agricultural applications. Adv Res 4: 212-229. |
[97] | Guira F, Some K, Kabore D, et al. (2017) Origins, production, and utilization of cassava in Burkina Faso, a contribution of a neglected crop to household food security. Food Sci Nutr 5: 415-423. |
[98] | Cock JH (2019) Cassava: New potential for a neglected crop. CRC Press, 208. |
[99] | Versino F, López OV, García MA (2019) Exploitation of by-products from cassava and ahipa starch extraction as filler of thermoplastic corn starch. Compos Part B: Eng 182: 107653. |
[100] | Phun-iam M, Anusontpornperm S, Thanachit S, et al. (2018) Yield response of cassava Huay Bong 80 variety grown in an oxyaquicpaleustult to cassava starch waste and nitrogen fertilizer. Agric Natur Resour 52: 573-580. |
[101] | Apata DF, Babalola TO (2012) The use of cassava, sweet potato and cocoyam, and their by-products by non-ruminants. Int J Food Sci Nutr 2: 54-62. |
[102] | Achi CG, Coker AO, Sridhar MKC (2018) Cassava processing wastes: Options and potentials for resource recovery in Nigeria. In: Ghosh S (Eds.), Utilization and management of bioresources. Springer, Singapore, 77-89. |
[103] | de Souza Araújo P, da Silva PGP, de Souza AS, et al. (2020) Changes in biochemical composition of cassava and beet residues during solid state bioprocess with Pleurotus ostreatus. Biocatal Agric Biotechnol 26: 101641. |
[104] | Marin EM, Zajul M, Goldman M. et al. (2020) Effects of solid-state fermentation and the potential use of cassava by-products as fermented food. Waste Biomass Valor 11: 1289-1299. |
[105] | Budzianowski WM (2017) High-value low-volume bioproducts coupled to bioenergies with potential to enhance business development of sustainable biorefineries. Renewable Sustainable Energy Rev 70: 793-804. |
[106] | Elemike EE, Oseghale OC, Okoye AC (2015) Utilization of cellulosic cassava waste for bio-ethanol production. J Environ Chem Eng 3: 2797-2800. |
[107] | Adeleke KM, Itabiyi OE, Ilori OO (2018) Temperature effect on the product yield from pyrolysis of cassava peels. Int J Sci & Eng Res 9: 953. |
[108] | Martínez DG, Feiden A, Bariccatt R, et al. (2018) Ethanol production from waste of cassava processing. Appl Sci 8: 2158. |
[109] | Sivamani S, Chandrasekaran AP, Balajii M, et al. (2018) Evaluation of the potential of cassava-based residues for biofuels production. Rev Environ Sci Biotechnol 17: 553-570. |
[110] | Trakulvichean S, Chaiprasert P, Otmakhova J, et al. (2017) Comparison of fermented animal feed and mushroom growth media as two value-added options for waste cassava pulp management. Waste Manage Res 35: 1210-1219. |
[111] | Pinheiro KH, Watanabe LS, Nixdorf SL, et al. (2018) Cassava bagasse as a substrate to produce cyclodextrins. Starch‐Stärke 70: 30. |
[112] | Polachini TC, Fachin L, Betiol L, et al. (2016) Water adsorption isotherms and thermodynamic properties of cassava bagasse. Thermochim Acta 632: 79-85. |
[113] | Versino F, López OV, Garcia MA (2015) Sustainable use of cassava (Manihot esculenta) roots as raw material for biocomposites development. Ind Crops Prod 65: 79-89. |
[114] | Teixeira EM, Daniel P, Curvelo AAS, et al. (2009) Cassava bagasse cellulosa nanofibrils reinforced plasticized cassava starch. Carbohydr Polym 78: 422-431. |
[115] | Pasquini D, Teixeira EM, Curvelo AAS, et al. (2010) Extraction of cellulose whiskers from cassava bagasse and their applications as reinforcing agent in natural rubber. Ind Crops Prod 32: 486-490. |
[116] | Á lvarez-Castillo A, García-Hernández E, Domínguez-Domínguez MM, et al. (2012) Aprovechamiento integral de los materiales lignocelulósicos. Revista Iberoamericana de polímeros 13: 140-150. |
[117] | Edhirej A, Sapuan SM, Jawaid M, et al. (2017) Cassava: Its polymer, fiber, composite, and application. Polym Compos 38: 555-570. |
[118] | Thatoi H, Dash PK, Mohapatra S, et al. (2016) Bioethanol production from tuber crops using fermentation technology: a review. Int J Sustainable Energy 35: 443-468. |
[119] | Wangpor J, Prayoonyong P, Sakdaronnarong C, et al. (2017) Bioethanol production from cassava starch by enzymatic hydrolysis, fermentation and ex-situ nanofiltration. Energy Procedia 138: 883-888. |
[120] | Candra KP, Murdianto W (2019) Optimization method for the bioethanol production from Giant cassava (Manihot esculenta var. Gajah) originated from east Kalimantan. Indones J Chem 19: 176-182. |
[121] | Apata DF, Babalola TO (2012) The use of cassava, sweet potato and cocoyam, and their by-products by non-ruminants. Int J Food Sci Nut 2: 54-62. |
[122] | Morgan NK, Choct M (2016) Cassava: Nutrient composition and nutritive value in poultry diets. Anim Nut 2: 253-261. |
1. | José Luis Del Rosario-Arellano, Gloria Ivette Bolio-López, Alex Valadez-González, Luis Zamora-Peredo, Noé Aguilar-Rivera, Isaac Meneses-Márquez, Pablo Andrés-Meza, Otto Raúl Leyva-Ovalle, Exploration of cassava clones for the development of biocomposite films, 2021, 9, 2372-0484, 85, 10.3934/matersci.2022006 | |
2. | Pablo Andrés–Meza, Noé Aguilar–Rivera, Isaac Meneses–Márquez, José Luis Del Rosario–Arellano, Gloria Ivette Bolio–López, Otto Raúl Leyva–Ovalle, Cassava cultivation; current and potential use of agroindustrial co–products, 2024, 11, 2372-0352, 248, 10.3934/environsci.2024012 |
Source of variation | Clones | ||
MMEXV5 | MMEXV40 | MMEXCH23 | |
Pulp (%) | 80.58 ± 1.96a† | 80.12 ± 0.99a | 75.47 ± 3.31b |
Peel (%) | 19.41 ± 1.96b | 19.88 ± 0.99b | 24.52 ± 3.29a |
Pulp starch (%) | 8.96 ± 1.14b | 10.59 ± 1.04a | 9.53 ± 0.94ab |
Bagasse starch (%) | 1.91 ± 0.69b | 1.98 ± 0.49b | 2.79 ± 0.78a |
Total starch (%) | 10.87 ± 1.40b | 12.57 ± 1.27a | 12.32 ± 1.02ab |
Fresh bagasse yield (%) | 60.18 ± 2.32b | 57.36 ± 2.51b | 64.39 ± 3.00a |
Dry bagasse yield (%) | 20.19 ± 2.00ab | 19.06 ± 1.66b | 21.96 ± 2.62a |
Note: † Means with the same letter in a row are not significantly different (Tukey, 0.05). |
Feature | PC1 | PC2 |
Plant height | 0.55 | −0.84 |
Leaf lobe length | 0.99 | −0.13 |
Leaf lobe width | 0.83 | 0.56 |
Stem diameter | 0.18 | 0.98 |
Total number of storage roots | 1.00 | −0.06 |
Number of commercial storage roots | 0.77 | −0.63 |
Storage root yield | 0.97 | −0.25 |
Peel | −0.99 | −0.13 |
Pulp | 0.99 | 0.13 |
Pulp starch | −0.05 | 1.00 |
Bagasse starch | −1.00 | −0.06 |
Total starch | −0.41 | 0.91 |
Dry matter | −0.99 | 0.12 |
Fresh bagasse yield | −0.81 | −0.59 |
Dry bagasse yield | −0.82 | −0.58 |
Eigenvalues | 9.97 | 5.03 |
Explained variance (%) | 0.66 | 0.34 |
Accumulated variance (%) | 0.66 | 1.00 |
Source of variation | Clones | ||
MMEXV5 | MMEXV40 | MMEXCH23 | |
Pulp (%) | 80.58 ± 1.96a† | 80.12 ± 0.99a | 75.47 ± 3.31b |
Peel (%) | 19.41 ± 1.96b | 19.88 ± 0.99b | 24.52 ± 3.29a |
Pulp starch (%) | 8.96 ± 1.14b | 10.59 ± 1.04a | 9.53 ± 0.94ab |
Bagasse starch (%) | 1.91 ± 0.69b | 1.98 ± 0.49b | 2.79 ± 0.78a |
Total starch (%) | 10.87 ± 1.40b | 12.57 ± 1.27a | 12.32 ± 1.02ab |
Fresh bagasse yield (%) | 60.18 ± 2.32b | 57.36 ± 2.51b | 64.39 ± 3.00a |
Dry bagasse yield (%) | 20.19 ± 2.00ab | 19.06 ± 1.66b | 21.96 ± 2.62a |
Note: † Means with the same letter in a row are not significantly different (Tukey, 0.05). |
Feature | PC1 | PC2 |
Plant height | 0.55 | −0.84 |
Leaf lobe length | 0.99 | −0.13 |
Leaf lobe width | 0.83 | 0.56 |
Stem diameter | 0.18 | 0.98 |
Total number of storage roots | 1.00 | −0.06 |
Number of commercial storage roots | 0.77 | −0.63 |
Storage root yield | 0.97 | −0.25 |
Peel | −0.99 | −0.13 |
Pulp | 0.99 | 0.13 |
Pulp starch | −0.05 | 1.00 |
Bagasse starch | −1.00 | −0.06 |
Total starch | −0.41 | 0.91 |
Dry matter | −0.99 | 0.12 |
Fresh bagasse yield | −0.81 | −0.59 |
Dry bagasse yield | −0.82 | −0.58 |
Eigenvalues | 9.97 | 5.03 |
Explained variance (%) | 0.66 | 0.34 |
Accumulated variance (%) | 0.66 | 1.00 |