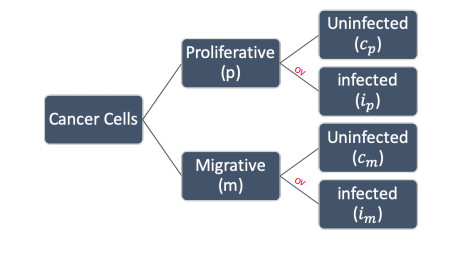
Citation: Eman Koshlaf, Andrew S Ball. Soil bioremediation approaches for petroleum hydrocarbon polluted environments[J]. AIMS Microbiology, 2017, 3(1): 25-49. doi: 10.3934/microbiol.2017.1.25
[1] | Abdulhamed Alsisi, Raluca Eftimie, Dumitru Trucu . Nonlocal multiscale modelling of tumour-oncolytic viruses interactions within a heterogeneous fibrous/non-fibrous extracellular matrix. Mathematical Biosciences and Engineering, 2022, 19(6): 6157-6185. doi: 10.3934/mbe.2022288 |
[2] | Nada Almuallem, Dumitru Trucu, Raluca Eftimie . Oncolytic viral therapies and the delicate balance between virus-macrophage-tumour interactions: A mathematical approach. Mathematical Biosciences and Engineering, 2021, 18(1): 764-799. doi: 10.3934/mbe.2021041 |
[3] | Taeyong Lee, Adrianne L. Jenner, Peter S. Kim, Jeehyun Lee . Application of control theory in a delayed-infection and immune-evading oncolytic virotherapy. Mathematical Biosciences and Engineering, 2020, 17(3): 2361-2383. doi: 10.3934/mbe.2020126 |
[4] | Joseph Malinzi, Rachid Ouifki, Amina Eladdadi, Delfim F. M. Torres, K. A. Jane White . Enhancement of chemotherapy using oncolytic virotherapy: Mathematical and optimal control analysis. Mathematical Biosciences and Engineering, 2018, 15(6): 1435-1463. doi: 10.3934/mbe.2018066 |
[5] | Baba Issa Camara, Houda Mokrani, Evans K. Afenya . Mathematical modeling of glioma therapy using oncolytic viruses. Mathematical Biosciences and Engineering, 2013, 10(3): 565-578. doi: 10.3934/mbe.2013.10.565 |
[6] | Christian Engwer, Markus Knappitsch, Christina Surulescu . A multiscale model for glioma spread including cell-tissue interactions and proliferation. Mathematical Biosciences and Engineering, 2016, 13(2): 443-460. doi: 10.3934/mbe.2015011 |
[7] | Dominik Wodarz . Computational modeling approaches to studying the dynamics of oncolytic viruses. Mathematical Biosciences and Engineering, 2013, 10(3): 939-957. doi: 10.3934/mbe.2013.10.939 |
[8] | Farinaz Forouzannia, Sivabal Sivaloganathan, Mohammad Kohandel . A mathematical study of the impact of cell plasticity on tumour control probability. Mathematical Biosciences and Engineering, 2020, 17(5): 5250-5266. doi: 10.3934/mbe.2020284 |
[9] | Marcello Delitala, Tommaso Lorenzi . Emergence of spatial patterns in a mathematical model for the co-culture dynamics of epithelial-like and mesenchymal-like cells. Mathematical Biosciences and Engineering, 2017, 14(1): 79-93. doi: 10.3934/mbe.2017006 |
[10] | Prathibha Ambegoda, Hsiu-Chuan Wei, Sophia R-J Jang . The role of immune cells in resistance to oncolytic viral therapy. Mathematical Biosciences and Engineering, 2024, 21(5): 5900-5946. doi: 10.3934/mbe.2024261 |
The go or grow (GOG) hypothesis or the migration-proliferation dichotomy, proposes that cell proliferation and cell migration are two temporally exclusive events: cells either migrate or proliferate and they can periodically switch between proliferative and migratory states [1]. Studies on the GOG hypothesis are conflicting, with some studies supporting and confirming this hypothesis in vitro for different cancer cell types (e.g., glioma, melanoma, or breast cancer) [2,3,4,5,6,7,8,9] and other studies challenging this hypothesis [1,10,11]. For example, Tektonidis et al. [9] presented a computational data-driven study of in vitro glioma invasion based on three experimental papers, and concluded that the GOG mechanism combined with self-repulsion and a density-dependent phenotypic switch is mandatory to duplicate the experimental results [7,12]. On the other hand, Corcoran et al. [1] used time-lapse video-microscopy to monitor directional migration, invasion and mitosis of cancer cells, and concluded that in medulloblastoma cell lines there is no evidence to support the GOG hypothesis. More precisely, their results suggested that migrating and non-migrating cell lines have similar mitotic activities. Similarly, Garay et al. [10] tested this hypothesis on 12 mesothelioma, 13 melanoma and 10 lung cancer cell lines using time-lapse video-microscopy, and their results also contradicted the concept of GOG hypothesis.
Over the last decades, mathematical models have been used in addition to experimental studies to shed some light on this go or grow hypothesis. Many of these models are discrete models of cellular automata (CA) type or lattice gas cellular automata (LGCA) type [7,13,14,15,16,17]. There are also various continuum models described by partial differential equations [11,18,19,20,21,22,23,24,25]. These continuum models can either model separately the migrating and proliferative cancer cell sub-populations [20,21,22,24], or can model them via a single equation for one cancer population that can move for some time instances and proliferate for other time instances [11,18]. Those models that consider separate sub-populations of migrating and proliferative cancer cells incorporate also transition (i.e., switching) rates between these two sub-populations. These transition rates can be either constant [20,26] or density dependent: they can depend for example on the total density of cells [20,22], on the concentration of integrins bound to extracellular matrix (ECM) fibres [23], on the density of ECM fibres [25], on the level of oxygen [19,24].
It should be mentioned here that some GOG models have been reduced to simpler forms; for example, in [20] the authors assumed that proliferating cell sub-population could stop proliferating and thus their go-or-growth model was reduced to a go-or-rest model.
The majority of continuum mathematical models in the literature for the GOG hypothesis focus mainly on local interactions between cells. However, it is known that cells can mechanically sense and react to the presence of other cells up to 100μm away [27], and thus more and more mathematical models have been recently developed to consider such non-local cell-cell and cell-ECM interactions [28,29,30,31]. Nevertheless, these nonlocal models do not usually incorporate the GOG hypothesis.
In this study we plan to investigate (for the first time - to our knowledge) the impact of the GOG hypothesis on oncolytic virotherapies. These oncolytic virotherapies are cancer therapies that use oncolytic viruses (OVs), i.e., viruses that replicate inside and destroy cancer cells. Despite some clinical successes with these oncolytic virotherapies (currently a few such viruses are in the late stages of various clinical trials [32]) there are still many open questions related to the interactions between oncolytic viruses and tumour cells [33]. And, to our knowledge, it is not clear at this moment how the spread of oncolytic viruses through the solid tumours is affected by the GOG hypothesis. In this study, we investigate this particular aspect using a modelling and computational approach, which allows us to test numerically various hypotheses related to cancer-OV interactions.
To this end, we extend our previous non-local multi-scale mathematical model for cancer-oncolytic viruses (OV) interactions [34], by considering also the GOG hypothesis. We consider distinct proliferative and migrating cancer cell subpopulations, and assume that they can become infected with an oncolytic virus (see Figure 1). With the help of this new model, we explore numerically different aspects of the GOG hypothesis, as well as the possibility of having local vs. non-local cell interactions and their impact on cancer invasion in the context of oncolytic virotherapies. We need to emphasise here that with the help of this new model we investigate (for the first time - to our knowledge) the impact of the GOG hypothesis on oncolytic virotherapies.
We describe the new multiscale model in Section 2. The computational approach used to simulate numerically this model is described briefly in Section 3. Then, numerical simulations are presented in Section 4. We conclude in Section 5 with a brief summary and discussion of the results.
Adopting the multiscale moving boundary modelling approach introduced initially in [35], in the following we explore the dynamic interaction between an invading heterotypic tumour and an oncolytic virus. Indeed, considering here the go or grow hypothesis [1], the invading tumour is assumed to consist of two subpopulations of cancer cells, namely migrating and proliferative, which exercise their dynamics within the surrounding ECM and that can become infected by an oncolytic virus over a time interval [0,T]. For t∈[0,T], denoting by Ω(t) the spatial support of the progressing tumour that evolves inside a maximal tissue cube Y (i.e., Ω(t)⊂Y), for any x∈Ω(t), let cm(x,t), cp(x,t), and e(x,t) represent the spatial densities of the migrating cancer cells subpopulation, the proliferating cancer cells subpopulation, and the ECM, respectively. Furthermore, denoting here the oncolytic virus density by v(x,t), ∀x∈Ω(t), as both the migrating and the proliferating cancer cells can become infected by the oncolytic virus v(x,t), let im(x,t) and ip(x,t) represent the densities of infected migrating cancer cells and proliferating cancer cells, respectively (see Figure 1).
By incorporating here the GOG hypothesis, we expand and generalise the modelling framework for tumour-OV interaction proposed in [29,34]. Indeed, building on the multiscale framework introduced in [35], we explore these complex cancer-OV interactions by accounting for the interlinked two-scale dynamics that connects the tissue-scale (macro-scale) tumour macro-dynamics with the cell-scale (micro-scale) proteolytic activity of MDEs that occurs along the invasive edge of the tumour. In the following we describe in detail the macro-dynamics, the micro-dynamics, as well as the double feed-back loop that connects the two scales of activity in our new model.
For each t∈[0,t], and each x∈Ω(t), denoting the total cancer cell population by ctotal(x,t)
ctotal(x,t)=cp(x,t)+ip(x,t)+cm(x,t)+im(x,t), | (2.1) |
and defining the total tumour vector to be u(x,t)=(cp(x,t),ip(x,t),cm(x,t),im(x,t),e(x,t))T, the volume fraction of space occupied by the tumour can therefore be expressed mathematically as
ρ(u)=νee(x,t)+νc(ctotal(x,t)), | (2.2) |
where νe represents the fraction of physical space occupied by the ECM and νc is the fraction of physical space occupied collectively by all cancer subpopulations.
For the tumour dynamics, we assume that the motility of each of the cancer cells subpopulations is due to a combination of random movement (described by linear diffusion term) and a directed migration due to cell-cell and cell-ECM adhesion. The spatial fluxes triggered by cell adhesion that cause the directed cells migration are considered here both from a local and non-local perspective [36,37,38,39,40,41], and in following we will detail their mathematical formulation. For convenience, for each cancer cell subpopulation c∈{cp,cm,ip,im}, we consider a global notation φc(u) describing the effect of the cell adhesion processes either locally, through adhesive interactions between cancer cells and ECM (whereby the tumour cells exercise haptotactic movement [42] towards higher levels of ECM), or non-locally, where both cell-cell and cell-ECM adhesive interactions are accounted for within an appropriate cell sensing region. Thus, φc(u) is mathematically formalised as
φc(u):={ηc∇⋅(c∇e),local {haptotactic interactions between cancer cells and ECM},∇⋅(cAc(⋅,⋅,u(⋅,⋅))),non-local {cell - cells and cell - ECM interactions on a cell sensing region}, | (2.3) |
where, for any given subpopulation c∈{cp,cm,ip,im}, we have that ηc>0 is a constant haptotactic rate associated to c, while Ac(x,t,u(⋅,t)) is a non-local spatial flux term that is detailed as follows. Indeed, following a similar approach as in [28,43,44], ∀c∈{cp,cm,ip,im}, at each spatio-temporal point (x,t) the cell adhesion flux Ac(x,t,u(⋅,t)) cumulates the strengths of the cell-cell and cell-matrix adhesion junctions that cells from cancer subpopulation c that distributed at (x,t) establish with the other cell subpopulations and the ECM distributed within an appropriate maximal sensing region B(x,R) of radius R>0. This is formulated mathematically as
Ac(x,t,u(⋅,t))=1R∫B(0,R)n(y)K(‖y‖2)[Sccc(x+y,t)dy+>Scee(x+y,t)](1−ρ(u))+χΩ(t)(x+y,t)dy. | (2.4) |
here, χΩ(t)(⋅) is the characteristic function of Ω(t), while the term (1−ρ(u))+:=max{(1−ρ(u)),0} enables the avoidance of local overcrowding. Further, for any y∈B(0,R), n(y) denotes the unit radial vector originating from x and pointing to x+y∈B(x,R), which is given by
n(y):={y‖y‖2ify∈B(0,R)∖{(0,0)},(0,0)otherwise. | (2.5) |
with ∥⋅∥2 being the usual Euclidean norm. Moreover, K(⋅):[0,R]→[0,1] is a radially symmetric kernel that explores the dependance of the strengths of the established cell adhesion junctions on the radial distance from the centre of the sensing region x to ζ∈B(x,R). Since these adhesion junction strengths are assumed to decrease as the distance r:=∥x−ζ∥2 increases, K therefore is taken here of the form
K(r):=32πR2(1−r2R),∀r∈[0,R]. | (2.6) |
Furthermore, since in this study we focus only on self-adhesion and we do not consider cross-adhesion bonds between four cancer cells subpopulation, in Eq (2.4) we have that Scc and Sce for any given cancer cell subpopulation c∈{cp,cm,ip,im} represent the adhesive interaction strengths for the self−cell−cell adhesion and cell-ECM, respectively. While the cell-ECM adhesion strength Sce is considered to be a positive constant, the cell-cell adhesion strength Scc explores here the fact that the ability of the cell distributed at x to establish cell-cell adhesive junctions with the cells distributed at the other locations y∈B(x,R) depends on the amount of intercellular Ca2+ ions available within the ECM [45,46]. As a consequence, adopting a similar approach to the one in [47], we assume here that Scc is dependent on the ECM density and it takes the form
Scc(e)=Smaxccexp(1−11−(1−e(x,t))2), | (2.7) |
with Smaxcc representing the maximum strength of cell-cell adhesive junctions established by the cancer cells subpopulation c∈{cp,cm,ip,im} . Therefore, the adhesive strengths for cell-cell and cell-ECM adhesion for all four cancer subpopulation can be compactly expressed via the diagonal matrices
Scell−cell=[Scpcp0000Sipip0000Scmcm0000Simim]andScell−ECM=[Scpe0000Sipe0000Scme0000Sime], | (2.8) |
respectively.
Finally, in the context of the GOG hypothesis, another important aspect that occurs during the tumour dynamics is the transitions between from the proliferative cancer subpopulation and the migrating one. Adopting a similar form to the one proposed in [15,16,26], the transition from proliferative to migrating cancer cells is captured here through the switching term λcp,cm that is given by
λcp,cm=ω2cm−ω1cp, | (2.9) |
where ω1 is the rate of switching from proliferative state cp to migration state cm, and ω2 is the rate of switching from migration to proliferative state. On the other hand, the transition from migrating to proliferative cancer cells is expressed through the switching term λcm,cp defined as
λcm,cp=−λcp,cm. | (2.10) |
Thus, for each of the cancer cells subpopulation c∈{cp,cm,ip,im}, the spatial transport is a combination of random movement (expressed through diffusion) and directed movement due adhesion (explored either locally or non-locally, and represented compactly through φc(u)). Furthermore, for the particular case of the migrating and proliferative cells subpopulation cp, and cm, besides the spatial transport and in addition to their own proliferation (considered here of logistic type [48,49]), their dynamics is also affected by the cell population "exchanges" due to proliferative-migrating transitions (i.e., transitions between proliferative and migrating subpopulations) as well as by the presence of the oncolytic virus that is able to infect cells from both populations. Finally, for their part, the infected cancer cells subpopulations, while exercising a spatial transport of the type described above, they contribute to virus replication and die. Therefore, the dynamics for each cancer cell population can be expressed mathematically as follows.
First, the governing equation for the uninfected proliferative cancer cell subpopulation is given by
∂cp∂t=DcpΔcp−φcp(u)+μpcp(1−ρ(u))−ϱpcpv+λcp,cm, | (2.11) |
where Dcp>0 is a constant diffusion coefficient, the term φcp(u) represents the directed movement triggered by cell-adhesion processes that corresponds to cp and is described in Eq (2.3) for c=cp. Further, μp>0 is an intrinsic constant proliferation rate, ϱp>0 is the rate at which the oncolytic virus infects the proliferative cancer cell population, and λcp,cm is the switching term given in (2.9), representing the process through which migrating cancer cells transition towards proliferative state during the tumour dynamics.
The infected proliferative cancer cell population, ip(t,x), which emerges within this dynamics due to the OV infection of cp, also exercises a spatio-temporal dynamics that is governed by the following equation
∂ip∂t=DipΔip−φip(u)+ϱpcpv−δipip, | (2.12) |
where Dip>0 is a constant random motility coefficient, and φip(u) is the spatial influence of the cell-adhesion processes that is described in Eq (2.3) and corresponds to ip. The cancer cell population increases with at rate ϱp due to the new infections of the proliferative cancer cells, and decreases at rate δip>0 due to infected cell death.
Further, since for the migrating cancer cell population we always take into account not only cell-ECM adhesion but also cell-cell self-adhesion, the directed cell migration term φcm(u) that is defined in Eq (2.3) and corresponds to cm is in this case constantly of the non-local form
φcm(u)=∇⋅(cmAcm(⋅,⋅,u(⋅,⋅))), |
where the spatial flux Acm(⋅,⋅,u(⋅,⋅)) is the one defined in Eq (2.4) for c=cm. As a consequence, the governing equation for the uninfected migrating cell population is
∂cm∂t=DcmΔcm−∇⋅(cmAcm)+μmcm(1−ρ(u))−ϱmcmv+λcm,cp, | (2.13) |
where Dcm>0 is a constant diffusion coefficient, μm>0 is a constant proliferation coefficient, ϱm>0 is a constant rate at which the uninfected migrating population diminishes due infection by the oncolytic virus v. Further, λcm,cp is the switching term given in Eq (2.10) that represents the net transition from the proliferative into the uninfected migrating state that occurs per unit time during the tumour dynamics.
The fourth tumour cell population is the infected migrating cancer cell subpopulation im(t,x) that emerges within this dynamics due to infections by the OV, and its spatio-temporal dynamics is governed by the following equation
∂im∂t=DimΔim−φip(u)+ϱmcmv−δimim. | (2.14) |
where Dim>0 is a constant random motility coefficient, and φim(u) represents the directed migration induced by the cell-adhesion processes that corresponds to im and is described in Eq (2.3) for c=im Further, the infected migrating population expand at a rate ϱm due to new infections occurring among the uninfected migrating cells, and they also die at rate δim>0.
At the same time, the ECM is degraded by both uninfected and infected cancer cell populations and is remodelled within the limit of available space. Thus, its governing dynamics is given mathematically by
∂e∂t=−e(αcpcp+αipip+αcmcm+αimim)+μ2e(1−ρ(u)), | (2.15) |
where αcp>0, αip>0, αcm>0, and αim>0 are the ECM degradation rates caused by cancer cells subpopulation cp, ip, cm, and im, respectively. Further, μ2>0 is a constant ECM remodelling rate.
Concerning the oncolytic virus spatio-temporal dynamics, we adopt here a similar reasoning as in [34], and we assume that the OV motion is described by a random movement that is biased by a "haptotactic-like" spatial transport towards higher ECM levels. Thus, the dynamics of the oncolytic virus that we consider here is governed by
∂v∂t=DvΔv−ηv∇⋅(v∇e)+bmim+bpip−(ϱmcm+ϱpcp)v−δvv, | (2.16) |
where Dv>0 is a constant random motility coefficient, ηv>0 is a constant haptotactic coefficient, bm,bp>0 are a viral replication rates within infected proliferating and infected migrating cancer cells, respectively, and δv>0 is the viral decay rate.
Finally, the coupled interacting tumour − OV macro-dynamics is governed by Eqs (2.11)-(2.16) in the presence of initial conditions
cp(x,0)=c0p(x),ip(x,0)=i0p(x),cm(x,0)=c0m(x),andim(x,0)=i0m(x),∀x∈Ω(0), | (2.17) |
while assuming zero-flux boundary conditions at the moving tumour interface ∂Ω(t).
During their macro-scale dynamics, the four cancer cells subpopulations that get near the tumour interface (i.e., within the outer proliferating rim of the tumour) are able to secrete matrix degrading enzymes (such as the matrix metalloproteinases [50,51]), providing this way a source of MDEs for a cell-scale (micro-scale) proteolytic micro-dynamics that takes place along the invasive edge of the tumour. Indeed, in the presence of this source of MDEs (induced by the tumour macro-dynamics), a cross-interface micro-scale MDEs spatial transport occurs within a micro-scale neighbourhood of the tumour boundary of an appropriate cell-scale thickness ϵ>0, denoted here simply by Nϵ(∂Ω(t)). The areas of significant ECM degradation caused by the pattern of propagation of the advancing front of MDEs within the peritumoural region Nϵ(∂Ω(t))∖Ω(t) will ultimately be explored by the cancer cells that will progress in those regions [51], and so precisely these boundary regions (affected by significant ECM degradation) will shape the pattern of tumour progression. Thus, following the modelling approach introduced in [35] we depict these regions of significant ECM degradations by exploring the MDEs micro-dynamic processes within Nϵ(∂Ω(t)), which enables us ultimately to determine the law of the macro-scale tumour boundary movement.
To formalise these laws of macro-scale boundary movement induced by the boundary MDEs micro-dynamics, we adopt here the approach introduced in [35]. Therefore, the micro-scale neighbourhood Nϵ(∂Ω(t)) is given here as a union of a covering bundle of ϵ−size overlapping micro-domains {ϵY}ϵY∈Pϵ(t), namely,
Nϵ(∂Ω(t)):=⋃ϵY∈P(t)ϵY. |
This enables us to decompose the MDEs micro-dynamics on Nϵ(∂Ω(t)) by exploring this as a union of micro-dynamic processes occurring on each ϵY∈Pϵ(t). At any instance in time t0>0, on each micro-domain ϵY∈Pϵ(t0), a source of MDEs appears at every micro-scale location z∈ϵY∩Ω(t0) as a collective contribution of all the cells (both infected and uninfected) from the tumour outer proliferating rim that arrive during their dynamics within a distance ρ>0 from z. Thus, over any small time interval of length Δt>0, [t0,t0+Δt] and at any micro-scale spatial location z∈ϵY, this MDEs source is therefore given as
fϵY(z,τ)={∫B(z,ρ)∩Ω(t0)(γcpcp+γipip+γcmcm+γimim)(x,t0+τ)dxλ(B(z,ρ)∩Ω(t0)),z∈ϵY∩Ω(t0),0,otherwise, | (2.18) |
where λ(⋅) is the standard Lebesgue measure on RN, the ball B(z,r):={x∈Y:‖z−x‖∞≤ρ} is the maximal outer proliferating rim region from where cells that get to contribute to the formation of MDEs source at (z,τ)∈ϵY×[t0,t0+Δt], and γcp, γip, γcm, γim are all positive constants representing the contributions of the cancer subpopulations of uninfected proliferative cells, infected cancer cells, uninfected migrating cells, and infected migrating cells, respectively.
In the presence of the micro-scale source of MDEs induced from the macro-dynamics on each micro-domain ϵY, these matrix degrading enzymes exhibits a diffusion transport process within the entire ϵY. Thus, denoting the MDEs distribution at (z,τ)∈ϵY×[0,Δt] by m(z,τ), the MDEs micro-dynamics on each ϵY is given by
∂m∂τ=DmΔm+fϵY(z,τ) | (2.19) |
where z∈ϵY, τ∈[0,Δt]. Furthermore, since we assume no pre-existing MDEs within ϵY prior to the initiation of the proteolytic micro-dynamics, the MDEs micro-dynamics Eq (2.25g) takes place in the presence of zero initial conditions. Furthermore, we assume the presence of zero-flux boundary condition, namely
m(z,0)=0,n⋅∇m∣∂Ω=0, | (2.20) |
where nϵY is the outward unit normal on the frontier of the micro-domain ∂ϵY.
During the micro-dynamics Eqs (2.19) and (2.20), the MDEs transported across the interface in the peritumoural region ϵY∖Ω(t0) interact with ECM distribution that they encounter, resulting in degradation of ECM constituents. The advancement of MDEs within the peritumoural region ϵY∖Ω(t) lead to a degradation of the ECM in that cell-scale region, and determines the way the macroscopic tumour boundary evolves, leading to the establishment of a boundary movement law. Indeed, following the derivation in [35], the MDEs micro-dynamics on each micro-domain ϵY enables us to derive the movement characteristics for the relocation of the macro-scale tumour boundary ∂Ω(t)∩ϵY, expressing these through the derivation of a direction of movement ηϵY and a displacement magnitude ξϵY in that direction for the advancement of ∂Ω(t)∩ϵY within the peritumoural region Nϵ(∂Ω(t))∖Ω(t). To simplify the representation, the choreographic movement exercised by the ∂Ω(t)∩ϵY over a given time span [t0,t0+Δt] is represented back at macro-scale through the movement of the associated boundary midpoint x∗ϵY of ϵY (defined topologically with full details in [35], and which can be regarded as "the center of ∂Ω(t)∩ϵY"), as illustrated in Figure 2. For completeness, we briefly outline below the main steps involved in deriving the boundary relocation characteristics that were introduced in [35].
On the cell-scale neighbouring bundle Nϵ(∂Ω(t0)) of the tumour interface, for each of the boundary micro-domains ϵY∈P(t0) at a given a time instance t0>0, we use the regularity property of Lebesgue measure [52] to depict the first dyadic decomposition {Dk}k∈IϵY of ϵY that has the property that the union of those dyadic cubes Dk included in the complement of Ω(t0) approximate to a given global micro-scale accuracy δΩ(⋅)>0. This is schematically illustrated by the small green squares in Figure 2 that are situated outside the black tumour boundary ∂Ω(t0)∩ϵY. Further, denoting by yk the barycenter of Dk, we sub-select a sub-family of dyadic cubes {Dk}k∈I∗ϵY⊂{Dk}k∈IϵY that consists only of those dyadic cubes that are situated furthest away from the boundary midpoint x∗ϵY (corresponding to ϵY) with the property that they carry an amount of MDEs above the mean of MDEs transported within the entire preritumoural region ϵY∖Ω(t0), hence covering precisely the region of significant ECM degradation caused by MDEs within ϵY∖Ω(t0), as illustrated in Figure 2. Thus, by cumulating the contribution to the significant ECM degradation within ϵY∖Ω(t0) of all the dyadic cubes {Dk}k∈I∗ϵY while accounting on both their relative spatial location with respect to x∗ϵY and the amount of MDEs that they get to carry at time τf:=t0+Δt, we obtain the direction of choreographic boundary relocation (exercised by the ∂Ω(t)∩ϵY) due to micro-scale MDEs degradation. Therefore, this boundary movement direction is given by the positive direction of the emerging line defined by the position vectors involved {→ykx∗ϵY}k∈I∗ϵY magnified accordingly by the MDEs mass that each dyadic cube in {Dk}k∈I∗ϵY, carries, namely
ηϵY=x∗ϵY+ν∑k∈I∗ϵY(∫Dkm(z,τf)dz)(yk−x∗ϵY),ν∈[0,∞). | (2.21) |
Furthermore, the magnitude of the actual boundary movement in direction ηϵY is appropriately given as a weighted sum of the Euclidean magnitudes of the position vectors {∥ykx∗ϵY∥2}k∈I∗ϵY, with the weights accounting on the relative contribution brought to the ECM degradation of each of the corresponding dyadic cubes. Thus the movement magnitude in direction ηϵY is given by
ξϵY(x):=∑k∈I∗ϵY∫Dkm(z,τf)dz∑k∈I∗ϵY∫Dkm(z,τf)dz∥→xyk∥2. | (2.22) |
Therefore, as the tumour boundary relocation induced by the micro-dynamics on each ϵY is represented at macro-scale through the movement of the boundary midpoint x∗ϵY, in the context that enough but not complete ECM degradation occurs within ϵY∖Ω(t0) (tissue condition that is detailed and explored in full in [35]), we have that x∗ϵY exercises a relocation to a new position ~x∗ϵY that is given by
~x∗ϵY=x∗ϵY+ξϵY(x)~ηϵY∥~ηϵY∥2, | (2.23) |
where
~ηϵY:=∑k∈I∗ϵY(∫Dkm(z,τf)dz)(yk−x∗ϵY), | (2.24) |
and is illustrated through the dark blue arrow in Figure 2. Thus, a law for macro-scale tumour boundary movement is this way induced by the MDEs micro-dynamics, enabling us to capture the evolution of the tumour boundary over the time interval [t0,t0+Δt] from its state Ω(t0) at t0 to a new spatial configuration at Ω(t0+Δt) at t0+Δt. This relocated domain Ω(t0+Δt) allows the initiation of the dynamics on the next time interval [t0,t0+Δt] where the tumour-oncolytic virus interaction continues its proceedings.
In summary, the multiscale moving boundary model that we obtained for the tumour−OV interaction (schematically illustrated in Figure 2) is structured as follows,
the tumour-OVmacro-dynamics:∂cp∂t=DcpΔcp−φcp(u)+μpcp(1−ρ(u))−ϱpcpv+λcp,cm, | (2.25a) |
∂ip∂t=DipΔip−φip(u)+ϱpcpv−δipip, | (2.25b) |
∂cm∂t=DcmΔcm−∇⋅(cmAcm)+μmcm(1−ρ(u))−ϱmcmv+λcm,cp, | (2.25c) |
∂im∂t=DimΔim−φip(u)+ϱmcmv−δimim, | (2.25d) |
∂e∂t=−e(αcpcp+αipip+αcmcm+αimim)+μ2e(1−ρ(u)), | (2.25e) |
∂v∂t=DvΔv−ηv∇⋅(v∇e)+bmim+bpip−(ϱmcm+ϱpcp)v−δvv, | (2.25f) |
boundary MDEsmicro-dynamics:∂m∂τ=DmΔm+fϵY(z,τ) | (2.25g) |
The macro-dynamics and micro-dynamics are connected through a double feedback loop enabled by:
● a top-down link by which the macro-dynamics induces the source for the micro-dynamics given in Eq (2.18).
● a bottom-up link by which the MDEs micro-dynamics induces and determines the law for the macro-scale tumour boundary movement.
The numerical approach and computational implementation of the novel multiscale moving boundary model require a number of steps that build on the multiscale moving boundary computational framework initially introduced by [35] and further expanded in [34,47].
Macro-scale computations. The maximal macro-scale tissue domain Y⊂R2, where the tumour-OV interacting macro-dynamics Eq 2.25(a)-(f) takes place, is considered here to be Y:=[0,4]×[0,4] and is discretised uniformly using a spatial step size Δx=Δy:=h, with h>0. Let's denote by Yd the discretised Y, i.e., Yd:={(x1i,x2j)}i,j=1…N, with N=[4/h]+1. Further, as the macro-dynamics Eq 2.25(a)-(f) is addressed only on the expanding tumour domain Ω(t)⊂Y, for convenience, for any t>0, we denote the discretised tumour domain by Ωd(t) (i.e., Ωd(t)=Yd∩Ω(t)) and the discretised tumour boundary by ∂Ωd(t) (i.e., the frontier of Ωd(t) is ∂Ωd(t)). To carry out the computations exclusively on the expanding tumour, the numerical scheme that we developed here involves a method of lines-type approach combined with a non-local predictor corrector time-marching method introduced in [47] (and, for completeness, summarised also in Appendices A and B). Finally, as the tumour progresses, Ωd(t) is appropriately expanded by activating and including within tumour domain the new points invaded by cancer within Yd.
Approximating the micro-dynamics and its top-down and bottom-up links with the tumour−OV macro-dynamics. At any instance of time t0, we consider that the cell-scale covering bundle {ϵY}ϵY∈Pϵ(t0) of the discretised tumour interface ∂Ωd(t0) consists of overlapping squares ϵY of micro-scale size ϵ:=2h, which are centred at each of the tumour interface spatial node (x1s,x2p)∈∂Ωd(t0), i.e.,
{ϵY}ϵY∈Pϵ(t0)={B∥⋅∥∞((x1s,x2p),ϵ/2)|(x1s,x2p)∈∂Ωd(t0)} |
where ∥⋅∥∞ is the usual ∞−norm, and B∥⋅∥∞((x1s,x2p),ϵ/2):={(z1,z2)∈R2|∥(x1s,x2p)−(z1,z2)∥∞≤ϵ/2} is the closed ball of radius ϵ/2.} By adopting a similar approach to the one introduced in [35], we use using bilinear shape functions to calculate the MDE source given by Eq (2.18) on each micro-domain ϵY. To solve MDEs micro-dynamics Eq (2.25g), we use backward Euler in time combined with central differences for the spatial discretisation. After finding the MDE distribution m(z,τ), with (z,τ)∈ϵY×[0,Δt], we follow the modelling and computational approach introduced in [35] to determine the direction ηϵY and displacement magnitude ξϵY for the movement of the tumour boundary ∂Ω(t0)∩ϵY that is captured by each micro-domain ϵY:=B∥⋅∥∞ ((x1s,x2p),ϵ) and is represented trough the movement of its midpoint (x1s,x2p)∈∂Ωd(t0). Finally, we use these movement characteristics induced from the micro-dynamics (i.e., ηϵY and ξϵY, ∀ϵY∈Pϵ(t0)) to proceed with the corresponding global relocation of the macro-scale tumour boundary ∂Ωd(t0) to its new spatial configuration ∂Ωd(t0+Δt), which emerges due to the multiscale tumour evolution over the time interval [t0,t0+Δt].
In our numerical experiments, we explore the multiscale model dynamics on three distinct local and non-local scenarios that we consider within the macro-dynamics Eq 2.25(a)-(f) for the directed migration due to cell adhesion for cancer cell subpopulations cp, ip, and im. Specifically, we consider the following cases:
1. The cell-adhesion interactions for both the uninfected proliferative subpopulation cp and for the infected subpopulations ip, and im are considered to be local of haptotactic type, i.e., in the coupled macro-dynamics in Eq 2.25(a)-(f) we have φcp(u)=ηcp∇⋅(cp∇e), φip(u)=ηip∇⋅(ip∇e), and φim(u)=ηim∇⋅(im∇e). Thus, the macro-dynamics Eq 2.25(a)-(f) is in this case of the form:
∂cp∂t=DcpΔcp−ηcp∇⋅(cp∇e)+μpcp(1−ρ(u))−ϱpcpv+λcp,cm, | (3.1a) |
∂ip∂t=DipΔip−ηip∇⋅(ip∇e)+ϱpcpv−δipip, | (3.1b) |
∂cm∂t=DcmΔcm−∇⋅(cmAcm)+μmcm(1−ρ(u))−ϱmcmv+λcm,cp, | (3.1c) |
∂im∂t=DimΔim−ηim∇⋅(im∇e)+ϱmcmv−δimim, | (3.1d) |
∂e∂t=−e(αcpcp+αipip+αcmcm+αimim)+μ2e(1−ρ(u)), | (3.1e) |
∂v∂t=DvΔv−ηv∇⋅(v∇e)+bmim+bpip−(ϱmcm+ϱpcp)v−δvv, | (3.1f) |
with results for this case shown in Figure 4.
2. The cell-adhesion interactions for the uninfected proliferative subpopulation cp are considered now to be non-local, while the infected subpopulations ip, and im are still considered to be local of haptotactic type. Hence, in the coupled macro-dynamics in Eq 2.25(a)-(f) we have φcp(u)=∇⋅(cpAcp(⋅,⋅,u(⋅,⋅))), while φip(u)=ηip∇⋅(ip∇e), and φim(u)=ηim∇⋅(im∇e). Thus, the macro-dynamics Eq 2.25(a)-(f) is in this case of the form:
∂cp∂t=DcpΔcp−∇⋅(cpAcp(⋅,⋅,u(⋅,⋅)))+μpcp(1−ρ(u))−ϱpcpv+λcp,cm, | (3.2a) |
∂ip∂t=DipΔip−ηip∇⋅(ip∇e)+ϱpcpv−δipip, | (3.2b) |
∂cm∂t=DcmΔcm−∇⋅(cmAcm)+μmcm(1−ρ(u))−ϱmcmv+λcm,cp, | (3.2c) |
∂im∂t=DimΔim−ηim∇⋅(im∇e)+ϱmcmv−δimim, | (3.2d) |
∂e∂t=−e(αcpcp+αipip+αcmcm+αimim)+μ2e(1−ρ(u)), | (3.2e) |
∂v∂t=DvΔv−ηv∇⋅(v∇e)+bmim+bpip−(ϱmcm+ϱpcp)v−δvv, | (3.2f) |
with results for this case shown in Figure 5(a).
3. Finally, all the cell-adhesion interactions for both the uninfected proliferative subpopulation cp and for the infected subpopulations ip, and im are considered to be non-local, i.e., in the coupled macro-dynamics in Eq 2.25(a)-(f) we have φcp(u)=∇⋅(cpAcp(⋅,⋅,u(⋅,⋅))), φip(u)=∇⋅(ipAip(⋅,⋅,u(⋅,⋅))), and φim(u)=∇⋅(imAim(⋅,⋅,u(⋅,⋅))). Thus, the macro-dynamics Eq 2.25(a)-(f) is in this case of the form:
∂cp∂t=DcpΔcp−∇⋅(cpAcp)+μpcp(1−ρ(u))−ϱpcpv+λcp,cm, | (3.3a) |
∂ip∂t=DipΔip−∇⋅(ipAip)+ϱpcpv−δipip, | (3.3b) |
∂cm∂t=DcmΔcm−∇⋅(cmAcm)+μmcm(1−ρ(u))−ϱmcmv+λcm,cp, | (3.3c) |
∂im∂t=DimΔim−∇⋅(imAim)+ϱmcmv−δimim, | (3.3d) |
∂e∂t=−e(αcpcp+αipip+αcmcm+αimim)+μ2e(1−ρ(u)), | (3.3e) |
∂v∂t=DvΔv−ηv∇⋅(v∇e)+bmim+bpip−(ϱmcm+ϱpcp)v−δvv, | (3.3f) |
with results for this case shown in Figure 5(b).
The initial conditions for the uninfected proliferative cancer cell population, cp(x,0) is chosen to describe a small localised pre-existing tumour aggregation. This is given by the following equations:
c0p(x)=0.5(exp(−‖x−(2,2)‖222h)−exp(−3.0625))(χB((2,2),0.5−γ)∗ψγ),∀x∈Y, | (3.4) |
whose plot is shown in Figure 3(a). Here ψγ:RN→R+ is the usual standard mollifier of radius γ<<Δx3 given by
ψγ(x):=1γNψ(xγ), | (3.5) |
where ψ is the smooth compact support function given by
ψ(x):={exp1‖x‖22−1if‖x‖2<1,0otherwise. | (3.6) |
Moreover, we assume that the tumour is detected early enough so that migration is not initiated at the start of these simulations, and thus
c0m(x)=0,∀x∈Y. | (3.7) |
Also, since there is no infection at this stage, we assume that both infected proliferative (ip(x,0)) and migrating (im(x,0)) cancer cells are zero:
i0p(x)=0,andi0m(x)=0,∀x∈Y. | (3.8) |
Furthermore, the initial condition for the ECM density, e(x,0), is represented by an arbitrarily chosen heterogeneous pattern described by the following equations (as in [47])
e(x,0)=12min{h(ζ1(x),ζ2(x)),1−c0p(x)}, | (3.9) |
and is shown in Figure 3(b). Here, we have
h(ζ1(x),ζ2(x)):=12+14sin(ξζ1(x)ζ2(x))3⋅sin(ξζ2(x)ζ1(x)),(ζ1(x),ζ2(x)):=13(x+32)∈[0,1]2,∀x∈Y,andξ=7π. | (3.10) |
While other types of heterogeneous ECM patterns could be considered (see [53]), here we focus our attention to explore cancer-viral dynamics on this particular ECM pattern.
Finally, the initial conditions for the OV population, v(x,0) is chosen to describe one single injection in the middle of the tumour aggregation, as in [34]. This is described by the equation
v0(x)=Φ(x)⋅θ(v), | (3.11) |
where
Φ(x)=18(exp(−‖x−(2,2)‖222h)−exp(−1.6625)),andθ(v)={1ifΦ(x)>5×10−5,0otherwise. | (3.12) |
In computations, the initial condition is smoothed out on the frontier of the viral density support Γv:=∂{x∈Y|v0(x)>0} via the averaging
v(x1,x2)=18(−v(x1,x2)+∑i,j∈{−1,0,1}v(x1+ih,x2+jh)),∀(x1,x2)∈Γv. | (3.13) |
The numerical results presented in this Section are obtained with the parameter values described in Table 1 which, for convenience we call them 'baseline parameters'. Whenever we vary these parameters, we state clearly the new values we use for those simulations. Note that these baseline parameters are based on other papers or on our own estimates. For instance, using the GOG hypothesis, we estimated that Dcp is likely much smaller than Dcm. Since we could not find an exact value for Dcp (Dcm was assumed to be 0.00035, as in [43]), we arbitrarily estimated Dcp=10−5.
Param. | Value | Description | Reference |
Dcm | 0.00035 | Uninfected migrating cancer cell diffusion coefficient | [43] |
Dim | 0.0054 | Infected migrating cancer cell diffusion coefficient | [54] |
Dip | 0.00054 | Infected proliferative cancer cell diffusion coefficient | Estimated |
Dcp | 10−5 | Uninfected proliferative cancer cell diffusion coefficient | Estimated |
Dv | 0.0036 | Constant diffusion coefficient for OV | [54] |
ηcp | 0.00285 | Infected proliferative cancer cell haptotaxis coefficient | Estimated |
ηim | 0.0285 | Infected migrating cancer cell haptotaxis coefficient | [29] |
ηip | 0.00285 | Infected proliferative cancer cell haptotaxis coefficient | Estimated |
ηv | 0.0285 | OV haptotaxis coefficient | [29] |
μm | 0.5 | Proliferation rate for uninfected migrating cancer cells | [41] |
μp | 0.75 | Proliferation rate for uninfected proliferative cancer cells | [41] |
Scmcm | 0.1 | Maximum rate of cell-cell adhesion strength | [44] |
Scpcp | 0.05 | Maximum rate of cell-cell adhesion strength | Estimated |
Simim | 0.1 | Maximum rate of cell-cell adhesion strength | Estimated |
Sipip | 0.05 | Maximum rate of cell-cell adhesion strength | Estimated |
Scme | 0.5 | Rate of Cell-ECM adhesion strength | [55] |
Scpe | 0.001 | Rate of Cell-ECM adhesion strength | Estimated |
Sime | 0.5 | Rate of Cell-ECM adhesion strength | Estimated |
Sipe | 0.001 | Rate of Cell-ECM adhesion strength | Estimated |
ω1 | 0.1 | Rate of switching from proliferative state (cp) to migration state (cm) | Estimated |
ω2 | 0.4 | Rate of switching from migration (cm) to proliferative state (cp). | Estimated |
αcm | 0.075 | ECM degradation rate by uninfected cancer cells | Estimated |
αim | αcm2 | ECM degradation rate by infected cancer cells | [29] |
αcp | 0.075 | ECM degradation rate by uninfected cancer cells | Estimated |
αip | αcm2 | ECM degradation rate by infected cancer cells | Estimated |
μ2 | 0.02 | Remodelling term coefficient | [34] |
ϱm | 0.079 | Infection rate of cm cells by OV | [29] |
ϱp | 0.079 | Infection rate of cells by OV | Estimated |
δim | 0.05 | Death rate of infected cancer cells | [54] |
δip | 0.05 | Death rate of infected cancer cells | Estimated |
bm | 40 | Replicating rate of OVs in infected cancer cells cm | Estimated |
bp | 40 | Replicating rate of OVs in infected cancer cells cp | Estimated |
δv | 0.05 | Death rate of OV | [54] |
νe | 1 | The fraction of physical space occupied by the ECM | [47] |
νc | 1 | The fraction of physical space occupied by cancer cells | [47] |
γcm | 1.5 | MDEs secretion rate by uninfected cancer cell | [56] |
γim | 1 | MDEs secretion rate by infected cancer cell | [56] |
γcp | 1 | MDEs secretion rate by uninfected cancer cell | Estimated |
γip | 1.5 | MDEs secretion rate by infected cancer cell | Estimated |
Dm | 0.004 | MDE diffusion coefficient | [57] |
We start in Section 4.1 by investigating numerically the impact of local vs. nonlocal approaches used to describe the cell-cell and cell-matrix adhesion flux. Then, in Section 4.2, we investigate the impact of varying the adhesion strength in the non-local cell flux. Following that we focus on the system of Eq (3.1) without haptotaxis for cp (i.e.,ηcp=0), to investigate the impact of varying different parameters: in Section 4.3 we vary the impact of transition rate between migrating and proliferative cells, in Section 4.4 we vary the impact of OVs infection rate, and in Section 4.5 we vary the impact of OVs replication rate.
First, we focus on model (3.1), described in detail in Section 3.2. In Figure 4(a) we show the dynamics of our multiscale model in the absence of haptotactic terms for the proliferative uninfected cells (ηcp=0), while in Figure 4(b) we show the dynamics of this model in the presence of such haptotactic terms (where ηcp=0.00285, as given in Table 1). We can see that, for the parameter values used in these simulations, there is no difference in the spatial distribution of migrating uninfected or infected cancer cells between panels (a) and (b). However, the addition of haptotactic movement impacts the spatial distribution of proliferative uninfected cancer cells, leading to a more localised cancer cells distribution.
Next, we investigate numerically the dynamics of models (3.2) and (3.3). In Figure 5(a) we show the dynamics of our multi-scale model (3.2) that has two non-local fluxes cm and cp, while in Figure 5(b) we show what happens when we consider non-local fluxes for all cancer cells subpopulations (i.e., model (3.3)). Clearly there is a difference between these results and the previous one. The cancer cells for the case with two non-local fluxes (i.e., for proliferative cp and migrating cm cells) are more invasive, but have lower densities compared to the case where we assume four non-local adhesion fluxes. We can also see that the OV density for the case described by model (3.2) (with two nonlocal fluxes) is almost double compared to the OV density for the case described by model (3.3) (with four nonlocal fluxes).
In this subsection we investigate the effect of cell-cell and cell-matrix adhesion strengths for the two non-local subpopulations (i.e., model (3.2)) versus the four non-local subpopulations (i.e., model (3.3)). In [34] the authors studied the impact of different adhesion strengths in a model with one homogeneous cancer population and showed that when cell-cell adhesion strength was lower than cell-matrix adhesion strength it led to larger tumour spread. Since here we focus on two different cancer cell sub-populations (i.e., migrating and proliferative), we assume that cell-cell adhesion strength is lower than cell-matrix adhesion strength for the migrating cancer cells cm,im (to allow for cell migration), and the other way around for the proliferative cancer cells cp,ip (to reduce cell migration). The results of numerical simulations with these different adhesion strengths are shown in Figure 5. In this case we see relatively similar tumour spread patterns for (a) model (3.2) with two non-local sub-populations and for (b) model (3.3) with four non-local sub-populations. The only difference is a slight increase in the density for uninfected cancer cells and a decrease in the density of infected cancer cells for the model in sub-panels (b).
Since it is difficult to measure the adhesion strengths for cells with different phenotypes, in Figure 6 we also investigate numerically what happens with tumour and virus spread patterns when we assume that all cancer subpopulations have similar cell-cell adhesion strengths that are lower than their cell-matrix adhesion strengths. In this case we see that the cancer cells show less spatial spread compare to the case in Figure 5. Moreover, the OV (which has the highest density in the middle of the tumour mass) cannot destroy the tumour in that region; this is more evident in sub-panels (b) (for model (3.3) with four non-local sub-populations), where the level of the virus is also very reduced. In sub-panels (a) (for model (3.2) with two non-local sub-populations) we still see a bit of reduction in tumour size in the middle of the tumour region where the level of OV is similar as in Figure 5.
We conclude from these two numerical studies that the magnitudes of cell-cell and cell-matrix adhesion strengths for different cancer cell phenotypes (here migrating and proliferative cells), combined with their local/non-local character, influence significantly the spread of the virus through the tumour.
In the next three sub-sections we return to model (3.1) without haptotaxis for cp cells (i.e., ηcp=0), and investigate the impact of transition rates between migrating and proliferative cell sub-populations, as well as the impact of virus infection and replication rates.
We return now to model (3.1) without haptotaxis for cp (i.e., ηcp=0), and investigate numerically the impact of varying the transition rates between migrating and proliferating cancer cells, and the differences from the baseline results shown before in Figure 4(a). In Figure 7 we investigate the spatial spread of tumour and virus populations when (a) ω2=ω1=0.1, and (b) ω2=ω14=0.025. We see that decreasing ω2 leads to an increase in the density of migrating cells (cm,im, in sub-panel (b)) compared to the case in Figure 4(a). Moreover, we see an increase in the spatial spread of the tumour between sub-panel (a) and sub-panel (b) where there are more migrating cancer cells.
In Figure 8 we investigate the impact of the infection rates of cancer cells by the virus particles for the model (3.1) when we ignore the haptotaxis for cp (i.e., ηcp=0); we compare the results with those in Figure 7(b). In Figure 8(a) we assume that the proliferating cells have a faster infection rates compared to the migrating cells: ϱp=3ϱm=0.316. In Figure 8(b) we assume that the migrating cells have a faster infection rates compared to the proliferating cells: ϱm=3ϱp=0.316. We see that by increasing the OV infection rate for any cancer subpopulation it leads to an increase viral density, better viral spread and better killing of cancer cells. This cancer-killing effect is slightly more pronounced in sub-panels (a) where ϱp>ϱm.
In Figure 9 we investigate the impact of varying the OV replication rate for model (3.1) without cp haptotaxis (i.e., ηcp=0), and the results are compared to Figure 7(b). When (a) bp=50≥bm=40, the impact of increasing bp by a small amount is more evident on the proliferative cancer population cp and on the virus density. When (b) bp=bm8=5 and bm=40, it is clear that decreasing bp while keeping bm fixed leads to a very low OV density and a higher density of proliferative cells cp in the middle of the tumour mass compared to the case (a) when bp>bm. Compared to the results in Figure 7(b) we deduce that increasing bp leads to a reduction in cp but not cm and an increase in virus v levels, while decreasing bp leads to an increase in both cp and cm and a drastic reduction in virus v levels.
In this study we proposed a new multiscale moving boundary model that considers the local/non-local interactions between cancer cells and ECM, as well as the infections of cancer cells with oncolytic viruses (OV), all in the context of the go or grow hypothesis. This model generalises the previous studies in [34] (that focused on nonlocal multi-scale moving boundary models for oncolytic virotherapies in the context of a homogeneous cancer population) and [29,58] (that focused on local multiscale moving boundary models for oncolytic virotherapies in the context of a homogeneous cancer population). Here, we consider a heterogeneous cancer cell population formed of two sub-populations: mainly-migrating and mainly-proliferative cells.
Using this new model, we investigated not only the impact of different cell-cell and cell-ECM interaction strengths on the overall spread of cancer cells and OVs (see Figures 4-6), but also the effect of changes in the transition rates between the migrating and proliferative cells (see Figure 7), as well as the effects of varying the infection rates of different cancer cells (Figure 8), and the proliferation rates of viruses inside different cancer cells (Figure 9). First, we have seen that the magnitudes of cell-cell and cell-matrix adhesion strengths for different cancer cell phenotypes (i.e., migrating and proliferative cells), combined with their local/non-local character, influence significantly the spread of the virus through the tumour. Second, we have seen that the killing of cancer cells by the OVs is slightly more pronounced when the proliferating cells have a faster infection rate compared to the migrating cells (i.e., ϱp>ϱm). This suggests that giving the virus during a certain time interval, when the majority of cells in the solid tumour are in a proliferative phase, might eventually lead to better cancer killing. Finally, we have seen that viral replication inside proliferating cells (and viral burst size from these cells) might affect in some cases not only the density of proliferative cells but also the density of migrating cells. For example, when bp>bm, the migrating cells do not seem to be greatly impacted; however, for bp<bm, the migrating cells are impacted.
To conclude, we emphasise that the heterogeneity of solid cancers (formed of sub-populations of cells with different phenotypes; e.g., mainly-migrating and mainly-proliferative cells) might impact the success of oncolytic therapies, where the virus needs to spread throughout the tumour to be able to eliminate it. We would also like to suggest that one possible explanation for the contradictory experimental results in regard to validity of the go or grow hypothesis for various cancer cell lines (see our discussion in the Introduction) might be related to the differences in the heterogeneity of tumours for these different cell lines. However, this hypothesis will have to be tested experimentally in the future.
The first author would like to acknowledge the financial support received from the Saudi Arabian Cultural Bureau in the UK on behalf of Taibah University, Medina, Saudi Arabia.
All authors declare no conflicts of interest in this paper.
In order to carry out the macro-scale computation exclusively on the developing tumour Ω(t0), for any t0>0, we define the spatial cancer indicator function I(⋅,⋅):{1,...,M}×{1,...,M}→{0,1} given by
I(s,p):={1if(xs,xp)∈Ω(t0),0if(xs,xp)∉Ω(t0). | (A.1) |
Further, in order to identify the immediate outside nodes that are neighbours to the tumour boundary (which are needed during the computation), we define the spatially closest outside neighbour indicator functions Hx,+1(⋅,⋅), Hx,−1(⋅,⋅), Hy,+1(⋅,⋅), Hy,−1(⋅,⋅):{2,...,M−1}×{2,...,M−1}→{0,1}, defined by
Hx,±1(s,p):=|I(s,p)−I(s,p±1)|⋅I(s,p),Hy,±1(s,p):=|I(s±1,p)−I(s,p)|⋅I(s,p). | (A.2) |
These enable us to exercise the computations for the cancer cells dynamics only on the expanding cancer region Ω(t0). Indeed, these indicator functions allow us to take advantage of the zero-flux conditions at the moving boundary ∂Ω(t0) and appropriately assign density values that are required in computations (and that are dictated by the boundary conditions) at each of these closest outside points to the tumour boundary (along each spatial direction). Finally, these closest outside points to the tumour boundary are given by the union of pre-images H−1x,−1({1})∪H−1x,+1({1})∪H−1y,−1({1})∪H−1y,+1({1}).
This section explain finite difference-midpoint method used through the paper, into two subsections local flux and non-local flux to accounts for all fluxes considered in this paper.
At any spatial discretised spatial location (x1s,x2p)∈Ω(t0), and any discretised time tt:=t0+lδt, ∀l∈{0,…,[Δt/δt]}, we denote by cls,p the discretised densities at the ((x1s,x2p),tl) for each of the subpopulations c∈{cp,ip,cm,im}. Similar notations we adopt also for the discretised adhesion fluxes at ((x1s,x2p),tl), namely Alc,s,p stands for the discretised adhesion flux for each of the subpopulations c∈{cp,ip,cm,im}. In this context, using the zero-flux conditions across the tumour's moving boundary (accounted here for via the indicators in equations Eqs (A.1) and (A.2)), we are able to cary out computations on the expanding spatial mesh by involving midpoint approximations for the cancer subpopulation densities
cls,p±12:=cls,p+[Hx,±1(s,p)cls,p+I(s,p±1)cls,p±1]2,cls±12,p:=cls,p+[Hy,±1(s,p)cls,p+I(s±1,p)cls±1,p]2, | (B.1) |
as well as for the adhesion fluxes
Alc,s,p±12:=Alc,s,p+[Hx,±1(s,p)Alc,s,p+I(s,p±1)Alc,s,p±1]2,Alc,s±12,p:=Alc,s,p+[Hy,±1(s,p)Alc,s,p+I(s±1,p)Alc,s±1,p]2, | (B.2) |
while the central differences at the virtual midpoint nodes (s,p±12) and (s±12,p) are given by:
● for c:
[cx]ls,p+12:=[Hx,+1(s,p)cls,p+I(s,p+1)cls,p+1]−cls,pΔx,[cx]ls,p−12:=cls,p−[Hx,−1(s,p)cls,p+I(s,p−1)cls,p−1]Δx,[cy]ls+12,p:=[Hy,+1(s,p)cls,p+I(s+1,p)cls+1,p]−cls,pΔy,[cy]ls−12,p:=cls,p−[Hy,−1(s,p)cls,p+I(s−1,p)cls−1,p]Δy, | (B.3) |
Hence, the discretisation of the spatial operator ∇⋅[Dc∇c−cAc(t,x,u(t,⋅))] in Eq (2.25c) is obtained by
(∇⋅[Dc∇c−cA(t,x,u(t,⋅))])ls,p≈Dc([cx]ls,p+12−[cx]ls,p−12)−cls,p+12⋅Alc,s,p+12+cls,p−12⋅Alc,s,p−12Δx+Dc([cy]ls+12,p−[cy]ls−12,p)−cls+12,p⋅Alc,s+12,p+cls−12,p⋅Alc,s−12,pΔy. | (B.4) |
Denoting now by Flc,s,p the discretised value of the flux Fc:=Dc∇c−cAc(t,x,u(t,⋅)) at the spatio-temporal node ((xs,xp),tl), we observe that the discretisation of ∇⋅Fc=∇⋅[Dc∇c−cAc(t,x,u(t,⋅))] given in Eq (2.25c) can therefore be equivalently expressed in a compact form as
(∇⋅Fc)ls,p≃Flc,s,p+12−Flc,s,p−12+Flc,s+12,p+Flc,s−12,ph, | (B.5) |
where
Flc,s,p±12=Dc[cx]ls,p±12−cls,p±12⋅Alc,s,p±12,Flc,s±12,p=Dc[cy]ls±12,p−cls±12,p⋅Alc,s±12,p. |
For the case when the spatial flux is given through the local operator Fc:=DcΔc−ηc∇⋅c∇e) where c∈{cp,ip,im}, we have the discretisation
(Fc)ls,p≈Dc([cx]ls,p+12−[cx]ls,p−12)−ηccls,p+12⋅[ex]ls,p+12+ηccls,p−12⋅[ex]ls,p−12Δx+Dc([cy]ls+12,p−[cy]ls−12,p)−ηccls+12,p⋅[ey]ls+12,p+ηccls−12,p⋅[ey]ls−12,pΔy, | (B.6) |
where
cls,p±12:=cls,p+[Hx,±1(s,p)cls,p+I(s,p±1)cls,p±1]2,cls±12,p:=cls,p+[Hy,±1(s,p)cls,p+I(s±1,p)cls±1,p]2, | (B.7) |
and
[cx]ls,p+12:=[Hx,+1(s,p)cls,p+I(s,p+1)cls,p+1]−cls,pΔx,[cx]ls,p−12:=cls,p−[Hx,−1(s,p)cls,p+I(s,p−1)cls,p−1]Δx,[cy]ls+12,p:=[Hy,+1(s,p)cls,p+I(s+1,p)cls+1,p]−cls,pΔy,[cy]ls−12,p:=cls,p−[Hy,−1(s,p)cls,p+I(s−1,p)cls−1,p]Δy, | (B.8) |
and for e:
[ex]ls,p+12:=els,p+1−els,ph,and[ex]ls,p−12:=els,p−els,p−1h,[ey]ls+12,p:=els+1,p−els,ph,and[ey]ls−12,p:=els,p−els−1,ph. | (B.9) |
Finally, for the haptotactic-like virus spatial operator ηv∇⋅(v∇e) is discretised as
(−ηv∇⋅(v∇e))ls,p≈−ηvvls,p+12⋅[ex]ls,p+12+ηvvls,p−12⋅[ex]ls,p−12Δx+−ηvvls+12,p⋅[ey]ls+12,p+ηvvls−12,p⋅[ey]ls−12,pΔy, | (B.10) |
We denote by Hc(⋅,⋅,⋅) the right-hand side spatial operator for each of the tumour subpopulations Eq 2.25(a)-(d), with the non-transport part of this operator further denoted by fc(u,v). Then, maintaining the same notation style, at any discretised spatio-temporal node ((xs,xp),tl), we have that
Hc(Flc,s,p,cls,p,uls,p):=(∇⋅Fc)ls,p+fc(uls,p,vlp). | (C.1) |
On the time interval [tl,tl+1], we first predict c at tl+12 using an explicit method as follows
˜cl+12s,p=cls,p+Δt2Hc(Flc,s,p,cli,j,uls,p). | (C.2) |
Further, using ˜cl+12s,p we calculate the corresponding predicted flux ˜Fl+12c,s,p at tl+12. Then, we construct a non-local corrector that involves the average of the flux at the active neighbouring spatial locations
{(xs,xp±1)},{(xs±1,xp)},{(xs±1,xp−1)},{(xs±1,xp+1)}∩Ω(t0). | (C.3) |
Denoting the set of indices corresponding to these active locations by N, we we establish a corrector for the spatial flux, given by
F∗l+12c,s,p=1card(N)∑(σ,ζ)∈N˜Fl+12c,σ,ζ, | (C.4) |
which ultimately enables us to use a trapezoidal-type approximation and obtain a corrected value for c at tl+12 as
cl+12s,p=cls,p+Δt4[Hc(Fls,p,cli,j,uls,p)+Hc(F∗l+12s,p,˜cl+12s,p,˜ul+12s,p)], | (C.5) |
Here, ˜ul+12s,p is the "half-time predicted" tumour vector obtained for the current subpopulation c given by the half-time predicted value ˜cl+12s,p, while all the other tumour subpopulations and the ECM (that enter as components of u) being given by their accepted values at ((xs,xp),tl).
Then, using
ˉcl+12s,p:=cl+12s,p+cls,p2, | (C.6) |
to calculate the flux Fl+12c at tl+12, we initiate the predictor-corrector steps described above on the second half of this time interval, which we now repeat on [tl+12,tl+1], and we finally obtain cl+1s,p given through the analogue of Eq (C.5) that results (through the same procedure, described in Eqs (C.2)-(C.5)) on this second half-interval (i.e., on [tl+12,tl+1]. Finally, the same time-marching steps are adopted also for virus equations Eq (2.25f).
[1] | Petroleum B, BP statistical review of world energy, 2015. Available from: www. bp. com/statisticalreview. |
[2] | Sihag S, Pathak H, Jaroli D (2014) Factors affecting the rate of biodegradation of polyaromatic hydrocarbons. Int J Pure App Biosci 2: 185-202. |
[3] |
Stroud JL, Paton GI, Semple KT (2007) Microbe-aliphatic hydrocarbon interactions in soil: implications for biodegradation and bioremediation. J Appl Microbiol 102: 1239-1253. doi: 10.1111/j.1365-2672.2007.03401.x
![]() |
[4] | Das N, Chandran P (2011) Microbial degradation of petroleum hydrocarbon contaminants: an overview. Biotechnol Res Int 2011: 941810. |
[5] |
Gallego JL, Loredo J, Llamas JF, et al. (2001) Bioremediation of diesel-contaminated soils: evaluation of potential in situ techniques by study of bacterial degradation. Biodegradation 12: 325-335. doi: 10.1023/A:1014397732435
![]() |
[6] | Gong R, Hochmuth M, Weatherburn DC (2003) Polycyclic aromatic hydrocarbons and diesel particulates. |
[7] |
Latif I, Karim A, Zuki A, et al. (2010) Pulmonary modulation of benzo [a] pyrene-induced hemato-and hepatotoxicity in broilers. Poultry Sci 89: 1379-1388. doi: 10.3382/ps.2009-00622
![]() |
[8] |
Samanta SK, Singh OV, Jain RK (2002) Polycyclic aromatic hydrocarbons: environmental pollution and bioremediation. Trends Biotechnol 20: 243-248. doi: 10.1016/S0167-7799(02)01943-1
![]() |
[9] |
Huang XD, El-Alawi Y, Penrose DM, et al. (2004) A multi-process phytoremediation system for removal of polycyclic aromatic hydrocarbons from contaminated soils. Environ Pollut 130: 465-476. doi: 10.1016/j.envpol.2003.09.031
![]() |
[10] |
Arthur EL, Rice PJ, Rice PJ, et al. (2005) Phytoremediation—An Overview. CRC Crit Rev Plant Sci 24: 109-122. doi: 10.1080/07352680590952496
![]() |
[11] |
Guo J, Feng R, Ding Y, et al. (2014) Applying carbon dioxide, plant growth-promoting rhizobacterium and EDTA can enhance the phytoremediation efficiency of ryegrass in a soil polluted with zinc, arsenic, cadmium and lead. J Environ Manage 141: 1-8. doi: 10.1016/j.jenvman.2013.12.039
![]() |
[12] | Onwurah I, Ogugua V, Onyike N, et al. (2007) Crude oil spills in the environment, effects and some innovative clean-up biotechnologies. |
[13] |
Yemashova NA, Murygina VP, Zhukov DV, et al. (2007) Biodeterioration of crude oil and oil derived products: a review. Rev Environ Sci Biotechnol 6: 315-337. doi: 10.1007/s11157-006-9118-8
![]() |
[14] |
Head IM, Jones DM, Röling WF (2006) Marine microorganisms make a meal of oil. Nat Rev Microbiol 4: 173-182. doi: 10.1038/nrmicro1348
![]() |
[15] | Yasin G, Bhanger MI, Ansari TM, et al. (2013) Quality and chemistry of crude oils. J Pet Technol Altern Fuels 4: 53-63. |
[16] | Alvarez PJ, Illman WA (2005) Bioremediation and natural attenuation: process fundamentals and mathematical models. John Wiley & Sons. |
[17] |
Martinez-Gomez C, Vethaak A, Hylland K, et al. (2010) A guide to toxicity assessment and monitoring effects at lower levels of biological organization following marine oil spills in European waters. ICES J Mar Sci 67: 1105-1118. doi: 10.1093/icesjms/fsq017
![]() |
[18] |
Haritash A, Kaushik C (2009) Biodegradation aspects of polycyclic aromatic hydrocarbons (PAHs): a review. J Hazard Mater 169: 1-15. doi: 10.1016/j.jhazmat.2009.03.137
![]() |
[19] |
Mishra S, Jyot J, Kuhad RC, et al. (2001) Evaluation of inoculum addition to stimulate in situ bioremediation of oily-sludge-contaminated soil. Appl Environ Microb 67: 1675-1681. doi: 10.1128/AEM.67.4.1675-1681.2001
![]() |
[20] |
Kim KH, Jahan SA, Kabir E, et al. (2013) A review of airborne polycyclic aromatic hydrocarbons (PAHs) and their human health effects. Environ Int 60: 71-80. doi: 10.1016/j.envint.2013.07.019
![]() |
[21] | Masih J, Singhvi R, Kumar K, et al. (2012) Seasonal variation and sources of polycyclic aromatic hydrocarbons (PAHs) in indoor and outdoor air in a semi arid tract of northern India. AAQR 12: 515-525. |
[22] | Perelo LW (2010) Review: in situ and bioremediation of organic pollutants in aquatic sediments. J Hazard Mater 177: 81-89. |
[23] |
Gaspar A, Zellermann E, Lababidi S, et al. (2012) Characterization of saturates, aromatics, resins, and asphaltenes heavy crude oil fractions by atmospheric pressure laser ionization Fourier transform ion cyclotron resonance mass spectrometry. Energ Fuel 26: 3481-3487. doi: 10.1021/ef3001407
![]() |
[24] |
Leyva C, Ancheyta J, Berrueco C, et al. (2013) Chemical characterization of asphaltenes from various crude oils. Fuel Process Technol 106: 734-738. doi: 10.1016/j.fuproc.2012.10.009
![]() |
[25] |
Vinas M, Grifoll M, Sabate J, et al. (2002) Biodegradation of a crude oil by three microbial consortia of different origins and metabolic capabilities. J Ind Microbiol Biot 28: 252-260. doi: 10.1038/sj.jim.7000236
![]() |
[26] |
He L, Lin F, Li X, et al. (2015) Interfacial sciences in unconventional petroleum production: from fundamentals to applications. Chem Soc Rev 44: 5446-5494. doi: 10.1039/C5CS00102A
![]() |
[27] |
Fuentes S, Méndez V, Aguila P, et al. (2014) Bioremediation of petroleum hydrocarbons: catabolic genes, microbial communities, and applications. Appl Microbiol Biot 98: 4781-4794. doi: 10.1007/s00253-014-5684-9
![]() |
[28] |
Dong TT, Lee BK (2009) Characteristics, toxicity, and source apportionment of polycylic aromatic hydrocarbons (PAHs) in road dust of Ulsan, Korea. Chemosphere 74: 1245-1253. doi: 10.1016/j.chemosphere.2008.11.035
![]() |
[29] |
Ou S, Zheng J, Zheng J, et al. (2004) Petroleum hydrocarbons and polycyclic aromatic hydrocarbons in the surficial sediments of Xiamen Harbour and Yuan Dan Lake, China. Chemosphere 56: 107-112. doi: 10.1016/j.chemosphere.2004.02.022
![]() |
[30] |
Chandra S, Sharma R, Singh K, et al. (2013) Application of bioremediation technology in the environment contaminated with petroleum hydrocarbon. Ann Microbiol 63: 417-431. doi: 10.1007/s13213-012-0543-3
![]() |
[31] |
Paruk JD, Adams EM, Uher-Koch H, et al. (2016) Polycyclic aromatic hydrocarbons in blood related to lower body mass in common loons. Sci Total Environ 565: 360-368. doi: 10.1016/j.scitotenv.2016.04.150
![]() |
[32] |
Militon C, Boucher D, Vachelard C, et al. (2010) Bacterial community changes during bioremediation of aliphatic hydrocarbon-contaminated soil. Fems Microbiol Ecol 74: 669-681. doi: 10.1111/j.1574-6941.2010.00982.x
![]() |
[33] |
Wasmund K, Burns KA, Kurtböke DI, et al. (2009) Novel alkane hydroxylase gene (alkB) diversity in sediments associated with hydrocarbon seeps in the Timor Sea, Australia. Appl Environ Microb 75: 7391-7398. doi: 10.1128/AEM.01370-09
![]() |
[34] |
Adgate JL, Goldstein BD, McKenzie LM (2014) Potential public health hazards, exposures and health effects from unconventional natural gas development. Environ Sci Technol 48: 8307-8320. doi: 10.1021/es404621d
![]() |
[35] | Zhu X, Venosa AD, Suidan MT, et al. (2001) Guidelines for the bioremediation of marine shorelines and freshwater wetlands. US EPA |
[36] | Sadler R, Connell D (2003) Analytical methods for the determination of total petroleum hydrocarbons in soil. In: Proceedings of the fifth national workshop on the assessment of site contamination, 133-150. |
[37] | Van Beilen JB, Funhoff EG (2007) Alkane hydroxylases involved in microbial alkane degradation. Appl Microbiol Biot 74: 13-21. |
[38] |
Hatzinger PB, Alexander M (1995) Effect of aging of chemicals in soil on their biodegradability and extractability. Environ Sci Technol 29: 537-545. doi: 10.1021/es00002a033
![]() |
[39] |
Semple KT, Morriss A, Paton G (2003) Bioavailability of hydrophobic organic contaminants in soils: fundamental concepts and techniques for analysis. Eur J Soil Sci 54: 809-818. doi: 10.1046/j.1351-0754.2003.0564.x
![]() |
[40] |
Baboshin M, Golovleva L (2012) Aerobic bacterial degradation of polycyclic aromatic hydrocarbons (PAHs) and its kinetic aspects. Microbiology 81: 639-650. doi: 10.1134/S0026261712060021
![]() |
[41] |
Rojo F (2009) Degradation of alkanes by bacteria. Environ Microbiol 11: 2477-2490. doi: 10.1111/j.1462-2920.2009.01948.x
![]() |
[42] | Wentzel A, Ellingsen TE, Kotlar HK, et al. (2007) Bacterial metabolism of long-chain n-alkanes. Appl Microbiol Biot 76: 1209-1221. |
[43] | Kotani T, Yurimoto H, Kato N, et al. (2007) Novel acetone metabolism in a propane-utilizing bacterium, Gordonia sp. strain TY-5. J Bacteriol 189: 886-893. |
[44] |
Bamforth SM, Singleton I (2005) Bioremediation of polycyclic aromatic hydrocarbons: current knowledge and future directions. J Chem Technol Biot 80: 723-736. doi: 10.1002/jctb.1276
![]() |
[45] |
Kanaly RA, Harayama S (2010) Advances in the field of high-molecular-weight polycyclic aromatic hydrocarbon biodegradation by bacteria. Microb Biotechnol 3: 136-164. doi: 10.1111/j.1751-7915.2009.00130.x
![]() |
[46] |
Seo JS, Keum YS, Li QX (2009) Bacterial degradation of aromatic compounds. Int J Environ Res Public Health 6: 278-309. doi: 10.3390/ijerph6010278
![]() |
[47] | Maigari AU, Maigari MU (2015) Microbial metabolism of polycyclic aromatic hydrocarbons (PAHs): a review. Int J Sci Eng 6: 1449-1459. |
[48] | Rojo F, (2010) Enzymes for aerobic degradation of alkanes, In: Handbook of hydrocarbon and lipid microbiology, Springer, 781-797. |
[49] |
Tortella GR, Diez MC, Durán N (2005) Fungal diversity and use in decomposition of environmental pollutants. Crit Rev Microbiol 31: 197-212. doi: 10.1080/10408410500304066
![]() |
[50] |
Brooijmans RJ, Pastink MI, Siezen RJ (2009) Hydrocarbon-degrading bacteria: the oil-spill clean-up crew. Microb Biotechnol 2: 587-594. doi: 10.1111/j.1751-7915.2009.00151.x
![]() |
[51] |
Germida J, Frick C, Farrell R, et al. (2002) Phytoremediation of oil-contaminated soils. Dev Soil Sci 28: 169-186. doi: 10.1016/S0166-2481(02)80015-0
![]() |
[52] | Wang YN, Cai H, Chi CQ, et al. (2007) Halomonas shengliensis sp. nov., a moderately halophilic, denitrifying, crude-oil-utilizing bacterium. Int J Syst Evol Micr 57: 1222-1226. |
[53] | Mnif S, Chamkha M, Sayadi S (2009) Isolation and characterization of Halomonas sp. strain C2SS100, a hydrocarbon-degrading bacterium under hypersaline conditions. J Appl Microbiol 107: 785-794. |
[54] |
Borzenkov I, Milekhina E, Gotoeva M, et al. (2006) The properties of hydrocarbon-oxidizing bacteria isolated from the oilfields of Tatarstan, Western Siberia, and Vietnam. Microbiology 75: 66-72. doi: 10.1134/S0026261706010127
![]() |
[55] | Dastgheib SMM, Amoozegar MA, Khajeh K, et al. (2011) A halotolerant Alcanivorax sp. strain with potential application in saline soil remediation. Appl Microbiol Biot 90: 305-312. |
[56] |
Chaillan F, Le Flèche A, Bury E, et al. (2004) Identification and biodegradation potential of tropical aerobic hydrocarbon-degrading microorganisms. Res Microbiol 155: 587-595. doi: 10.1016/j.resmic.2004.04.006
![]() |
[57] |
Moody JD, Freeman JP, Fu PP, et al. (2004) Degradation of benzo [a] pyrene by Mycobacterium vanbaalenii PYR-1. Appl Environ Microb 70: 340-345. doi: 10.1128/AEM.70.1.340-345.2004
![]() |
[58] |
Van Beilen JB, Li Z, Duetz WA, et al. (2003) Diversity of alkane hydroxylase systems in the environment. Oil Gas Sci Technol 58: 427-440. doi: 10.2516/ogst:2003026
![]() |
[59] |
McKew BA, Coulon F, Osborn AM, et al. (2007) Determining the identity and roles of oil-metabolizing marine bacteria from the Thames estuary, UK. Environ Microbiol 9: 165-176. doi: 10.1111/j.1462-2920.2006.01125.x
![]() |
[60] |
Pilon-Smits E (2005) Phytoremediation. Annu Rev Plant Biol 56: 15-39. doi: 10.1146/annurev.arplant.56.032604.144214
![]() |
[61] |
Holden P, LaMontagne M, Bruce A, et al. (2002) Assessing the role of Pseudomonas aeruginosa surface-active gene expression in hexadecane biodegradation in sand. Appl Environ Microb 68: 2509-2518. doi: 10.1128/AEM.68.5.2509-2518.2002
![]() |
[62] |
Makadia TH, Adetutu EM, Simons KL, et al. (2011) Re-use of remediated soils for the bioremediation of waste oil sludge. J Environ Manage 92: 866-871. doi: 10.1016/j.jenvman.2010.10.059
![]() |
[63] |
Sheppard PJ, Simons KL, Kadali KK, et al. (2012) The importance of weathered crude oil as a source of hydrocarbonoclastic microorganisms in contaminated seawater. J Microbiol Biotechnol 22: 1185-1192. doi: 10.4014/jmb.1201.01049
![]() |
[64] | Rodríguez-Blanco A, Antoine V, Pelletier E, et al. (2010) Effects of temperature and fertilization on total vs. active bacterial communities exposed to crude and diesel oil pollution in NW Mediterranean Sea. Environ Pollut 158: 663-673. |
[65] |
Sarkar D, Ferguson M, Datta R, et al. (2005) Bioremediation of petroleum hydrocarbons in contaminated soils: comparison of biosolids addition, carbon supplementation, and monitored natural attenuation. Environ Pollut 136: 187-195. doi: 10.1016/j.envpol.2004.09.025
![]() |
[66] |
Tang J, Wang R, Niu X, et al. (2010) Enhancement of soil petroleum remediation by using a combination of ryegrass (Lolium perenne) and different microorganisms. Soil Till Res 110: 87-93. doi: 10.1016/j.still.2010.06.010
![]() |
[67] |
Li X, Wu Y, Lin X, et al. (2012) Dissipation of polycyclic aromatic hydrocarbons (PAHs) in soil microcosms amended with mushroom cultivation substrate. Soil Biol Biochem 47: 191-197. doi: 10.1016/j.soilbio.2012.01.001
![]() |
[68] | Agnello AC, Bagard M, Van Hullebusch ED, et al. (2016) Comparative bioremediation of heavy metals and petroleum hydrocarbons co-contaminated soil by natural attenuation, phytoremediation, bioaugmentation and bioaugmentation-assisted phytoremediation. Sci Total Environ 563: 693-703. |
[69] |
Kadali KK, Simons KL, Sheppard PJ, et al. (2012) Mineralisation of weathered crude oil by a hydrocarbonoclastic consortia in marine mesocosms. Water Air Soil Poll 223: 4283-4295. doi: 10.1007/s11270-012-1191-8
![]() |
[70] |
Yu K, Wong A, Yau K, et al. (2005) Natural attenuation, biostimulation and bioaugmentation on biodegradation of polycyclic aromatic hydrocarbons (PAHs) in mangrove sediments. Mar Pollut Bull 51: 1071-1077. doi: 10.1016/j.marpolbul.2005.06.006
![]() |
[71] |
Margesin R, Hämmerle M, Tscherko D (2007) Microbial activity and community composition during bioremediation of diesel-oil-contaminated soil: effects of hydrocarbon concentration, fertilizers, and incubation time. Microbial Ecol 53: 259-269. doi: 10.1007/s00248-006-9136-7
![]() |
[72] |
Festa S, Coppotelli B, Morelli I (2016) Comparative bioaugmentation with a consortium and a single strain in a phenanthrene-contaminated soil: Impact on the bacterial community and biodegradation. Appl Soil Ecol 98: 8-19. doi: 10.1016/j.apsoil.2015.08.025
![]() |
[73] | Nikolopoulou M, Kalogerakis N, (2010) Biostimulation strategies for enhanced bioremediation of marine oil spills including chronic pollution. In: Handbook of hydrocarbon and lipid microbiology, Springer, 2521-2529. |
[74] | Alexander M (1999) Biodegradation and bioremediation. Gulf Professional Publishing. |
[75] |
Walworth J, Pond A, Snape I, et al. (2007) Nitrogen requirements for maximizing petroleum bioremediation in a sub-Antarctic soil. Cold Reg Sci Technol 48: 84-91. doi: 10.1016/j.coldregions.2006.07.001
![]() |
[76] |
Piehler MF, Swistak J, Pinckney J, et al. (1999) Stimulation of diesel fuel biodegradation by indigenous nitrogen fixing bacterial consortia. Microbial Ecol 38: 69-78. doi: 10.1007/s002489900157
![]() |
[77] |
Li H, Zhao Q, Boufadel MC, et al. (2007) A universal nutrient application strategy for the bioremediation of oil-polluted beaches. Mar Pollut Bull 54: 1146-1161. doi: 10.1016/j.marpolbul.2007.04.015
![]() |
[78] |
Chaillan F, Chaineau C, Point V, et al. (2006) Factors inhibiting bioremediation of soil contaminated with weathered oils and drill cuttings. Environ Pollut 144: 255-265. doi: 10.1016/j.envpol.2005.12.016
![]() |
[79] |
Sayara T, Borràs E, Caminal G, et al. (2011) Bioremediation of PAHs-contaminated soil through composting: Influence of bioaugmentation and biostimulation on contaminant biodegradation. Int Biodeter Biodegr 65: 859-865. doi: 10.1016/j.ibiod.2011.05.006
![]() |
[80] |
Ros M, Rodriguez I, Garcia C, et al. (2010) Microbial communities involved in the bioremediation of an aged recalcitrant hydrocarbon polluted soil by using organic amendments. Bioresour Technol 101: 6916-6923. doi: 10.1016/j.biortech.2010.03.126
![]() |
[81] |
Blyth W, Shahsavari E, Morrison PD, et al. (2015) Biosurfactant from red ash trees enhances the bioremediation of PAH contaminated soil at a former gasworks site. J Environ Manage 162: 30-36. doi: 10.1016/j.jenvman.2015.07.041
![]() |
[82] |
Mair J, Schinner F, Margesin R (2013) A feasibility study on the bioremediation of hydrocarbon-contaminated soil from an alpine former military site: effects of temperature and biostimulation. Cold Reg Sci Technol 96: 122-128. doi: 10.1016/j.coldregions.2013.07.006
![]() |
[83] |
Liu W, Luo Y, Teng Y, et al. (2010) Bioremediation of oily sludge-contaminated soil by stimulating indigenous microbes. Environ Gecochem Hlth 32: 23-29. doi: 10.1007/s10653-009-9262-5
![]() |
[84] |
Palmroth MR, Pichtel J, Puhakka JA (2002) Phytoremediation of subarctic soil contaminated with diesel fuel. Bioresour Technol 84: 221-228. doi: 10.1016/S0960-8524(02)00055-X
![]() |
[85] |
Schaefer M, Juliane F (2007) The influence of earthworms and organic additives on the biodegradation of oil contaminated soil. Appl Soil Ecol 36: 53-62. doi: 10.1016/j.apsoil.2006.11.002
![]() |
[86] |
Suja F, Rahim F, Taha MR, et al. (2014) Effects of local microbial bioaugmentation and biostimulation on the bioremediation of total petroleum hydrocarbons (TPH) in crude oil contaminated soil based on laboratory and field observations. Int Biodeter Biodegr 90: 115-122. doi: 10.1016/j.ibiod.2014.03.006
![]() |
[87] | Al-Sulaimani HS, Al-Wahaibi YM, Al-Bahry S, et al. (2010) Experimental investigation of biosurfactants produced by Bacillus species and their potential for MEOR in Omani oil field. Society of Petroleum Engineers. |
[88] |
Reid BJ, Jones KC, Semple KT (2000) Bioavailability of persistent organic pollutants in soils and sediments—a perspective on mechanisms, consequences and assessment. Environ Pollut 108: 103-112. doi: 10.1016/S0269-7491(99)00206-7
![]() |
[89] |
Liu PWG, Chang TC, Chen CH, et al. (2014) Bioaugmentation efficiency investigation on soil organic matters and microbial community shift of diesel-contaminated soils. Int Biodeter Biodegr 95: 276-284. doi: 10.1016/j.ibiod.2014.05.004
![]() |
[90] |
Hyun S, Ahn MY, Zimmerman AR, et al. (2008) Implication of hydraulic properties of bioremediated diesel-contaminated soil. Chemosphere 71: 1646-1653. doi: 10.1016/j.chemosphere.2008.01.026
![]() |
[91] |
Nam K, Chung N, Alexander M (1998) Relationship between organic matter content of soil and the sequestration of phenanthrene. Environ Sci Technol 32: 3785-3788. doi: 10.1021/es980428m
![]() |
[92] | Alexander M (2000) Aging, bioavailability, and overestimation of risk from environmental pollutants. Environ Sci Technol 34: 4259-4265. |
[93] |
Si-Zhong Y, Hui-Jun J, Zhi W, et al. (2009) Bioremediation of oil spills in cold environments: a review. Pedosphere 19: 371-381. doi: 10.1016/S1002-0160(09)60128-4
![]() |
[94] | Okoh AI (2006) Biodegradation alternative in the cleanup of petroleum hydrocarbon pollutants. Biotechnol Mol Biol Rev 1: 38-50. |
[95] | Margesin R, Schinner F (2001) Biodegradation and bioremediation of hydrocarbons in extreme environments. Appl Microbiol Biot 56: 650-663. |
[96] |
Perfumo A, Banat IM, Marchant R, et al. (2007) Thermally enhanced approaches for bioremediation of hydrocarbon-contaminated soils. Chemosphere 66: 179-184. doi: 10.1016/j.chemosphere.2006.05.006
![]() |
[97] |
Scherr K, Aichberger H, Braun R, et al. (2007) Influence of soil fractions on microbial degradation behavior of mineral hydrocarbons. Eur J Soil Biol 43: 341-350. doi: 10.1016/j.ejsobi.2007.03.009
![]() |
[98] |
Amellal N, Portal J-M, Berthelin J (2001) Effect of soil structure on the bioavailability of polycyclic aromatic hydrocarbons within aggregates of a contaminated soil. Appl Geochem 16: 1611-1619. doi: 10.1016/S0883-2927(01)00034-8
![]() |
[99] |
Chaerun S, Tazaki K (2005) How kaolinite plays an essential role in remediating oil-polluted seawater. Clay Miner 40: 481-491. doi: 10.1180/0009855054040185
![]() |
[100] | Atlas RM, Philp J (2005) Bioremediation. Applied microbial solutions for real-world environmental cleanup. ASM Press. |
[101] |
Zucchi M, Angiolini L, Borin S, et al. (2003) Response of bacterial community during bioremediation of an oil-polluted soil. J Appl Microbiol 94: 248-257. doi: 10.1046/j.1365-2672.2003.01826.x
![]() |
[102] | Tang J, Wang M, Wang F, et al. (2011) Eco-toxicity of petroleum hydrocarbon contaminated soil. J Environ Sci 23: 845-851. |
[103] |
Sheppard PJ, Adetutu EM, Makadia TH, et al. (2011) Microbial community and ecotoxicity analysis of bioremediated, weathered hydrocarbon-contaminated soil. Soil Research 49: 261-269. doi: 10.1071/SR10159
![]() |
1. | Abdulhamed Alsisi, Raluca Eftimie, Dumitru Trucu, Nonlocal multiscale modelling of tumour-oncolytic viruses interactions within a heterogeneous fibrous/non-fibrous extracellular matrix, 2022, 19, 1551-0018, 6157, 10.3934/mbe.2022288 | |
2. | Léon Masurel, Carlo Bianca, Annie Lemarchand, Space-velocity thermostatted kinetic theory model of tumor growth, 2021, 18, 1551-0018, 5525, 10.3934/mbe.2021279 | |
3. | Sabrina Glaschke, Hana M. Dobrovolny, Spatiotemporal spread of oncolytic virus in a heterogeneous cell population, 2024, 183, 00104825, 109235, 10.1016/j.compbiomed.2024.109235 | |
4. | O. E. Adebayo, S. Urcun, G. Rolin, S. P. A. Bordas, D. Trucu, R. Eftimie, Mathematical investigation of normal and abnormal wound healing dynamics: local and non-local models, 2023, 20, 1551-0018, 17446, 10.3934/mbe.2023776 | |
5. | Carlo Bianca, A decade of thermostatted kinetic theory models for complex active matter living systems, 2024, 50, 15710645, 72, 10.1016/j.plrev.2024.06.015 | |
6. | Hana M. Dobrovolny, How do viruses get around? A review of mathematical modeling of in-host viral transmission, 2025, 604, 00426822, 110444, 10.1016/j.virol.2025.110444 |
Param. | Value | Description | Reference |
Dcm | 0.00035 | Uninfected migrating cancer cell diffusion coefficient | [43] |
Dim | 0.0054 | Infected migrating cancer cell diffusion coefficient | [54] |
Dip | 0.00054 | Infected proliferative cancer cell diffusion coefficient | Estimated |
Dcp | 10−5 | Uninfected proliferative cancer cell diffusion coefficient | Estimated |
Dv | 0.0036 | Constant diffusion coefficient for OV | [54] |
ηcp | 0.00285 | Infected proliferative cancer cell haptotaxis coefficient | Estimated |
ηim | 0.0285 | Infected migrating cancer cell haptotaxis coefficient | [29] |
ηip | 0.00285 | Infected proliferative cancer cell haptotaxis coefficient | Estimated |
ηv | 0.0285 | OV haptotaxis coefficient | [29] |
μm | 0.5 | Proliferation rate for uninfected migrating cancer cells | [41] |
μp | 0.75 | Proliferation rate for uninfected proliferative cancer cells | [41] |
Scmcm | 0.1 | Maximum rate of cell-cell adhesion strength | [44] |
Scpcp | 0.05 | Maximum rate of cell-cell adhesion strength | Estimated |
Simim | 0.1 | Maximum rate of cell-cell adhesion strength | Estimated |
Sipip | 0.05 | Maximum rate of cell-cell adhesion strength | Estimated |
Scme | 0.5 | Rate of Cell-ECM adhesion strength | [55] |
Scpe | 0.001 | Rate of Cell-ECM adhesion strength | Estimated |
Sime | 0.5 | Rate of Cell-ECM adhesion strength | Estimated |
Sipe | 0.001 | Rate of Cell-ECM adhesion strength | Estimated |
ω1 | 0.1 | Rate of switching from proliferative state (cp) to migration state (cm) | Estimated |
ω2 | 0.4 | Rate of switching from migration (cm) to proliferative state (cp). | Estimated |
αcm | 0.075 | ECM degradation rate by uninfected cancer cells | Estimated |
αim | αcm2 | ECM degradation rate by infected cancer cells | [29] |
αcp | 0.075 | ECM degradation rate by uninfected cancer cells | Estimated |
αip | αcm2 | ECM degradation rate by infected cancer cells | Estimated |
μ2 | 0.02 | Remodelling term coefficient | [34] |
ϱm | 0.079 | Infection rate of cm cells by OV | [29] |
ϱp | 0.079 | Infection rate of cells by OV | Estimated |
δim | 0.05 | Death rate of infected cancer cells | [54] |
δip | 0.05 | Death rate of infected cancer cells | Estimated |
bm | 40 | Replicating rate of OVs in infected cancer cells cm | Estimated |
bp | 40 | Replicating rate of OVs in infected cancer cells cp | Estimated |
δv | 0.05 | Death rate of OV | [54] |
νe | 1 | The fraction of physical space occupied by the ECM | [47] |
νc | 1 | The fraction of physical space occupied by cancer cells | [47] |
γcm | 1.5 | MDEs secretion rate by uninfected cancer cell | [56] |
γim | 1 | MDEs secretion rate by infected cancer cell | [56] |
γcp | 1 | MDEs secretion rate by uninfected cancer cell | Estimated |
γip | 1.5 | MDEs secretion rate by infected cancer cell | Estimated |
Dm | 0.004 | MDE diffusion coefficient | [57] |
Param. | Value | Description | Reference |
Dcm | 0.00035 | Uninfected migrating cancer cell diffusion coefficient | [43] |
Dim | 0.0054 | Infected migrating cancer cell diffusion coefficient | [54] |
Dip | 0.00054 | Infected proliferative cancer cell diffusion coefficient | Estimated |
Dcp | 10−5 | Uninfected proliferative cancer cell diffusion coefficient | Estimated |
Dv | 0.0036 | Constant diffusion coefficient for OV | [54] |
ηcp | 0.00285 | Infected proliferative cancer cell haptotaxis coefficient | Estimated |
ηim | 0.0285 | Infected migrating cancer cell haptotaxis coefficient | [29] |
ηip | 0.00285 | Infected proliferative cancer cell haptotaxis coefficient | Estimated |
ηv | 0.0285 | OV haptotaxis coefficient | [29] |
μm | 0.5 | Proliferation rate for uninfected migrating cancer cells | [41] |
μp | 0.75 | Proliferation rate for uninfected proliferative cancer cells | [41] |
Scmcm | 0.1 | Maximum rate of cell-cell adhesion strength | [44] |
Scpcp | 0.05 | Maximum rate of cell-cell adhesion strength | Estimated |
Simim | 0.1 | Maximum rate of cell-cell adhesion strength | Estimated |
Sipip | 0.05 | Maximum rate of cell-cell adhesion strength | Estimated |
Scme | 0.5 | Rate of Cell-ECM adhesion strength | [55] |
Scpe | 0.001 | Rate of Cell-ECM adhesion strength | Estimated |
Sime | 0.5 | Rate of Cell-ECM adhesion strength | Estimated |
Sipe | 0.001 | Rate of Cell-ECM adhesion strength | Estimated |
ω1 | 0.1 | Rate of switching from proliferative state (cp) to migration state (cm) | Estimated |
ω2 | 0.4 | Rate of switching from migration (cm) to proliferative state (cp). | Estimated |
αcm | 0.075 | ECM degradation rate by uninfected cancer cells | Estimated |
αim | αcm2 | ECM degradation rate by infected cancer cells | [29] |
αcp | 0.075 | ECM degradation rate by uninfected cancer cells | Estimated |
αip | αcm2 | ECM degradation rate by infected cancer cells | Estimated |
μ2 | 0.02 | Remodelling term coefficient | [34] |
ϱm | 0.079 | Infection rate of cm cells by OV | [29] |
ϱp | 0.079 | Infection rate of cells by OV | Estimated |
δim | 0.05 | Death rate of infected cancer cells | [54] |
δip | 0.05 | Death rate of infected cancer cells | Estimated |
bm | 40 | Replicating rate of OVs in infected cancer cells cm | Estimated |
bp | 40 | Replicating rate of OVs in infected cancer cells cp | Estimated |
δv | 0.05 | Death rate of OV | [54] |
νe | 1 | The fraction of physical space occupied by the ECM | [47] |
νc | 1 | The fraction of physical space occupied by cancer cells | [47] |
γcm | 1.5 | MDEs secretion rate by uninfected cancer cell | [56] |
γim | 1 | MDEs secretion rate by infected cancer cell | [56] |
γcp | 1 | MDEs secretion rate by uninfected cancer cell | Estimated |
γip | 1.5 | MDEs secretion rate by infected cancer cell | Estimated |
Dm | 0.004 | MDE diffusion coefficient | [57] |