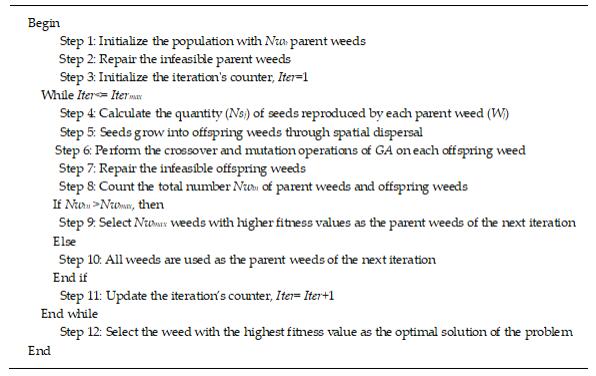
Citation: Shadrack Mubanga Chisenga, Tilahun Seyoum Workneh, Geremew Bultosa, Mark Laing. Proximate composition, cyanide contents, and particle size distribution of cassava flour from cassava varieties in Zambia[J]. AIMS Agriculture and Food, 2019, 4(4): 869-891. doi: 10.3934/agrfood.2019.4.869
[1] | Yixin Zhuo, Ling Li, Jian Tang, Wenchuan Meng, Zhanhong Huang, Kui Huang, Jiaqiu Hu, Yiming Qin, Houjian Zhan, Zhencheng Liang . Optimal real-time power dispatch of power grid with wind energy forecasting under extreme weather. Mathematical Biosciences and Engineering, 2023, 20(8): 14353-14376. doi: 10.3934/mbe.2023642 |
[2] | Ning Zhou, Chen Zhang, Songlin Zhang . A multi-strategy firefly algorithm based on rough data reasoning for power economic dispatch. Mathematical Biosciences and Engineering, 2022, 19(9): 8866-8891. doi: 10.3934/mbe.2022411 |
[3] | Guohao Sun, Sen Yang, Shouming Zhang, Yixing Liu . A hybrid butterfly algorithm in the optimal economic operation of microgrids. Mathematical Biosciences and Engineering, 2024, 21(1): 1738-1764. doi: 10.3934/mbe.2024075 |
[4] | Lihe Liang, Jinying Cui, Juanjuan Zhao, Yan Qiang, Qianqian Yang . Ultra-short-term forecasting model of power load based on fusion of power spectral density and Morlet wavelet. Mathematical Biosciences and Engineering, 2024, 21(2): 3391-3421. doi: 10.3934/mbe.2024150 |
[5] | Lingling Li, Congbo Li, Li Li, Ying Tang, Qingshan Yang . An integrated approach for remanufacturing job shop scheduling with routing alternatives. Mathematical Biosciences and Engineering, 2019, 16(4): 2063-2085. doi: 10.3934/mbe.2019101 |
[6] | Shanshan Pan, Jinbao Jian, Linfeng Yang . Solution to dynamic economic dispatch with prohibited operating zones via MILP. Mathematical Biosciences and Engineering, 2022, 19(7): 6455-6468. doi: 10.3934/mbe.2022303 |
[7] | Bowen Ding, Zhaobin Ma, Shuoyan Ren, Yi Gu, Pengjiang Qian, Xin Zhang . A genetic algorithm with two-step rank-based encoding for closed-loop supply chain network design. Mathematical Biosciences and Engineering, 2022, 19(6): 5925-5956. doi: 10.3934/mbe.2022277 |
[8] | Yanmei Jiang, Mingsheng Liu, Jianhua Li, Jingyi Zhang . Reinforced MCTS for non-intrusive online load identification based on cognitive green computing in smart grid. Mathematical Biosciences and Engineering, 2022, 19(11): 11595-11627. doi: 10.3934/mbe.2022540 |
[9] | Yejun Hu, Liangcai Dong, Lei Xu . Multi-AGV dispatching and routing problem based on a three-stage decomposition method. Mathematical Biosciences and Engineering, 2020, 17(5): 5150-5172. doi: 10.3934/mbe.2020279 |
[10] | Mehrdad Ahmadi Kamarposhti, Ilhami Colak, Kei Eguchi . Optimal energy management of distributed generation in micro-grids using artificial bee colony algorithm. Mathematical Biosciences and Engineering, 2021, 18(6): 7402-7418. doi: 10.3934/mbe.2021366 |
Economic dispatch (ED) [1] in power systems is an important issue for obtaining the steady-state and economic operations of systems that is a typical constrained optimization problem with multiple variables. The optimization goal of the ED problem is to determine the most economic power outputs of generators while satisfying multiple constraints, such as the generation capacity limits, power demand balance, network transmission losses, ramp rate limits and prohibited operating zones. Considering the valve-point effects (VPE) of multivalve steam turbines for the ED problem, the objective cost function is a nonlinear and nonconvex function, which is hard to solve [2]. Especially in large-scale power systems with multiple generators, the ED problem is a complex optimization problem with several local optimal solutions, and thus the global optimal solution is hard to find.
In recent years, several optimization algorithms, including conventional algorithms and meta-heuristic algorithms, have been proposed to solve the ED problems. Some conventional algorithms, such as linear programming (LP) [3], self-adaptive dynamic programming (SADP) [4], iterative dynamic programming (IDP) [1] and evolutionary programming (EP) [5], have been applied to solve the ED problems. These methods solve the ED problems using the simplified optimization model in which the valve-point effects, ramp rate limits, prohibited operating zones and transmission losses are not considered. Moreover, the optimal results obtained by these methods may be the local optima and have lower computational accuracy. The drawbacks of conventional algorithms prompt researchers to study meta-heuristic algorithms for solving ED problems.
Recently, many meta-heuristic algorithms have been proposed to solve the various optimization problems, such as flow shop scheduling [6,7,8], steelmaking scheduling [9], job shop scheduling [10,11,12,13], flexible task scheduling [14] and chiller loading optimization [15,16,17]. Due to the better optimization performance, many meta-heuristic algorithms have also been applied to solve the complex ED problems, and these algorithms include the genetic algorithm (GA) [18,19,20,21], particle swarm optimization (PSO) and its variants [22,23,24,25,26], firefly algorithm (FA) [27], oppositional real coded chemical reaction optimization (ORCCRO) [28], differential evolution (DE) [29,30], chaotic bat algorithm (CBA) [31], oppositional invasive weed optimization (OIWO) [32], teaching learning based optimization (TLBO) [33], tournament-based harmony search (THS) [34], grey wolf optimization (GWO) [35,36], hybrid artificial algae algorithm (HAAA) [37], orthogonal learning competitive swarm optimizer (OLCSO) [2], backtracking search algorithm (BSA) [38], social spider algorithm (SSA) [39], civilized swarm optimization (CSO) [40], kinetic gas molecule optimization (KGMO) [41] and hybrid methods [42,43,44,45]. Although the above meta-heuristic algorithms have been shown to be efficient in solving ED problems, the optimal results obtained by these algorithms are not the most economical.
By mimicking the colonization behavior of weeds in nature, the invasive weed optimization (IWO) algorithm was proposed by Mehrabian and Lucas [46] to optimize multidimensional functions. The experimental results demonstrated that IWO can obtain superior optimization results compared to other evolutionary-based algorithms. Due to its robustness, convergence, high accuracy and searching ability, the IWO algorithm has been applied to solve many engineering optimization problems. However, when IWO is used to solve the ED problem in large-scale power systems, the optimization power outputs of generators obtained by IWO consumes more generation costs compared to the reported methods in literature. To further improve the optimization performance of IWO in solving ED problems, especially ED problems in the large-scale power systems, inspired by the effective application of hybrid methods in solving ED problems [37,42,43,44,45], a hybrid invasive weed optimization (HIWO) algorithm that hybridizes IWO with GA is developed in this study. The motivation behind choosing GA integrated with IWO is to get a better dispatch solution using the crossover operation between offspring weed and its parent weed to improve the local search ability of IWO, and executing the mutation operation on offspring weeds to increase the diversity of the population. The main contributions of this study are as follows: (1) the economic dispatch problem with various practical constraints is investigated by minimizing the total power generation cost; (2) the crossover and mutation operations of GA are proposed to improve the optimization performance of IWO; and (3) an effective repair method of handing constraints is investigated to repair the infeasible dispatch solutions.
The rest of this paper is organized as follows. Section 2 gives the mathematical formulation of the ED problem. Section 3 introduces a hybrid invasive weed optimization (HIWO) algorithm. Section 4 presents the application method of HIWO on ED problems. Section 5 shows the experimental results and analysis on six power systems with different scales. The conclusion is finally given in Section 6.
The ED problem in power systems is to find the optimal dispatch solution of the power outputs of generators, while the total power generation cost of the system is minimized and all the constraints are satisfied.
The optimization objective of the ED problem is to minimize the power generation cost (SC) consumed by N number of generators in the power system, as shown in Eq 1.
Min.SC=N∑i=1Ci(Pi) | (1) |
where Pi and Ci are the power output and generation cost of the ith generator, respectively.
For the ED problem neglecting valve-point effects, Ci is calculated by Eq 2. For the ED problem considering valve-point effects, Eq 3 is used to calculate Ci [2,32].
Ci(Pi)=ai⋅Pi2+bi⋅Pi+ci | (2) |
Ci(Pi)=ai⋅Pi2+bi⋅Pi+ci+|ei⋅sin(fi⋅(Pmini−Pi))| | (3) |
where ai, bi and ci are the cost coefficients of the ith generator; ei and fi are valve-point coefficients of the ith generator;
The feasible dispatch solutions of the ED problem should satisfy the following constraints.
The power output of each generator must be in the range specified by the minimum (
Pmini≤Pi≤Pmaxi | (4) |
The power outputs of generators should satisfy the system power demand (PD). For the ED problem neglecting network transmission losses (PL), the power demand balance is expressed as Eq 5 [30]. For the ED problem considering PL, the power demand balance is expressed as Eq 6.
N∑i=1Pi=PD | (5) |
N∑i=1Pi=PD+PL | (6) |
PL can be calculated using the power flow analysis method [47] or the B-coefficients method [48]. This study adopts the following B-coefficients method to calculate PL.
L=N∑i=1N∑j=1PiBijPj+N∑i=1B0iPi+B00 | (7) |
where Bij, B0i and B00 represent the loss coefficients.
In the actual operation of the power system, to avoid the excessive stress on the boiler and combustion equipment, the change rate of the power output of each generating unit should be within the ramp rate limit, as shown in Eq 8.
{Pi−P0i≤URiP0i−Pi≤DRi | (8) |
where
When taking into account both the generation capacity limits and ramp rate limits, the value range of Pi can be rewritten as Eq 9.
max{Pmini,P0i−DRi}≤Pi≤min{Pmaxi,P0i+URi} | (9) |
Considering the operation limitations of machine components, the power outputs of some generators cannot lie in the prohibited zones, as shown in Eq 10.
Pi∈{Pmini≤Pi≤Pli,1Pui,k−1≤Pi≤Pli,kPui,npi≤Pi≤Pmaxik=2,3,⋯,npi | (10) |
where
IWO is a novel evolutionary computation algorithm based on weed swarm intelligence. By simulating the propagation and growth behaviors of weeds in nature, IWO searches for the optimal solution of the problem in the solution space. The calculation steps of IWO include initialization, reproduction, spatial dispersal and selection. The initial population with Nwo weed individuals is randomly generated in the feasible solution space, in which each weed consisting of variables represents a feasible solution. Then, each weed Wj in the population reproduces seeds, and the seeds grow into offspring weeds through spatial dispersal. The amount (Nsj) of seeds reproduced by Wj is calculated by using Eq 11.
Nsj=Fitj−FitminFitmax−Fitmin⋅(Nsmax−Nsmin)+Nsmin | (11) |
where Fitj is the fitness value of Wj; Fitmin and Fitmax are the minimum and maximum fitness values in the weed population, respectively; Nsmin and Nsmax are the minimum and maximum of the number of seeds, respectively.
The parent weeds with higher fitness values can reproduce more seeds, and they have more offspring weeds in the population. This reproduction strategy means that IWO can converge rapidly and reliably to the approximate optimal solution. Offspring weeds are randomly distributed around their parent weed according to a normal distribution with a standard deviation (σit). The calculation formula of σit is shown in Eq 12. Along with the increase of the iteration times, σit is gradually reduced from an initial value (σiv) to a final value (σfv), which makes the search range of IWO be gradually reduced. This strategy makes IWO have the whole space search capability in early iterations and high local convergence in later iterations. After all the seeds grow into weeds, the Nwmax weeds with higher fitness values are selected from all the weeds as the parent weeds of the next iteration. Through Itermax times iterations, the weed with the highest fitness value is the optimal solution of the problem.
σit=(Itermax−Iter)mItermaxm⋅(σiv−σfv)+σfv | (12) |
where m is the nonlinear modulation index, and Iter and Itermax are the current number and maximum of iterations, respectively.
In the proposed HIWO algorithm, IWO is used to explore the solution space around parent weeds. After the seeds reproduced by parent weeds have grown into offspring weeds, the crossover and mutation operations of GA are performed on offspring weeds for improving the quality and diversity of solutions, which can improve the convergence speed and avoid the premature convergence of the algorithm.
The execution flow of HIWO is represented by the pseudo code shown in Figure 1.
Each offspring weed (OW(j, q)) (q = 1, 2, …, Nsj ) crosses with its parent weed (Wj) to generate a new weed (
(a) If
(b) If
After the new offspring weed (
For each offspring weed (OW(j, q)) (q = 1, 2, …, Nsj ), randomly select X mutation points from N variables
σm=(Pmaxi−Pmini)⋅rand(0,1) | (13) |
where rand (0, 1) is a random number between 0 and 1.
In the proposed HIWO algorithm, the first task is the encoding to represent each solution considering all of the constraints. Each weed (Wj) is represented as a row vector consisting of power outputs of generators, as shown in Eq 14. The weed population is initialized by randomly generating the power outputs of generators by using Eq 15. Then, infeasible weeds are repaired into feasible solutions by using the repair method in Section 4.2. Weeds in the initial population are used as the parent weeds to reproduce seeds, which grows into offspring weeds through spatial dispersal. The weeds with higher fitness value can reproduce more seeds. The fitness function used in this study is shown in Eq 16. Each offspring weed will perform the crossover and mutation procedures, like in the canonical GA, and thus can increase the diversity of the population. Then, the repair procedure is applied on the infeasible offspring weeds to make them satisfy with all of the constraints. If the total quantity of parent weeds and offspring weeds is larger than the specified population size, select the weeds with higher fitness values as the parent weeds of the next iteration. Otherwise, all the weeds are used as parent weeds. After multiple times iterations, the best weed with the highest fitness value is selected as the optimal dispatch solution of the ED problem.
Wj=(P1,P2,⋯,PN) | (14) |
Pi=(Pmaxi−Pmini)⋅rand(0,1)+Pminii=1,2,⋯,N | (15) |
Fitj=1SCj | (16) |
where SCj and Fitj represent the power generation cost and fitness value of the jth weed, respectively.
An effective repair method of handing constraints is proposed in this study to repair infeasible weeds into feasible solutions. The detail repair steps are stated in the following.
Step 1: Modify the
Pi={max{Pmini,P0i−DRi}if Pi<max{Pmini,P0i−DRi}min{Pmaxi,P0i+URi}if Pi>min{Pmaxi,P0i+URi} | (17) |
Step 2: Calculate the constraint violation (V) of the power demand balance. For the ED problem considering transmission losses, V is calculated by using Eq 18. For the ED problem neglecting transmission losses, V is calculated by using Eq 19. If
V=|N∑i=1Pi−PD−N∑i=1N∑j=1PiBijPj−N∑i=1B0iPi−B00| | (18) |
V=|N∑i=1Pi−PD| | (19) |
Step 3: Determine the modification sequence of N generators. For each generator i (i = 1, 2, …, N), calculate the modification value
P′i=PD−∑r∈RPr | (20) |
Bii(P′i)2+(2∑r∈RPrBir+B0i−1)P′i+(PD+∑r∈R∑t∈RPrBrtPt+∑r∈RB0rPr−∑r∈RPr+B00)=0 | (21) |
P′i=−(2∑r∈RPrBir+B0i−1)−√(2∑r∈RPrBir+B0i−1)2−4Bii(PD+∑r∈R∑t∈RPrBrtPt+∑r∈RB0rPr−∑r∈RPr+B00)2Bii | (22) |
For each generator i (i = 1, 2, …, N), assume that the ith generator is selected as the revised generator, and Pi is replaced by
CXi=Ci(P′i)−Ci(Pi) | (23) |
PCVi=CXi−min(CX)max(CX)−min(CX)+PVi−min(PV)max(PV)−min(PV) | (24) |
Step 4: Modify the power output of each generator in turn according to the modification sequence stored in S until the power demand balance constraint is satisfied. When the ith (
Step 5: Output the modified weed (Wj).
To validate the optimization ability of HIWO on ED problems with various practical constraints, six classical ED problems in the small, medium, large and very large-scale power systems were selected as the studied test cases. For each test case, the optimal dispatch results obtained by HIWO in 50 independent runs, including the minimum cost (SCmin), average cost (SCavg), maximum cost (SCmax) and standard deviation of the costs (SCstd), are compared to those of algorithms reported in the literature. The best optimization performance among these algorithms is shown in boldface. The parameters of HIWO on six test systems are set as follows: the initial population size Nwo = 30, maximum population size Nwmax = 50, minimum number of seeds Nsmin = 1, maximum number of seeds Nsmax = 5, nonlinear modulation index m = 5, initial standard deviation
The 15-generator power system [2,24] considering transmission losses, ramp rate limits and prohibited operating zones is selected as the small-scale test system. The power load demand of the system is 2630 MW. In this test system study, the optimal power outputs of generators obtained by HIWO are shown in Table 1.The optimal dispatch results of HIWO are compared to those of OLCSO [2], WCA [49], ICS [50], FA [27], RTO [51], EMA [52] and IWO, as shown in Tables 2. Compared to other algorithms in terms of minimum, average, maximum and standard deviation of costs in 50 runs, the dispatch solution obtained by HIWO consumes the least cost.
Generators | Pi | Generators | Pi | Generators | Pi | Generators | Pi | Generators | Pi |
1 | 455.0000 | 4 | 130.0000 | 7 | 430.0000 | 10 | 159.7871 | 13 | 25.0000 |
2 | 380.0000 | 5 | 170.0000 | 8 | 71.2594 | 11 | 80.0000 | 14 | 15.0000 |
3 | 130.0000 | 6 | 460.0000 | 9 | 58.4944 | 12 | 80.0000 | 15 | 15.0000 |
Algorithms | SCmin ($) | SCavg ($) | SCmax ($) | SCstd |
EMA [52] | 32704.4503 | 32704.4504 | 32704.4506 | NA |
FA [27] | 32704.5000 | 32856.1000 | 33175.0000 | 147.17022 |
ICS [50] | 32706.7358 | 32714.4669 | 32752.5183 | NA |
WCA [49] | 32704.4492 | 32704.5096 | 32704.5196 | 4.513e-05 |
RTO [51] | 32701.8145 | 32704.5300 | 32715.1800 | 5.07 |
OLCSO [2] | 32692.3961 | 32692.3981 | 32692.4033 | 0.0022 |
IWO | 32691.8615 | 32691.9392 | 32692.1421 | 0.0927 |
HIWO | 32691.5614 | 32691.8615 | 32691.8616 | 0.0001 |
The 40-generator power system [32] considering valve-point effects and transmission losses is selected as the medium-scale test system. The power load demand of the system is 10500 MW. The optimal power outputs obtained by HIWO are shown in Table 3. The optimal dispatch results of HIWO are compared to those of ORCCRO [28], BBO [28], DE/BBO [28], SDE [29], OIWO [32], HAAA [37] and IWO, as shown in Tables 4. Compared to other algorithms in the literature, the proposed HIWO algorithm can obtain the cheapest dispatch solution in terms of minimum, average and maximum of costs in 50 runs.
Generators | Pi | Generators | Pi | Generators | Pi | Generators | Pi | Generators | Pi |
1 | 113.9993 | 9 | 289.4281 | 17 | 489.2798 | 25 | 523.2794 | 33 | 190.0000 |
2 | 113.9993 | 10 | 279.5996 | 18 | 489.2793 | 26 | 523.2794 | 34 | 200.0000 |
3 | 120.0000 | 11 | 243.5995 | 19 | 511.2795 | 27 | 10.0000 | 35 | 199.9999 |
4 | 179.7330 | 12 | 94.0000 | 20 | 511.2793 | 28 | 10.0000 | 36 | 164.7999 |
5 | 87.7999 | 13 | 484.0391 | 21 | 523.2794 | 29 | 10.0000 | 37 | 109.9998 |
6 | 139.9998 | 14 | 484.0390 | 22 | 523.2794 | 30 | 87.7999 | 38 | 110.0000 |
7 | 300.0000 | 15 | 484.0393 | 23 | 523.2794 | 31 | 190.0000 | 39 | 109.9999 |
8 | 299.9997 | 16 | 484.0391 | 24 | 523.2794 | 32 | 190.0000 | 40 | 549.9999 |
Algorithms | SCmin ($) | SCavg ($) | SCmax ($) | SCstd |
ORCCRO [28] | 136855.19 | 136855.19 | 136855.19 | NA |
BBO [28] | 137026.82 | 137116.58 | 137587.82 | NA |
DE/BBO [28] | 136950.77 | 136966.77 | 137150.77 | NA |
SDE [29] | 138157.46 | NA | NA | NA |
OIWO [32] | 136452.68 | 136452.68 | 136452.68 | NA |
HAAA [37] | 136433.5 | 136436.6 | NA | 3.341896 |
IWO | 136543.8580 | 137009.5641 | 137679.1073 | 292.9686 |
HIWO | 136430.9504 | 136435.2127 | 136441.1059 | 4.3238 |
To verify the dispatch performance of HIWO on large-scale power systems with multiple local optimal solutions, two cases studies are performed to compare the optimization results of HIWO and other algorithms. The detail information of these two cases is shown as follows.
Case Ⅰ: The 80-generator power system [37] considering valve-point effects. The power load demand is 21000 MW.
Case Ⅱ: The 110-generator power system [20,32] neglecting valve-point effects and transmission losses. The power load demand is 15000 MW.
In the case Ⅰ study, the optimal dispatch solution obtained by HIWO is shown in Table 5. The comparison results of generation costs generated by HIWO, THS [34], CSO [40], HAAA [37], GWO [35] and IWO are summarized in Table 6. It can be found from Table 6 that HIWO can obtain the cheapest dispatch solution compared to other algorithms.
Generators | Pi | Generators | Pi | Generators | Pi | Generators | Pi | Generators | Pi |
1 | 110.8335 | 17 | 489.3362 | 33 | 189.9994 | 49 | 284.6071 | 65 | 523.2794 |
2 | 111.5439 | 18 | 489.2794 | 34 | 165.1983 | 50 | 130.0000 | 66 | 523.2835 |
3 | 97.3834 | 19 | 511.2731 | 35 | 199.9997 | 51 | 94.0040 | 67 | 10.0000 |
4 | 179.7603 | 20 | 511.2666 | 36 | 199.9998 | 52 | 94.0000 | 68 | 10.0000 |
5 | 87.9806 | 21 | 523.2525 | 37 | 109.9999 | 53 | 214.7298 | 69 | 10.0000 |
6 | 139.9997 | 22 | 523.2805 | 38 | 110.0000 | 54 | 394.2675 | 70 | 87.8052 |
7 | 259.5584 | 23 | 523.2794 | 39 | 109.9987 | 55 | 394.2967 | 71 | 190.0000 |
8 | 284.7677 | 24 | 523.2794 | 40 | 511.2603 | 56 | 304.4839 | 72 | 189.9997 |
9 | 284.6331 | 25 | 523.2794 | 41 | 110.9296 | 57 | 489.3082 | 73 | 189.9991 |
10 | 130.0000 | 26 | 523.2958 | 42 | 110.8195 | 58 | 489.2773 | 74 | 164.7786 |
11 | 169.0220 | 27 | 10.0000 | 43 | 97.3706 | 59 | 511.2121 | 75 | 199.9994 |
12 | 94.0000 | 28 | 10.0000 | 44 | 179.7187 | 60 | 511.2992 | 76 | 200.0000 |
13 | 214.7422 | 29 | 10.0000 | 45 | 87.8560 | 61 | 523.2830 | 77 | 109.9990 |
14 | 394.1929 | 30 | 89.6856 | 46 | 139.9995 | 62 | 523.3201 | 78 | 110.0000 |
15 | 394.2794 | 31 | 189.9993 | 47 | 259.6320 | 63 | 523.2794 | 79 | 109.9996 |
16 | 394.3050 | 32 | 189.9992 | 48 | 284.6702 | 64 | 523.2794 | 80 | 511.2482 |
Algorithms | SCmin ($) | SCavg ($) | SCmax ($) | SCstd |
THS [34] | 243192.6899 | 243457.36 | NA | 120.9889 |
CSO [40] | 243195.3781 | 243546.6283 | 244038.7352 | NA |
HAAA [37] | 242815.9 | 242883 | 242944.5 | 29.2849 |
GWO [35] | 242825.4799 | 242829.8192 | 242837.1303 | 0.093 |
IWO | 246386.4038 | 248088.2077 | 249888.0623 | 844.0919 |
HIWO | 242815.2096 | 242836.1110 | 242872.4662 | 10.3458 |
In the case Ⅱ study, the optimal dispatch solution obtained by HIWO is shown in Table 7. The generation cost generated by HIWO are compared to those of ORCCRO [28], BBO [28], DE/BBO [28], OIWO [32], OLCSO [2] and IWO, which are summarized in Table 8. Compared to other algorithms in terms of minimum, average, maximum and standard deviation of costs in 50 runs, the optimal dispatch solution obtained by HIWO generates the least generation cost.
Generators | Pi | Generators | Pi | Generators | Pi | Generators | Pi | Generators | Pi |
1 | 2.4000 | 23 | 68.9000 | 45 | 659.9999 | 67 | 70.0000 | 89 | 82.4977 |
2 | 2.4000 | 24 | 350.0000 | 46 | 616.2499 | 68 | 70.0000 | 90 | 89.2333 |
3 | 2.4000 | 25 | 400.0000 | 47 | 5.4000 | 69 | 70.0000 | 91 | 57.5687 |
4 | 2.4000 | 26 | 400.0000 | 48 | 5.4000 | 70 | 359.9999 | 92 | 99.9986 |
5 | 2.4000 | 27 | 499.9992 | 49 | 8.4000 | 71 | 399.9999 | 93 | 439.9998 |
6 | 4.0000 | 28 | 500.0000 | 50 | 8.4000 | 72 | 399.9998 | 94 | 499.9999 |
7 | 4.0000 | 29 | 199.9997 | 51 | 8.4000 | 73 | 105.2864 | 95 | 600.0000 |
8 | 4.0000 | 30 | 99.9998 | 52 | 12.0000 | 74 | 191.4091 | 96 | 471.5717 |
9 | 4.0000 | 31 | 10.0000 | 53 | 12.0000 | 75 | 89.9996 | 97 | 3.6000 |
10 | 64.5432 | 32 | 19.9993 | 54 | 12.0000 | 76 | 49.9999 | 98 | 3.6000 |
11 | 62.2465 | 33 | 79.9950 | 55 | 12.0000 | 77 | 160.0000 | 99 | 4.4000 |
12 | 36.2739 | 34 | 249.9998 | 56 | 25.2000 | 78 | 295.4962 | 100 | 4.4000 |
13 | 56.6406 | 35 | 359.9999 | 57 | 25.2000 | 79 | 175.0102 | 101 | 10.0000 |
14 | 25.0000 | 36 | 399.9997 | 58 | 35.0000 | 80 | 98.2829 | 102 | 10.0000 |
15 | 25.0000 | 37 | 39.9998 | 59 | 35.0000 | 81 | 10.0000 | 103 | 20.0000 |
16 | 25.0000 | 38 | 69.9996 | 60 | 45.0000 | 82 | 12.0000 | 104 | 20.0000 |
17 | 154.9999 | 39 | 99.9998 | 61 | 45.0000 | 83 | 20.0000 | 105 | 40.0000 |
18 | 154.9993 | 40 | 119.9984 | 62 | 45.0000 | 84 | 199.9999 | 106 | 40.0000 |
19 | 155.0000 | 41 | 157.4299 | 63 | 184.9996 | 85 | 324.9972 | 107 | 50.0000 |
20 | 155.0000 | 42 | 219.9999 | 64 | 184.9996 | 86 | 440.0000 | 108 | 30.0000 |
21 | 68.9000 | 43 | 439.9999 | 65 | 184.9984 | 87 | 14.0886 | 109 | 40.0000 |
22 | 68.9000 | 44 | 559.9998 | 66 | 184.9997 | 88 | 24.0910 | 110 | 20.0000 |
Algorithms | SCmin ($) | SCavg ($) | SCmax ($) | SCstd |
ORCCRO [28] | 198016.29 | 198016.32 | 198016.89 | NA |
BBO [28] | 198241.166 | 198413.45 | 199102.59 | NA |
DE/BBO [28] | 198231.06 | 198326.66 | 198828.57 | NA |
OIWO [32] | 197989.14 | 197989.41 | 197989.93 | NA |
OLCSO [2] | 197988.8576 | 197989.5832 | 197990.4551 | 0.3699 |
IWO | 198252.3594 | 198621.3233 | 198902.7697 | 138.4714 |
HIWO | 197988.1927 | 197988.1969 | 197988.2045 | 0.0025 |
To investigate the dispatch performance of HIWO on very large-scale power systems, the following two cases studies are performed for comparing the optimization results of HIWO and other algorithms.
Case Ⅰ: The 140-generator Korea power system [23,32] neglecting transmission losses. The 12 generators consider the valve point effects. The power load demand is 49342 MW.
Case Ⅱ: The 160-generator power system [32] considering valve-point effects. The power load demand is 43200 MW.
In the case Ⅰ study, the optimal dispatch solution obtained by HIWO is shown in Table 9. The optimal results of HIWO are compared to those of SDE [29], OIWO [32], HAAA [37], GWO [35], KGMO [41] and IWO, as shown in Table 10. The corrected optimal result of OIWO is shown in italics. Compared to other algorithms in terms of minimum, average, maximum and standard deviation of costs in 50 runs, HIWO can obtain the cheapest dispatch solution.
Generators | Pi | Generators | Pi | Generators | Pi | Generators | Pi | Generators | Pi |
1 | 115.2442 | 29 | 500.9998 | 57 | 103.0000 | 85 | 115.0000 | 113 | 94.0000 |
2 | 189.0000 | 30 | 500.9994 | 58 | 198.0000 | 86 | 207.0000 | 114 | 94.0000 |
3 | 190.0000 | 31 | 505.9993 | 59 | 311.9941 | 87 | 207.0000 | 115 | 244.0000 |
4 | 190.0000 | 32 | 505.9997 | 60 | 281.1604 | 88 | 175.0000 | 116 | 244.0000 |
5 | 168.5393 | 33 | 506.0000 | 61 | 163.0000 | 89 | 175.0000 | 117 | 244.0000 |
6 | 189.9932 | 34 | 505.9998 | 62 | 95.0000 | 90 | 175.0000 | 118 | 95.0000 |
7 | 489.9992 | 35 | 499.9996 | 63 | 160.0000 | 91 | 175.0000 | 119 | 95.0000 |
8 | 489.9996 | 36 | 500.0000 | 64 | 160.0000 | 92 | 579.9998 | 120 | 116.0000 |
9 | 495.9997 | 37 | 240.9993 | 65 | 489.9465 | 93 | 645.0000 | 121 | 175.0000 |
10 | 495.9994 | 38 | 240.9999 | 66 | 196.0000 | 94 | 983.9998 | 122 | 2.0000 |
11 | 495.9997 | 39 | 773.9996 | 67 | 489.9717 | 95 | 977.9993 | 123 | 4.0000 |
12 | 496.0000 | 40 | 769.0000 | 68 | 489.9908 | 96 | 681.9997 | 124 | 15.0000 |
13 | 506.0000 | 41 | 3.0000 | 69 | 130.0000 | 97 | 719.9998 | 125 | 9.0000 |
14 | 509.0000 | 42 | 3.0000 | 70 | 234.7202 | 98 | 717.9993 | 126 | 12.0000 |
15 | 506.0000 | 43 | 249.2474 | 71 | 137.0000 | 99 | 719.9997 | 127 | 10.0000 |
16 | 504.9997 | 44 | 246.0287 | 72 | 325.4950 | 100 | 963.9998 | 128 | 112.0000 |
17 | 505.9997 | 45 | 249.9973 | 73 | 195.0000 | 101 | 958.0000 | 129 | 4.0000 |
18 | 505.9997 | 46 | 249.9863 | 74 | 175.0000 | 102 | 1006.9992 | 130 | 5.0000 |
19 | 504.9994 | 47 | 241.0622 | 75 | 175.0000 | 103 | 1006.0000 | 131 | 5.0000 |
20 | 505.0000 | 48 | 249.9950 | 76 | 175.0000 | 104 | 1012.9999 | 132 | 50.0000 |
21 | 504.9998 | 49 | 249.9916 | 77 | 175.0000 | 105 | 1019.9996 | 133 | 5.0000 |
22 | 505.0000 | 50 | 249.9995 | 78 | 330.0000 | 106 | 953.9999 | 134 | 42.0000 |
23 | 504.9998 | 51 | 165.0000 | 79 | 531.0000 | 107 | 951.9998 | 135 | 42.0000 |
24 | 504.9996 | 52 | 165.0000 | 80 | 530.9995 | 108 | 1005.9996 | 136 | 41.0000 |
25 | 536.9997 | 53 | 165.0000 | 81 | 398.6524 | 109 | 1013.0000 | 137 | 17.0000 |
26 | 536.9995 | 54 | 165.0000 | 82 | 56.0000 | 110 | 1020.9998 | 138 | 7.0000 |
27 | 548.9998 | 55 | 180.0000 | 83 | 115.0000 | 111 | 1014.9996 | 139 | 7.0000 |
28 | 548.9993 | 56 | 180.0000 | 84 | 115.0000 | 112 | 94.0000 | 140 | 26.0000 |
Algorithms | SCmin ($) | SCavg ($) | SCmax ($) | SCstd |
SDE [29] | 1560236.85 | NA | NA | NA |
OIWO [32] | 1559712.2604 | NA | NA | NA |
HAAA [37] | 1559710.00 | 1559712.87 | 1559731.00 | 4.1371 |
GWO [35] | 1559953.18 | 1560132.93 | 1560228.40 | 1.024 |
KGMO [41] | 1583944.60 | 1583952.14 | 1583963.52 | NA |
IWO | 1564050.0027 | 1567185.2227 | 1571056.6280 | 1678.8488 |
HIWO | 1559709.5266 | 1559709.6956 | 1559709.8959 | 0.0856 |
In the case Ⅱ study, the optimal dispatch solution obtained by HIWO is shown in Table 11. The optimal results of HIWO are compared to those of ORCCRO [28], BBO [28], DE/BBO [28], CBA [31], OIWO [32] and IWO, as shown in Table 12. Compared to other algorithms, HIWO can also obtain the cheapest dispatch solution in terms of minimum, average, maximum and standard deviation of costs.
Generators | Pi | Generators | Pi | Generators | Pi | Generators | Pi | Generators | Pi |
1 | 218.6095 | 33 | 280.6560 | 65 | 279.6118 | 97 | 287.7203 | 129 | 431.0758 |
2 | 209.2361 | 34 | 238.9676 | 66 | 238.5645 | 98 | 238.6988 | 130 | 275.8790 |
3 | 279.6486 | 35 | 279.9554 | 67 | 287.7296 | 99 | 426.2750 | 131 | 219.6189 |
4 | 240.3113 | 36 | 240.9831 | 68 | 241.2519 | 100 | 272.6741 | 132 | 210.4739 |
5 | 280.0206 | 37 | 290.1069 | 69 | 427.7708 | 101 | 217.5647 | 133 | 281.6640 |
6 | 238.4301 | 38 | 240.0425 | 70 | 272.9907 | 102 | 211.9593 | 134 | 238.9676 |
7 | 288.2326 | 39 | 426.3102 | 71 | 218.5918 | 103 | 280.6578 | 135 | 276.5752 |
8 | 239.5051 | 40 | 275.6392 | 72 | 212.7020 | 104 | 239.2363 | 136 | 239.3707 |
9 | 425.6549 | 41 | 219.6195 | 73 | 281.6629 | 105 | 276.3263 | 137 | 287.7806 |
10 | 275.6903 | 42 | 210.9690 | 74 | 238.9676 | 106 | 240.7144 | 138 | 238.5645 |
11 | 217.5646 | 43 | 282.6711 | 75 | 279.3688 | 107 | 290.0715 | 139 | 430.7874 |
12 | 212.4544 | 44 | 240.3113 | 76 | 237.6239 | 108 | 238.8332 | 140 | 275.8606 |
13 | 280.6558 | 45 | 279.7868 | 77 | 289.9995 | 109 | 425.7918 | 141 | 218.6539 |
14 | 238.6988 | 46 | 237.4895 | 78 | 239.9082 | 110 | 275.2705 | 142 | 210.7215 |
15 | 279.9370 | 47 | 287.7274 | 79 | 425.2406 | 111 | 217.5671 | 143 | 281.6640 |
16 | 240.7144 | 48 | 240.0425 | 80 | 276.0112 | 112 | 212.2069 | 144 | 239.3707 |
17 | 287.6968 | 49 | 427.4497 | 81 | 218.5923 | 113 | 281.6664 | 145 | 276.3578 |
18 | 239.7738 | 50 | 275.6817 | 82 | 212.2069 | 114 | 239.6394 | 146 | 239.6394 |
19 | 427.4049 | 51 | 219.6197 | 83 | 282.7049 | 115 | 276.0940 | 147 | 287.7565 |
20 | 275.6990 | 52 | 213.4447 | 84 | 237.7582 | 116 | 240.3113 | 148 | 239.3707 |
21 | 217.5665 | 53 | 282.6717 | 85 | 279.7940 | 117 | 290.0972 | 149 | 426.3023 |
22 | 212.2069 | 54 | 237.8926 | 86 | 239.3707 | 118 | 239.5051 | 150 | 275.6371 |
23 | 283.6805 | 55 | 276.2856 | 87 | 290.0916 | 119 | 429.4367 | 151 | 217.5647 |
24 | 239.7738 | 56 | 239.5051 | 88 | 239.2363 | 120 | 275.6690 | 152 | 212.2069 |
25 | 279.9011 | 57 | 287.6883 | 89 | 427.0504 | 121 | 217.5656 | 153 | 279.6493 |
26 | 240.9831 | 58 | 238.5645 | 90 | 275.7937 | 122 | 210.2264 | 154 | 238.4301 |
27 | 290.0737 | 59 | 429.9489 | 91 | 217.5643 | 123 | 280.6617 | 155 | 279.9078 |
28 | 240.8488 | 60 | 275.5096 | 92 | 212.9496 | 124 | 239.7738 | 156 | 240.4457 |
29 | 427.1007 | 61 | 218.5915 | 93 | 282.6732 | 125 | 275.9409 | 157 | 287.7385 |
30 | 276.2995 | 62 | 212.9496 | 94 | 240.4457 | 126 | 240.1769 | 158 | 238.5645 |
31 | 219.6189 | 63 | 282.6705 | 95 | 279.4854 | 127 | 287.6965 | 159 | 426.9110 |
32 | 211.7117 | 64 | 239.9082 | 96 | 240.1769 | 128 | 238.4301 | 160 | 272.7775 |
Algorithms | SCmin ($) | SCavg ($) | SCmax ($) | SCstd |
ORCCRO [28] | 10004.20 | 10004.21 | 10004.45 | NA |
OIWO [32] | 9981.9834 | 9982.991 | 9983.998 | NA |
BBO [28] | 10008.71 | 10009.16 | 10010.59 | NA |
DE/BBO [28] | 10007.05 | 10007.56 | 10010.26 | NA |
CBA [31] | 10002.8596 | 10006.3251 | 10045.2265 | 9.5811 |
IWO | 9984.8409 | 9985.5127 | 9986.1947 | 0.3252 |
HIWO | 9981.7867 | 9982.0010 | 9982.1922 | 0.0934 |
To illustrate the convergence ability of HIWO for solving different-scale ED problems with various constraints, the convergence curves of HIWO and IWO on six test systems are drawn, as shown in Figure 2. It can be found from Figure 2 that HIWO can converge to the optimal areas in the six test systems, and the convergence speed of HIWO on the 15, 40, 80, 110 and 140-generator power systems, is faster than that of IWO. Although the convergence speed of HIWO on the 160-generator power system is slower than that of IWO in the early evolutionary stage, it is faster than that of IWO in the later evolutionary stage. The reason is that the crossover and mutation decrease the fitness value of offspring weeds in 160-generator power system having lots of constraints, and then reduce the convergence speed in the early evolutionary stage, but increase the diversity of the population to jump out local optimization in the later stage.
In this paper, a hybrid HIWO algorithm combining IWO with GA is proposed to solve ED problems in power systems. The HIWO adopts IWO to explore the various regions in the solution space, while the crossover and mutation operations of GA are applied to improve the quality and diversity of solutions, thereby preventing the optimization from prematurity and enhancing the search capability. Moreover, an effective repair method is proposed to repair infeasible solutions to feasible solutions. The experimental results of the six test systems studies show that HIWO can obtain the cheapest dispatch solutions compared to other algorithms in the literature, and have a better optimization ability and faster convergence speed compared to the classical IWO. In summary, the proposed HIWO algorithm is an effective and promising approach for solving ED problems in different-scale power systems.
This research is partially supported by the National Science Foundation of China under grant numbers 61773192 and 61773246, the Key Laboratory of Computer Network and Information Integration (Southeast University), the Ministry of Education (K93-9-2017-02), and the State Key Laboratory of Synthetical Automation for Process Industries (PAL-N201602).
The authors declare no conflict of interest.
[1] |
Uchechukwu-Agua AD, Caleb OJ, Opara UL (2015) Postharvest handling and storage of fresh cassava root and products: a review. Food Bioprocess Technol 8: 729-748. doi: 10.1007/s11947-015-1478-z
![]() |
[2] |
Burns AE, Gleadow RM, Zacarias AM, et al. (2012) Variations in the chemical composition of cassava (Manihot esculenta Crantz) leaves and roots as affected by genotypic and environmental variation. J Agric Food Chem 60: 4946-4956. doi: 10.1021/jf2047288
![]() |
[3] | Agiriga A, Iwe M (2016) Optimization of Chemical Properties of Cassava Varieties Harvested at Different Times using Response Surface Methodology. Am J Adv Food Sci Technol 4: 10-21. |
[4] |
Charles AL, Sriroth K, Huang T-c (2005) Proximate composition, mineral contents, hydrogen cyanide and phytic acid of 5 cassava genotypes. Food Chem 92: 615-620. doi: 10.1016/j.foodchem.2004.08.024
![]() |
[5] |
Chiwona-Karltun L, Nyirenda D, Mwansa CN, et al. (2015) Farmer preference, utilisation, and biochemical composition of improved cassava (Manihot esculenta Crantz) varieties in southeastern Africa. Econ Bot 69: 42-56. doi: 10.1007/s12231-015-9298-7
![]() |
[6] | Lu D, Lu W (2012) Effects of protein removal on the physicochemical properties of waxy maize flours. Starch‐Stärke 64: 874-881. |
[7] | Emmanuel O, Clement A, Agnes S, et al. (2012) Chemical composition and cyanogenic potential of traditional and high yielding CMD resistant cassava (Manihot esculenta Crantz) varieties. Int Food Res J 19: 175-181. |
[8] |
Mtunguja MK, Laswai HS, Kanju E, et al. (2016) Effect of genotype and genotype by environment interaction on total cyanide content, fresh root, and starch yield in farmer‐preferred cassava landraces in Tanzania. Food Sci Nutr 4: 791-801. doi: 10.1002/fsn3.345
![]() |
[9] | Kashala-Abotnes E, Okitundu D, Mumba D, et al. (2018) Konzo: a distinct neurological disease associated with food (cassava) cyanogenic poisoning. Brain Res Bull 145: 87-91. |
[10] |
Montagnac JA, Davis CR, Tanumihardjo SA (2009) Processing techniques to reduce toxicity and antinutrients of cassava for use as a staple food. Compr Rev Food Sci Food Saf 8: 17-27. doi: 10.1111/j.1541-4337.2008.00064.x
![]() |
[11] | Mlingi NL, Bainbridge Z (1994) Reduction of cyanogen levels during sun-drying of cassava in Tanzania. Acta Hortic 375: 233-240. |
[12] | Dixon AG, Asiedu R, Bokanga M (1994) Breeding of cassava for low cyanogenic potential: problems, progress and prospects. Acta Hortic 375: 153-162. |
[13] | Sarkiyayi S, Agar T (2010) Comparative analysis on the nutritional and anti-nutritional contents of the sweet and bitter cassava varieties. Adv J Food Sci Technol 2: 328-334. |
[14] | Barbosa-Cánovas GV, Harte F, Yan HH (2012) Particle size distribution in food powders. Food Eng 1: 303-328. |
[15] |
Roa DF, Baeza RI, Tolaba MP (2015) Effect of ball milling energy on rheological and thermal properties of amaranth flour. J Food Sci Technol 52: 8389-8394. doi: 10.1007/s13197-015-1976-z
![]() |
[16] | Scientific H (2012) A guidebook to particle size analysis. Horiba Instruments, Inc., 1-29. |
[17] | Numfor F, Walter W (1996) Cohesiveness of native cassava starch pastes: effect of fermentation. Afr J Root Tuber Crops 1: 29-32. |
[18] | Eriksson E, Koch K, Tortoe C, et al. (2014) Evaluation of the physical and sensory characteristics of bread produced from three varieties of cassava and wheat composite flours. Food Pub Health 4: 214-222. |
[19] |
Benesi IR, Labuschagne MT, Dixon AG, et al. (2004) Stability of native starch quality parameters, starch extraction and root dry matter of cassava genotypes in different environments. J Sci Food Agric 84: 1381-1388. doi: 10.1002/jsfa.1734
![]() |
[20] | Nuwamanya E, Baguma Y, Rubaihayo P (2010) Physicochemical and functional characteristics of cassava starch in Ugandan varieties and their progenies. J Plant Breed Crop Sci 2: 001-011. |
[21] | AOAC (2012) Official Methods of Analysis of AOAC international. Gaithersburg, Maryland, USA: 19th edition, AOAC International. |
[22] |
Orjiekwe C, Solola A, Iyen E, et al. (2013) Determination of cyanogenic glucosides in cassava products sold in Okada, Edo State, Nigeria. Afr J Food Sci 7: 468-472. doi: 10.5897/AJFS2013.1012
![]() |
[23] |
Zhu F, Sakulnak R, Wang S (2016) Effect of black tea on antioxidant, textural, and sensory properties of Chinese steamed bread. Food Chem 194: 1217-1223. doi: 10.1016/j.foodchem.2015.08.110
![]() |
[24] | Sonaye S, Baxi R (2012) Particle size measurement and analysis of flour. Int J Eng Res Appl 2: 1839-1842. |
[25] | Ahmed J, Thomas L, Arfat YA (2018) Functional, rheological, microstructural and antioxidant properties of quinoa flour in dispersions as influenced by particle size. Food Res Int 116: 302-311. |
[26] |
Oladunmoye OO, Aworh OC, Maziya‐Dixon B, et al. (2014) Chemical and functional properties of cassava starch, durum wheat semolina flour, and their blends. Food Sci Nutr 2: 132-138. doi: 10.1002/fsn3.83
![]() |
[27] |
Lazaridou A, Marinopoulou A, Biliaderis CG (2019) Impact of flour particle size and hydrothermal treatment on dough rheology and quality of barley rusks. Food Hydrocolloids 87: 561-569. doi: 10.1016/j.foodhyd.2018.08.045
![]() |
[28] |
Farooq AM, Li C, Chen S, et al. (2018) Particle size affects structural and in vitro digestion properties of cooked rice flours. Int J Biol Macromol 118: 160-167. doi: 10.1016/j.ijbiomac.2018.06.071
![]() |
[29] | Etuk BR, Akpan I (2003) Optimum size distribution of sorghum grist for brewing purposes. Global J Pure Appl Sci 9: 259-264. |
[30] |
Hossen MS, Sotome I, Takenaka M, et al. (2011) Effect of particle size of different crop starches and their flours on pasting properties. Jpn J Food Eng 12: 29-35. doi: 10.11301/jsfe.12.29
![]() |
[31] |
Oladunmoye O, Akinoso R, Olapade A (2010) Evaluation of some physical-chemical properties of wheat, cassava, maize and cowpea flours for bread making. J Food Qual 33: 693-708. doi: 10.1111/j.1745-4557.2010.00351.x
![]() |
[32] |
Eleazu OC, Eleazu KC, Kolawole S (2014) Use of indigenous technology for the production of high quality cassava flour with similar food qualities as wheat flour. Acta Sci Pol, Technol Aliment 13: 249-256. doi: 10.17306/J.AFS.2014.3.3
![]() |
[33] |
Raigar R, Mishra H (2015) Effect of moisture content and particle sizes on physical and thermal properties of roasted B engal gram flour. J Food Process Preserv 39: 1839-1844. doi: 10.1111/jfpp.12419
![]() |
[34] |
Sharma A, Jana AH, Chavan RS (2012) Functionality of milk powders and milk‐based powders for end use applications-a review. Compr Rev Food Sci Food Saf 11: 518-528. doi: 10.1111/j.1541-4337.2012.00199.x
![]() |
[35] | Iwe M, Onyeukwu U, Agiriga A, et al. (2016) Proximate, functional and pasting properties of FARO 44 rice, African yam bean and brown cowpea seeds composite flour. Cogent Food Agric 2: 1142409. |
[36] |
Abdullah EC, Geldart D (1999) The use of bulk density measurements as flowability indicators. Powder Technol 102: 151-165. doi: 10.1016/S0032-5910(98)00208-3
![]() |
[37] | Van Toan N (2018) Preparation and Improved Quality Production of Flour and the Made Biscuits from Purple Sweet Potato. J Food Nutr 4: 1-14. |
[38] | Teye E, Asare A, Amoah R, et al. (2011) Determination of the dry matter content of cassava (Manihot esculenta, Crantz) tubers using specific gravity method. ARPN J Agric Biol Sci 6: 23-28. |
[39] |
Beyene G, Solomon FR, Chauhan RD, et al. (2018) Provitamin A biofortification of cassava enhances shelf life but reduces dry matter content of storage roots due to altered carbon partitioning into starch. Plant Biotechnol J 16: 1186-1200. doi: 10.1111/pbi.12862
![]() |
[40] |
Buddhakulsomsiri J, Parthanadee P, Pannakkong W (2018) Prediction models of starch content in fresh cassava roots for a tapioca starch manufacturer in Thailand. Comput Electron Agric 154: 296-303. doi: 10.1016/j.compag.2018.09.016
![]() |
[41] | Abera S, Rakshit SK (2003) Processing technology comparison of physicochemical and functional properties of cassava starch extracted from fresh root and dry chips. Starch‐Stärke 55: 287-296. |
[42] |
Pérez JC, Lenis JI, Calle F, et al. (2011) Genetic variability of root peel thickness and its influence in extractable starch from cassava (Manihot esculenta Crantz) roots. Plant Breed 130: 688-693. doi: 10.1111/j.1439-0523.2011.01873.x
![]() |
[43] |
Manano J, Ogwok P, Byarugaba-Bazirake GW (2017) Chemical composition of major cassava varieties in Uganda, targeted for industrialisation. J Food Res 7: 1. doi: 10.5539/jfr.v7n1p1
![]() |
[44] |
Passos MEAd, Moreira CFF, Pacheco MTB, et al. (2013) Proximate and mineral composition of industrialized biscuits. Food Sci Technol 33: 323-331. doi: 10.1590/S0101-20612013005000046
![]() |
[45] | Charles AL, Chang YH, Ko WC, et al. (2004) Some physical and chemical properties of starch isolates of cassava genotypes. Starch‐Stärke 56: 413-418. |
[46] |
Montagnac JA, Davis CR, Tanumihardjo SA (2009) Nutritional value of cassava for use as a staple food and recent advances for improvement. Compr Rev Food Sci Food Saf 8: 181-194. doi: 10.1111/j.1541-4337.2009.00077.x
![]() |
[47] |
Somendrika M, Wickramasinghe I, Wansapala M, et al. (2016) Analysing proximate composition of macro nutrients of Sri Lankan cassava variety 'Kirikawadi'. Pak J Nutr 15: 283. doi: 10.3923/pjn.2016.283.287
![]() |
[48] | Shittu T, Dixon A, Awonorin S, et al. (2008) Bread from composite cassava-wheat flour. Ⅱ: Effect of cassava genotype and nitrogen fertilizer on bread quality. Food Res Int 41: 569-578. |
[49] |
Costantino HR, Curley JG, Wu S, et al. (1998) Water sorption behavior of lyophilized protein-sugar systems and implications for solid-state interactions. Int J Pharm 166: 211-221. doi: 10.1016/S0378-5173(98)00050-7
![]() |
[50] | Larsson K (1982) Some effects of lipids on the structure of foods. Food Struct 1: 6. |
[51] |
Nwokocha LM, Aviara NA, Senan C, et al. (2009) A comparative study of some properties of cassava (Manihot esculenta, Crantz) and cocoyam (Colocasia esculenta, Linn) starches. Carbohydr Polym 76: 362-367. doi: 10.1016/j.carbpol.2008.10.034
![]() |
[52] |
Peroni F, Rocha T, Franco C (2006) Some structural and physicochemical characteristics of tuber and root starches. Food Sci Technol Int 12: 505-513. doi: 10.1177/1082013206073045
![]() |
[53] | Rojas CC, Nair B, Herbas A, et al. (2007) Proximal composition and mineral contents of six varieties of cassava (Mannihot esculenta Crantz), from Bolivia. Rev Boliv Quim 24: 71-77. |
[54] |
Eleazu C, Eleazu K (2012) Determination of the proximate composition, total carotenoid, reducing sugars and residual cyanide levels of flours of 6 new yellow and white cassava (Manihot esculenta Crantz) varieties. Am J Food Technol 7: 642-649. doi: 10.3923/ajft.2012.642.649
![]() |
[55] |
Pavlovich‐Abril A, Rouzaud‐Sández O, Romero‐Baranzini AL, et al. (2015) Relationships between chemical composition and quality‐related characteristics in bread making with wheat flour-fine bran blends. J Food Qual 38: 30-39. doi: 10.1111/jfq.12103
![]() |
[56] |
Katyal M, Virdi AS, Kaur A, et al. (2016) Diversity in quality traits amongst Indian wheat varieties I: Flour and protein characteristics. Food Chem 194: 337-344. doi: 10.1016/j.foodchem.2015.07.125
![]() |
[57] |
Yu L, Nanguet AL (2013) Comparison of antioxidant properties of refined and whole wheat flour and bread. Antioxidants 2: 370-383. doi: 10.3390/antiox2040370
![]() |
[58] | Schwantes D, Gonçalves AC, Coelho GF, et al. (2016) Chemical modifications of cassava peel as adsorbent material for metals ions from wastewater. J Chem 2016: 3694174. |
[59] |
Grundy MML, Edwards CH, Mackie AR, et al. (2016) Re-evaluation of the mechanisms of dietary fibre and implications for macronutrient bioaccessibility, digestion and postprandial metabolism. Br J Nutr 116: 816-833. doi: 10.1017/S0007114516002610
![]() |
[60] | Brett CT, Waldron KW (1996) Physiology and biochemistry of plant cell walls. Vol. 2, Springer Science & Business Media. |
[61] |
Do DT, Singh J, Oey I, et al. (2018) Biomimetic plant foods: Structural design and functionality. Trends Food Sci Technol 82: 46-59. doi: 10.1016/j.tifs.2018.09.010
![]() |
[62] | Oluwaniyi OO, Oladipo JO (2017) Comparative studies on the phytochemicals, nutrients and antinutrients content of cassava varieties. J Turk Chem Soc 4: 661-674. |
[63] |
Del Carmen Fernández-Alonso M, Díaz D, Alvaro Berbis M, et al. (2012) Protein-carbohydrate interactions studied by NMR: from molecular recognition to drug design. Curr Protein Pept Sci 13: 816-830. doi: 10.2174/138920312804871175
![]() |
[64] |
Pomeranz Y, Chung O (1978) Interaction of lipids with proteins and carbohydrates in breadmaking. J Am Oil Chem Soc 55: 285-289. doi: 10.1007/BF02676944
![]() |
[65] | Li L, Wang N, Ma S, et al. (2018) Relationship of moisture status and quality characteristics of fresh wet noodles prepared from different grade wheat flours from flour milling streams. J Chem 2018: 1-8. |
[66] | Ndubuisi N, Chidiebere A (2018) Cyanide in cassava: a review. Int J Genomics Data Min 10: 1-10. |
[67] |
Figueiredo PG, de Moraes-Dallaqua MA, Bicudo SJ, et al. (2015) Development of tuberous cassava roots under different tillage systems: descriptive anatomy. Plant Prod Sci 18: 241-245. doi: 10.1626/pps.18.241
![]() |
[68] | Zidenga T, Siritunga D, Sayre RT (2017) Cyanogen metabolism in Cassava roots: Impact on protein synthesis and root development. Front Recent Dev Plant Sci 8: 1-12. |
[69] | Samson SO, Akomolafe O, FK O (2017) Fermentation: A means of treating and improving the nutrition content of cassava (manihot esculenta C.) peels and reducing its cyanide content. Genomics Appl Biol 8: 17-25. |
[70] |
Akande SA, Onyegbula AF, Salawu RA, et al. (2017) Effects of post-harvest handling on hydrogen cyanide content of cassava products obtained from Ilorin-West urban markets, Nigeria. Afr J Food Sci 11: 362-368. doi: 10.5897/AJFS2017.1537
![]() |
[71] | FAO/WHO (1991) Joint FAO/WHO food standards programme. Codex Alimentarius Commission, XⅡ (Suppl. 4), Rome: FAO. |
[72] | Omolola AO, Kapila PF, Anyasi TA, et al. (2017) Optimization of colour and thermal properties of sweet cassava (Manihot esculenta Crantz Var. UVLNR 0005) flour using response surface methodology. Asian J Agric Res 11: 57-65. |
[73] | Eriksson E, Koch K, Tortoe C, et al. (2014) Physicochemical, functional and pasting characteristics of three varieties of cassava in wheat composite flours. Br J Appl Sci Technol 4: 1069-1073. |
[74] | Hu W, Tie W, Ou W, et al. (2018) Crosstalk between calcium and melatonin affects postharvest physiological deterioration and quality loss in cassava. Postharvest Biol Technol 140: 42-49. |
[75] | Sankhon A, Amadou I, Yao WR, et al. (2014) Comparison of physicochemical and functional properties of flour and starch extract in different methods from africa locust bean (Parkia Biglobosa) Seeds. Afr J Tradit, Complementary Altern Med 11: 264-272. |
[76] | Vasconcelos L, Brito A, Carmo C, et al. (2017) Phenotypic diversity of starch granules in cassava germplasm. Genet Mol Res: GMR 16: 1-10. |
[77] |
McClements DJ, Chung C, Wu B (2017) Structural design approaches for creating fat droplet and starch granule mimetics. Food Funct 8: 498-510. doi: 10.1039/C6FO00764C
![]() |
[78] |
Rodriguez-Sandoval E, Prasca-Sierra I, Hernandez V (2017) Effect of modified cassava starch as a fat replacer on the texture and quality characteristics of muffins. J Food Meas Charact 11: 1630-1639. doi: 10.1007/s11694-017-9543-0
![]() |
[79] | Jyothi AN, Kiran KS, Wilson B, et al. (2007) Wet storage of cassava starch: Use of sodium metabisulphite and acetic acid and the effect on starch properties. Starch‐Stärke 59: 141-148. |
1. | Qi Wang, Yufeng Guo, Dongrui Zhang, Yingwei Wang, Ying Xu, Jilai Yu, Research on wind farm participating in AGC based on wind power variogram characteristics, 2022, 19, 1551-0018, 8288, 10.3934/mbe.2022386 | |
2. | Swati Jain, Dr Krishna Teeth Chaturvedi, Review on Economic Load Dispatch and Associated Artificial Intelligence Algorithms, 2021, 7, 2582-4600, 34, 10.24113/ijoscience.v7i3.370 | |
3. | Mahdi Azizi, Milad Baghalzadeh Shishehgarkhaneh, Mahla Basiri, Optimum design of truss structures by Material Generation Algorithm with discrete variables, 2022, 3, 27726622, 100043, 10.1016/j.dajour.2022.100043 | |
4. | Dinu Calin Secui, Monica Liana Secui, Social small group optimization algorithm for large-scale economic dispatch problem with valve-point effects and multi-fuel sources, 2024, 54, 0924-669X, 8296, 10.1007/s10489-024-05517-8 | |
5. | Rahul Gupta, Ashish Khanna, Bal Virdee, AOBL-IPACO: A novel and optimized algorithm to mitigate losses in electrical grid systems, 2024, 2511-2104, 10.1007/s41870-024-02211-3 | |
6. | Thammarsat Visutarrom, Tsung-Che Chiang, Economic dispatch using metaheuristics: Algorithms, problems, and solutions, 2024, 150, 15684946, 110891, 10.1016/j.asoc.2023.110891 | |
7. | Xiaobao Yu, Zhenyu Dong, Dandan Zheng, Siwei Deng, Analysis of critical peak electricity price optimization model considering coal consumption rate of power generation side, 2023, 31, 1614-7499, 41514, 10.1007/s11356-023-29754-5 | |
8. | Balasim M. Hussein, Ahmed I. Jaber, Mohammed W. Abdulwahhab, Hayder J. Mohammed, Nikolay V. Korovkin, 2024, Application of Intelligent Optimization Algorithms on Economic Dispatch Problem, 979-8-3503-6370-8, 453, 10.1109/SCM62608.2024.10554111 | |
9. | Muhammet Demirbas, Serhat Duman, Burcin Ozkaya, Yunus Balci, Deniz Ersoy, M. Kenan Döşoğlu, Ugur Guvenc, Bekir Emre Altun, Hasan Uzel, Enes Kaymaz, Fuzzy-Based Fitness–Distance Balance Snow Ablation Optimizer Algorithm for Optimal Generation Planning in Power Systems, 2025, 18, 1996-1073, 3048, 10.3390/en18123048 |
Generators | Pi | Generators | Pi | Generators | Pi | Generators | Pi | Generators | Pi |
1 | 455.0000 | 4 | 130.0000 | 7 | 430.0000 | 10 | 159.7871 | 13 | 25.0000 |
2 | 380.0000 | 5 | 170.0000 | 8 | 71.2594 | 11 | 80.0000 | 14 | 15.0000 |
3 | 130.0000 | 6 | 460.0000 | 9 | 58.4944 | 12 | 80.0000 | 15 | 15.0000 |
Algorithms | SCmin ($) | SCavg ($) | SCmax ($) | SCstd |
EMA [52] | 32704.4503 | 32704.4504 | 32704.4506 | NA |
FA [27] | 32704.5000 | 32856.1000 | 33175.0000 | 147.17022 |
ICS [50] | 32706.7358 | 32714.4669 | 32752.5183 | NA |
WCA [49] | 32704.4492 | 32704.5096 | 32704.5196 | 4.513e-05 |
RTO [51] | 32701.8145 | 32704.5300 | 32715.1800 | 5.07 |
OLCSO [2] | 32692.3961 | 32692.3981 | 32692.4033 | 0.0022 |
IWO | 32691.8615 | 32691.9392 | 32692.1421 | 0.0927 |
HIWO | 32691.5614 | 32691.8615 | 32691.8616 | 0.0001 |
Generators | Pi | Generators | Pi | Generators | Pi | Generators | Pi | Generators | Pi |
1 | 113.9993 | 9 | 289.4281 | 17 | 489.2798 | 25 | 523.2794 | 33 | 190.0000 |
2 | 113.9993 | 10 | 279.5996 | 18 | 489.2793 | 26 | 523.2794 | 34 | 200.0000 |
3 | 120.0000 | 11 | 243.5995 | 19 | 511.2795 | 27 | 10.0000 | 35 | 199.9999 |
4 | 179.7330 | 12 | 94.0000 | 20 | 511.2793 | 28 | 10.0000 | 36 | 164.7999 |
5 | 87.7999 | 13 | 484.0391 | 21 | 523.2794 | 29 | 10.0000 | 37 | 109.9998 |
6 | 139.9998 | 14 | 484.0390 | 22 | 523.2794 | 30 | 87.7999 | 38 | 110.0000 |
7 | 300.0000 | 15 | 484.0393 | 23 | 523.2794 | 31 | 190.0000 | 39 | 109.9999 |
8 | 299.9997 | 16 | 484.0391 | 24 | 523.2794 | 32 | 190.0000 | 40 | 549.9999 |
Algorithms | SCmin ($) | SCavg ($) | SCmax ($) | SCstd |
ORCCRO [28] | 136855.19 | 136855.19 | 136855.19 | NA |
BBO [28] | 137026.82 | 137116.58 | 137587.82 | NA |
DE/BBO [28] | 136950.77 | 136966.77 | 137150.77 | NA |
SDE [29] | 138157.46 | NA | NA | NA |
OIWO [32] | 136452.68 | 136452.68 | 136452.68 | NA |
HAAA [37] | 136433.5 | 136436.6 | NA | 3.341896 |
IWO | 136543.8580 | 137009.5641 | 137679.1073 | 292.9686 |
HIWO | 136430.9504 | 136435.2127 | 136441.1059 | 4.3238 |
Generators | Pi | Generators | Pi | Generators | Pi | Generators | Pi | Generators | Pi |
1 | 110.8335 | 17 | 489.3362 | 33 | 189.9994 | 49 | 284.6071 | 65 | 523.2794 |
2 | 111.5439 | 18 | 489.2794 | 34 | 165.1983 | 50 | 130.0000 | 66 | 523.2835 |
3 | 97.3834 | 19 | 511.2731 | 35 | 199.9997 | 51 | 94.0040 | 67 | 10.0000 |
4 | 179.7603 | 20 | 511.2666 | 36 | 199.9998 | 52 | 94.0000 | 68 | 10.0000 |
5 | 87.9806 | 21 | 523.2525 | 37 | 109.9999 | 53 | 214.7298 | 69 | 10.0000 |
6 | 139.9997 | 22 | 523.2805 | 38 | 110.0000 | 54 | 394.2675 | 70 | 87.8052 |
7 | 259.5584 | 23 | 523.2794 | 39 | 109.9987 | 55 | 394.2967 | 71 | 190.0000 |
8 | 284.7677 | 24 | 523.2794 | 40 | 511.2603 | 56 | 304.4839 | 72 | 189.9997 |
9 | 284.6331 | 25 | 523.2794 | 41 | 110.9296 | 57 | 489.3082 | 73 | 189.9991 |
10 | 130.0000 | 26 | 523.2958 | 42 | 110.8195 | 58 | 489.2773 | 74 | 164.7786 |
11 | 169.0220 | 27 | 10.0000 | 43 | 97.3706 | 59 | 511.2121 | 75 | 199.9994 |
12 | 94.0000 | 28 | 10.0000 | 44 | 179.7187 | 60 | 511.2992 | 76 | 200.0000 |
13 | 214.7422 | 29 | 10.0000 | 45 | 87.8560 | 61 | 523.2830 | 77 | 109.9990 |
14 | 394.1929 | 30 | 89.6856 | 46 | 139.9995 | 62 | 523.3201 | 78 | 110.0000 |
15 | 394.2794 | 31 | 189.9993 | 47 | 259.6320 | 63 | 523.2794 | 79 | 109.9996 |
16 | 394.3050 | 32 | 189.9992 | 48 | 284.6702 | 64 | 523.2794 | 80 | 511.2482 |
Algorithms | SCmin ($) | SCavg ($) | SCmax ($) | SCstd |
THS [34] | 243192.6899 | 243457.36 | NA | 120.9889 |
CSO [40] | 243195.3781 | 243546.6283 | 244038.7352 | NA |
HAAA [37] | 242815.9 | 242883 | 242944.5 | 29.2849 |
GWO [35] | 242825.4799 | 242829.8192 | 242837.1303 | 0.093 |
IWO | 246386.4038 | 248088.2077 | 249888.0623 | 844.0919 |
HIWO | 242815.2096 | 242836.1110 | 242872.4662 | 10.3458 |
Generators | Pi | Generators | Pi | Generators | Pi | Generators | Pi | Generators | Pi |
1 | 2.4000 | 23 | 68.9000 | 45 | 659.9999 | 67 | 70.0000 | 89 | 82.4977 |
2 | 2.4000 | 24 | 350.0000 | 46 | 616.2499 | 68 | 70.0000 | 90 | 89.2333 |
3 | 2.4000 | 25 | 400.0000 | 47 | 5.4000 | 69 | 70.0000 | 91 | 57.5687 |
4 | 2.4000 | 26 | 400.0000 | 48 | 5.4000 | 70 | 359.9999 | 92 | 99.9986 |
5 | 2.4000 | 27 | 499.9992 | 49 | 8.4000 | 71 | 399.9999 | 93 | 439.9998 |
6 | 4.0000 | 28 | 500.0000 | 50 | 8.4000 | 72 | 399.9998 | 94 | 499.9999 |
7 | 4.0000 | 29 | 199.9997 | 51 | 8.4000 | 73 | 105.2864 | 95 | 600.0000 |
8 | 4.0000 | 30 | 99.9998 | 52 | 12.0000 | 74 | 191.4091 | 96 | 471.5717 |
9 | 4.0000 | 31 | 10.0000 | 53 | 12.0000 | 75 | 89.9996 | 97 | 3.6000 |
10 | 64.5432 | 32 | 19.9993 | 54 | 12.0000 | 76 | 49.9999 | 98 | 3.6000 |
11 | 62.2465 | 33 | 79.9950 | 55 | 12.0000 | 77 | 160.0000 | 99 | 4.4000 |
12 | 36.2739 | 34 | 249.9998 | 56 | 25.2000 | 78 | 295.4962 | 100 | 4.4000 |
13 | 56.6406 | 35 | 359.9999 | 57 | 25.2000 | 79 | 175.0102 | 101 | 10.0000 |
14 | 25.0000 | 36 | 399.9997 | 58 | 35.0000 | 80 | 98.2829 | 102 | 10.0000 |
15 | 25.0000 | 37 | 39.9998 | 59 | 35.0000 | 81 | 10.0000 | 103 | 20.0000 |
16 | 25.0000 | 38 | 69.9996 | 60 | 45.0000 | 82 | 12.0000 | 104 | 20.0000 |
17 | 154.9999 | 39 | 99.9998 | 61 | 45.0000 | 83 | 20.0000 | 105 | 40.0000 |
18 | 154.9993 | 40 | 119.9984 | 62 | 45.0000 | 84 | 199.9999 | 106 | 40.0000 |
19 | 155.0000 | 41 | 157.4299 | 63 | 184.9996 | 85 | 324.9972 | 107 | 50.0000 |
20 | 155.0000 | 42 | 219.9999 | 64 | 184.9996 | 86 | 440.0000 | 108 | 30.0000 |
21 | 68.9000 | 43 | 439.9999 | 65 | 184.9984 | 87 | 14.0886 | 109 | 40.0000 |
22 | 68.9000 | 44 | 559.9998 | 66 | 184.9997 | 88 | 24.0910 | 110 | 20.0000 |
Algorithms | SCmin ($) | SCavg ($) | SCmax ($) | SCstd |
ORCCRO [28] | 198016.29 | 198016.32 | 198016.89 | NA |
BBO [28] | 198241.166 | 198413.45 | 199102.59 | NA |
DE/BBO [28] | 198231.06 | 198326.66 | 198828.57 | NA |
OIWO [32] | 197989.14 | 197989.41 | 197989.93 | NA |
OLCSO [2] | 197988.8576 | 197989.5832 | 197990.4551 | 0.3699 |
IWO | 198252.3594 | 198621.3233 | 198902.7697 | 138.4714 |
HIWO | 197988.1927 | 197988.1969 | 197988.2045 | 0.0025 |
Generators | Pi | Generators | Pi | Generators | Pi | Generators | Pi | Generators | Pi |
1 | 115.2442 | 29 | 500.9998 | 57 | 103.0000 | 85 | 115.0000 | 113 | 94.0000 |
2 | 189.0000 | 30 | 500.9994 | 58 | 198.0000 | 86 | 207.0000 | 114 | 94.0000 |
3 | 190.0000 | 31 | 505.9993 | 59 | 311.9941 | 87 | 207.0000 | 115 | 244.0000 |
4 | 190.0000 | 32 | 505.9997 | 60 | 281.1604 | 88 | 175.0000 | 116 | 244.0000 |
5 | 168.5393 | 33 | 506.0000 | 61 | 163.0000 | 89 | 175.0000 | 117 | 244.0000 |
6 | 189.9932 | 34 | 505.9998 | 62 | 95.0000 | 90 | 175.0000 | 118 | 95.0000 |
7 | 489.9992 | 35 | 499.9996 | 63 | 160.0000 | 91 | 175.0000 | 119 | 95.0000 |
8 | 489.9996 | 36 | 500.0000 | 64 | 160.0000 | 92 | 579.9998 | 120 | 116.0000 |
9 | 495.9997 | 37 | 240.9993 | 65 | 489.9465 | 93 | 645.0000 | 121 | 175.0000 |
10 | 495.9994 | 38 | 240.9999 | 66 | 196.0000 | 94 | 983.9998 | 122 | 2.0000 |
11 | 495.9997 | 39 | 773.9996 | 67 | 489.9717 | 95 | 977.9993 | 123 | 4.0000 |
12 | 496.0000 | 40 | 769.0000 | 68 | 489.9908 | 96 | 681.9997 | 124 | 15.0000 |
13 | 506.0000 | 41 | 3.0000 | 69 | 130.0000 | 97 | 719.9998 | 125 | 9.0000 |
14 | 509.0000 | 42 | 3.0000 | 70 | 234.7202 | 98 | 717.9993 | 126 | 12.0000 |
15 | 506.0000 | 43 | 249.2474 | 71 | 137.0000 | 99 | 719.9997 | 127 | 10.0000 |
16 | 504.9997 | 44 | 246.0287 | 72 | 325.4950 | 100 | 963.9998 | 128 | 112.0000 |
17 | 505.9997 | 45 | 249.9973 | 73 | 195.0000 | 101 | 958.0000 | 129 | 4.0000 |
18 | 505.9997 | 46 | 249.9863 | 74 | 175.0000 | 102 | 1006.9992 | 130 | 5.0000 |
19 | 504.9994 | 47 | 241.0622 | 75 | 175.0000 | 103 | 1006.0000 | 131 | 5.0000 |
20 | 505.0000 | 48 | 249.9950 | 76 | 175.0000 | 104 | 1012.9999 | 132 | 50.0000 |
21 | 504.9998 | 49 | 249.9916 | 77 | 175.0000 | 105 | 1019.9996 | 133 | 5.0000 |
22 | 505.0000 | 50 | 249.9995 | 78 | 330.0000 | 106 | 953.9999 | 134 | 42.0000 |
23 | 504.9998 | 51 | 165.0000 | 79 | 531.0000 | 107 | 951.9998 | 135 | 42.0000 |
24 | 504.9996 | 52 | 165.0000 | 80 | 530.9995 | 108 | 1005.9996 | 136 | 41.0000 |
25 | 536.9997 | 53 | 165.0000 | 81 | 398.6524 | 109 | 1013.0000 | 137 | 17.0000 |
26 | 536.9995 | 54 | 165.0000 | 82 | 56.0000 | 110 | 1020.9998 | 138 | 7.0000 |
27 | 548.9998 | 55 | 180.0000 | 83 | 115.0000 | 111 | 1014.9996 | 139 | 7.0000 |
28 | 548.9993 | 56 | 180.0000 | 84 | 115.0000 | 112 | 94.0000 | 140 | 26.0000 |
Algorithms | SCmin ($) | SCavg ($) | SCmax ($) | SCstd |
SDE [29] | 1560236.85 | NA | NA | NA |
OIWO [32] | 1559712.2604 | NA | NA | NA |
HAAA [37] | 1559710.00 | 1559712.87 | 1559731.00 | 4.1371 |
GWO [35] | 1559953.18 | 1560132.93 | 1560228.40 | 1.024 |
KGMO [41] | 1583944.60 | 1583952.14 | 1583963.52 | NA |
IWO | 1564050.0027 | 1567185.2227 | 1571056.6280 | 1678.8488 |
HIWO | 1559709.5266 | 1559709.6956 | 1559709.8959 | 0.0856 |
Generators | Pi | Generators | Pi | Generators | Pi | Generators | Pi | Generators | Pi |
1 | 218.6095 | 33 | 280.6560 | 65 | 279.6118 | 97 | 287.7203 | 129 | 431.0758 |
2 | 209.2361 | 34 | 238.9676 | 66 | 238.5645 | 98 | 238.6988 | 130 | 275.8790 |
3 | 279.6486 | 35 | 279.9554 | 67 | 287.7296 | 99 | 426.2750 | 131 | 219.6189 |
4 | 240.3113 | 36 | 240.9831 | 68 | 241.2519 | 100 | 272.6741 | 132 | 210.4739 |
5 | 280.0206 | 37 | 290.1069 | 69 | 427.7708 | 101 | 217.5647 | 133 | 281.6640 |
6 | 238.4301 | 38 | 240.0425 | 70 | 272.9907 | 102 | 211.9593 | 134 | 238.9676 |
7 | 288.2326 | 39 | 426.3102 | 71 | 218.5918 | 103 | 280.6578 | 135 | 276.5752 |
8 | 239.5051 | 40 | 275.6392 | 72 | 212.7020 | 104 | 239.2363 | 136 | 239.3707 |
9 | 425.6549 | 41 | 219.6195 | 73 | 281.6629 | 105 | 276.3263 | 137 | 287.7806 |
10 | 275.6903 | 42 | 210.9690 | 74 | 238.9676 | 106 | 240.7144 | 138 | 238.5645 |
11 | 217.5646 | 43 | 282.6711 | 75 | 279.3688 | 107 | 290.0715 | 139 | 430.7874 |
12 | 212.4544 | 44 | 240.3113 | 76 | 237.6239 | 108 | 238.8332 | 140 | 275.8606 |
13 | 280.6558 | 45 | 279.7868 | 77 | 289.9995 | 109 | 425.7918 | 141 | 218.6539 |
14 | 238.6988 | 46 | 237.4895 | 78 | 239.9082 | 110 | 275.2705 | 142 | 210.7215 |
15 | 279.9370 | 47 | 287.7274 | 79 | 425.2406 | 111 | 217.5671 | 143 | 281.6640 |
16 | 240.7144 | 48 | 240.0425 | 80 | 276.0112 | 112 | 212.2069 | 144 | 239.3707 |
17 | 287.6968 | 49 | 427.4497 | 81 | 218.5923 | 113 | 281.6664 | 145 | 276.3578 |
18 | 239.7738 | 50 | 275.6817 | 82 | 212.2069 | 114 | 239.6394 | 146 | 239.6394 |
19 | 427.4049 | 51 | 219.6197 | 83 | 282.7049 | 115 | 276.0940 | 147 | 287.7565 |
20 | 275.6990 | 52 | 213.4447 | 84 | 237.7582 | 116 | 240.3113 | 148 | 239.3707 |
21 | 217.5665 | 53 | 282.6717 | 85 | 279.7940 | 117 | 290.0972 | 149 | 426.3023 |
22 | 212.2069 | 54 | 237.8926 | 86 | 239.3707 | 118 | 239.5051 | 150 | 275.6371 |
23 | 283.6805 | 55 | 276.2856 | 87 | 290.0916 | 119 | 429.4367 | 151 | 217.5647 |
24 | 239.7738 | 56 | 239.5051 | 88 | 239.2363 | 120 | 275.6690 | 152 | 212.2069 |
25 | 279.9011 | 57 | 287.6883 | 89 | 427.0504 | 121 | 217.5656 | 153 | 279.6493 |
26 | 240.9831 | 58 | 238.5645 | 90 | 275.7937 | 122 | 210.2264 | 154 | 238.4301 |
27 | 290.0737 | 59 | 429.9489 | 91 | 217.5643 | 123 | 280.6617 | 155 | 279.9078 |
28 | 240.8488 | 60 | 275.5096 | 92 | 212.9496 | 124 | 239.7738 | 156 | 240.4457 |
29 | 427.1007 | 61 | 218.5915 | 93 | 282.6732 | 125 | 275.9409 | 157 | 287.7385 |
30 | 276.2995 | 62 | 212.9496 | 94 | 240.4457 | 126 | 240.1769 | 158 | 238.5645 |
31 | 219.6189 | 63 | 282.6705 | 95 | 279.4854 | 127 | 287.6965 | 159 | 426.9110 |
32 | 211.7117 | 64 | 239.9082 | 96 | 240.1769 | 128 | 238.4301 | 160 | 272.7775 |
Algorithms | SCmin ($) | SCavg ($) | SCmax ($) | SCstd |
ORCCRO [28] | 10004.20 | 10004.21 | 10004.45 | NA |
OIWO [32] | 9981.9834 | 9982.991 | 9983.998 | NA |
BBO [28] | 10008.71 | 10009.16 | 10010.59 | NA |
DE/BBO [28] | 10007.05 | 10007.56 | 10010.26 | NA |
CBA [31] | 10002.8596 | 10006.3251 | 10045.2265 | 9.5811 |
IWO | 9984.8409 | 9985.5127 | 9986.1947 | 0.3252 |
HIWO | 9981.7867 | 9982.0010 | 9982.1922 | 0.0934 |
Generators | Pi | Generators | Pi | Generators | Pi | Generators | Pi | Generators | Pi |
1 | 455.0000 | 4 | 130.0000 | 7 | 430.0000 | 10 | 159.7871 | 13 | 25.0000 |
2 | 380.0000 | 5 | 170.0000 | 8 | 71.2594 | 11 | 80.0000 | 14 | 15.0000 |
3 | 130.0000 | 6 | 460.0000 | 9 | 58.4944 | 12 | 80.0000 | 15 | 15.0000 |
Algorithms | SCmin ($) | SCavg ($) | SCmax ($) | SCstd |
EMA [52] | 32704.4503 | 32704.4504 | 32704.4506 | NA |
FA [27] | 32704.5000 | 32856.1000 | 33175.0000 | 147.17022 |
ICS [50] | 32706.7358 | 32714.4669 | 32752.5183 | NA |
WCA [49] | 32704.4492 | 32704.5096 | 32704.5196 | 4.513e-05 |
RTO [51] | 32701.8145 | 32704.5300 | 32715.1800 | 5.07 |
OLCSO [2] | 32692.3961 | 32692.3981 | 32692.4033 | 0.0022 |
IWO | 32691.8615 | 32691.9392 | 32692.1421 | 0.0927 |
HIWO | 32691.5614 | 32691.8615 | 32691.8616 | 0.0001 |
Generators | Pi | Generators | Pi | Generators | Pi | Generators | Pi | Generators | Pi |
1 | 113.9993 | 9 | 289.4281 | 17 | 489.2798 | 25 | 523.2794 | 33 | 190.0000 |
2 | 113.9993 | 10 | 279.5996 | 18 | 489.2793 | 26 | 523.2794 | 34 | 200.0000 |
3 | 120.0000 | 11 | 243.5995 | 19 | 511.2795 | 27 | 10.0000 | 35 | 199.9999 |
4 | 179.7330 | 12 | 94.0000 | 20 | 511.2793 | 28 | 10.0000 | 36 | 164.7999 |
5 | 87.7999 | 13 | 484.0391 | 21 | 523.2794 | 29 | 10.0000 | 37 | 109.9998 |
6 | 139.9998 | 14 | 484.0390 | 22 | 523.2794 | 30 | 87.7999 | 38 | 110.0000 |
7 | 300.0000 | 15 | 484.0393 | 23 | 523.2794 | 31 | 190.0000 | 39 | 109.9999 |
8 | 299.9997 | 16 | 484.0391 | 24 | 523.2794 | 32 | 190.0000 | 40 | 549.9999 |
Algorithms | SCmin ($) | SCavg ($) | SCmax ($) | SCstd |
ORCCRO [28] | 136855.19 | 136855.19 | 136855.19 | NA |
BBO [28] | 137026.82 | 137116.58 | 137587.82 | NA |
DE/BBO [28] | 136950.77 | 136966.77 | 137150.77 | NA |
SDE [29] | 138157.46 | NA | NA | NA |
OIWO [32] | 136452.68 | 136452.68 | 136452.68 | NA |
HAAA [37] | 136433.5 | 136436.6 | NA | 3.341896 |
IWO | 136543.8580 | 137009.5641 | 137679.1073 | 292.9686 |
HIWO | 136430.9504 | 136435.2127 | 136441.1059 | 4.3238 |
Generators | Pi | Generators | Pi | Generators | Pi | Generators | Pi | Generators | Pi |
1 | 110.8335 | 17 | 489.3362 | 33 | 189.9994 | 49 | 284.6071 | 65 | 523.2794 |
2 | 111.5439 | 18 | 489.2794 | 34 | 165.1983 | 50 | 130.0000 | 66 | 523.2835 |
3 | 97.3834 | 19 | 511.2731 | 35 | 199.9997 | 51 | 94.0040 | 67 | 10.0000 |
4 | 179.7603 | 20 | 511.2666 | 36 | 199.9998 | 52 | 94.0000 | 68 | 10.0000 |
5 | 87.9806 | 21 | 523.2525 | 37 | 109.9999 | 53 | 214.7298 | 69 | 10.0000 |
6 | 139.9997 | 22 | 523.2805 | 38 | 110.0000 | 54 | 394.2675 | 70 | 87.8052 |
7 | 259.5584 | 23 | 523.2794 | 39 | 109.9987 | 55 | 394.2967 | 71 | 190.0000 |
8 | 284.7677 | 24 | 523.2794 | 40 | 511.2603 | 56 | 304.4839 | 72 | 189.9997 |
9 | 284.6331 | 25 | 523.2794 | 41 | 110.9296 | 57 | 489.3082 | 73 | 189.9991 |
10 | 130.0000 | 26 | 523.2958 | 42 | 110.8195 | 58 | 489.2773 | 74 | 164.7786 |
11 | 169.0220 | 27 | 10.0000 | 43 | 97.3706 | 59 | 511.2121 | 75 | 199.9994 |
12 | 94.0000 | 28 | 10.0000 | 44 | 179.7187 | 60 | 511.2992 | 76 | 200.0000 |
13 | 214.7422 | 29 | 10.0000 | 45 | 87.8560 | 61 | 523.2830 | 77 | 109.9990 |
14 | 394.1929 | 30 | 89.6856 | 46 | 139.9995 | 62 | 523.3201 | 78 | 110.0000 |
15 | 394.2794 | 31 | 189.9993 | 47 | 259.6320 | 63 | 523.2794 | 79 | 109.9996 |
16 | 394.3050 | 32 | 189.9992 | 48 | 284.6702 | 64 | 523.2794 | 80 | 511.2482 |
Algorithms | SCmin ($) | SCavg ($) | SCmax ($) | SCstd |
THS [34] | 243192.6899 | 243457.36 | NA | 120.9889 |
CSO [40] | 243195.3781 | 243546.6283 | 244038.7352 | NA |
HAAA [37] | 242815.9 | 242883 | 242944.5 | 29.2849 |
GWO [35] | 242825.4799 | 242829.8192 | 242837.1303 | 0.093 |
IWO | 246386.4038 | 248088.2077 | 249888.0623 | 844.0919 |
HIWO | 242815.2096 | 242836.1110 | 242872.4662 | 10.3458 |
Generators | Pi | Generators | Pi | Generators | Pi | Generators | Pi | Generators | Pi |
1 | 2.4000 | 23 | 68.9000 | 45 | 659.9999 | 67 | 70.0000 | 89 | 82.4977 |
2 | 2.4000 | 24 | 350.0000 | 46 | 616.2499 | 68 | 70.0000 | 90 | 89.2333 |
3 | 2.4000 | 25 | 400.0000 | 47 | 5.4000 | 69 | 70.0000 | 91 | 57.5687 |
4 | 2.4000 | 26 | 400.0000 | 48 | 5.4000 | 70 | 359.9999 | 92 | 99.9986 |
5 | 2.4000 | 27 | 499.9992 | 49 | 8.4000 | 71 | 399.9999 | 93 | 439.9998 |
6 | 4.0000 | 28 | 500.0000 | 50 | 8.4000 | 72 | 399.9998 | 94 | 499.9999 |
7 | 4.0000 | 29 | 199.9997 | 51 | 8.4000 | 73 | 105.2864 | 95 | 600.0000 |
8 | 4.0000 | 30 | 99.9998 | 52 | 12.0000 | 74 | 191.4091 | 96 | 471.5717 |
9 | 4.0000 | 31 | 10.0000 | 53 | 12.0000 | 75 | 89.9996 | 97 | 3.6000 |
10 | 64.5432 | 32 | 19.9993 | 54 | 12.0000 | 76 | 49.9999 | 98 | 3.6000 |
11 | 62.2465 | 33 | 79.9950 | 55 | 12.0000 | 77 | 160.0000 | 99 | 4.4000 |
12 | 36.2739 | 34 | 249.9998 | 56 | 25.2000 | 78 | 295.4962 | 100 | 4.4000 |
13 | 56.6406 | 35 | 359.9999 | 57 | 25.2000 | 79 | 175.0102 | 101 | 10.0000 |
14 | 25.0000 | 36 | 399.9997 | 58 | 35.0000 | 80 | 98.2829 | 102 | 10.0000 |
15 | 25.0000 | 37 | 39.9998 | 59 | 35.0000 | 81 | 10.0000 | 103 | 20.0000 |
16 | 25.0000 | 38 | 69.9996 | 60 | 45.0000 | 82 | 12.0000 | 104 | 20.0000 |
17 | 154.9999 | 39 | 99.9998 | 61 | 45.0000 | 83 | 20.0000 | 105 | 40.0000 |
18 | 154.9993 | 40 | 119.9984 | 62 | 45.0000 | 84 | 199.9999 | 106 | 40.0000 |
19 | 155.0000 | 41 | 157.4299 | 63 | 184.9996 | 85 | 324.9972 | 107 | 50.0000 |
20 | 155.0000 | 42 | 219.9999 | 64 | 184.9996 | 86 | 440.0000 | 108 | 30.0000 |
21 | 68.9000 | 43 | 439.9999 | 65 | 184.9984 | 87 | 14.0886 | 109 | 40.0000 |
22 | 68.9000 | 44 | 559.9998 | 66 | 184.9997 | 88 | 24.0910 | 110 | 20.0000 |
Algorithms | SCmin ($) | SCavg ($) | SCmax ($) | SCstd |
ORCCRO [28] | 198016.29 | 198016.32 | 198016.89 | NA |
BBO [28] | 198241.166 | 198413.45 | 199102.59 | NA |
DE/BBO [28] | 198231.06 | 198326.66 | 198828.57 | NA |
OIWO [32] | 197989.14 | 197989.41 | 197989.93 | NA |
OLCSO [2] | 197988.8576 | 197989.5832 | 197990.4551 | 0.3699 |
IWO | 198252.3594 | 198621.3233 | 198902.7697 | 138.4714 |
HIWO | 197988.1927 | 197988.1969 | 197988.2045 | 0.0025 |
Generators | Pi | Generators | Pi | Generators | Pi | Generators | Pi | Generators | Pi |
1 | 115.2442 | 29 | 500.9998 | 57 | 103.0000 | 85 | 115.0000 | 113 | 94.0000 |
2 | 189.0000 | 30 | 500.9994 | 58 | 198.0000 | 86 | 207.0000 | 114 | 94.0000 |
3 | 190.0000 | 31 | 505.9993 | 59 | 311.9941 | 87 | 207.0000 | 115 | 244.0000 |
4 | 190.0000 | 32 | 505.9997 | 60 | 281.1604 | 88 | 175.0000 | 116 | 244.0000 |
5 | 168.5393 | 33 | 506.0000 | 61 | 163.0000 | 89 | 175.0000 | 117 | 244.0000 |
6 | 189.9932 | 34 | 505.9998 | 62 | 95.0000 | 90 | 175.0000 | 118 | 95.0000 |
7 | 489.9992 | 35 | 499.9996 | 63 | 160.0000 | 91 | 175.0000 | 119 | 95.0000 |
8 | 489.9996 | 36 | 500.0000 | 64 | 160.0000 | 92 | 579.9998 | 120 | 116.0000 |
9 | 495.9997 | 37 | 240.9993 | 65 | 489.9465 | 93 | 645.0000 | 121 | 175.0000 |
10 | 495.9994 | 38 | 240.9999 | 66 | 196.0000 | 94 | 983.9998 | 122 | 2.0000 |
11 | 495.9997 | 39 | 773.9996 | 67 | 489.9717 | 95 | 977.9993 | 123 | 4.0000 |
12 | 496.0000 | 40 | 769.0000 | 68 | 489.9908 | 96 | 681.9997 | 124 | 15.0000 |
13 | 506.0000 | 41 | 3.0000 | 69 | 130.0000 | 97 | 719.9998 | 125 | 9.0000 |
14 | 509.0000 | 42 | 3.0000 | 70 | 234.7202 | 98 | 717.9993 | 126 | 12.0000 |
15 | 506.0000 | 43 | 249.2474 | 71 | 137.0000 | 99 | 719.9997 | 127 | 10.0000 |
16 | 504.9997 | 44 | 246.0287 | 72 | 325.4950 | 100 | 963.9998 | 128 | 112.0000 |
17 | 505.9997 | 45 | 249.9973 | 73 | 195.0000 | 101 | 958.0000 | 129 | 4.0000 |
18 | 505.9997 | 46 | 249.9863 | 74 | 175.0000 | 102 | 1006.9992 | 130 | 5.0000 |
19 | 504.9994 | 47 | 241.0622 | 75 | 175.0000 | 103 | 1006.0000 | 131 | 5.0000 |
20 | 505.0000 | 48 | 249.9950 | 76 | 175.0000 | 104 | 1012.9999 | 132 | 50.0000 |
21 | 504.9998 | 49 | 249.9916 | 77 | 175.0000 | 105 | 1019.9996 | 133 | 5.0000 |
22 | 505.0000 | 50 | 249.9995 | 78 | 330.0000 | 106 | 953.9999 | 134 | 42.0000 |
23 | 504.9998 | 51 | 165.0000 | 79 | 531.0000 | 107 | 951.9998 | 135 | 42.0000 |
24 | 504.9996 | 52 | 165.0000 | 80 | 530.9995 | 108 | 1005.9996 | 136 | 41.0000 |
25 | 536.9997 | 53 | 165.0000 | 81 | 398.6524 | 109 | 1013.0000 | 137 | 17.0000 |
26 | 536.9995 | 54 | 165.0000 | 82 | 56.0000 | 110 | 1020.9998 | 138 | 7.0000 |
27 | 548.9998 | 55 | 180.0000 | 83 | 115.0000 | 111 | 1014.9996 | 139 | 7.0000 |
28 | 548.9993 | 56 | 180.0000 | 84 | 115.0000 | 112 | 94.0000 | 140 | 26.0000 |
Algorithms | SCmin ($) | SCavg ($) | SCmax ($) | SCstd |
SDE [29] | 1560236.85 | NA | NA | NA |
OIWO [32] | 1559712.2604 | NA | NA | NA |
HAAA [37] | 1559710.00 | 1559712.87 | 1559731.00 | 4.1371 |
GWO [35] | 1559953.18 | 1560132.93 | 1560228.40 | 1.024 |
KGMO [41] | 1583944.60 | 1583952.14 | 1583963.52 | NA |
IWO | 1564050.0027 | 1567185.2227 | 1571056.6280 | 1678.8488 |
HIWO | 1559709.5266 | 1559709.6956 | 1559709.8959 | 0.0856 |
Generators | Pi | Generators | Pi | Generators | Pi | Generators | Pi | Generators | Pi |
1 | 218.6095 | 33 | 280.6560 | 65 | 279.6118 | 97 | 287.7203 | 129 | 431.0758 |
2 | 209.2361 | 34 | 238.9676 | 66 | 238.5645 | 98 | 238.6988 | 130 | 275.8790 |
3 | 279.6486 | 35 | 279.9554 | 67 | 287.7296 | 99 | 426.2750 | 131 | 219.6189 |
4 | 240.3113 | 36 | 240.9831 | 68 | 241.2519 | 100 | 272.6741 | 132 | 210.4739 |
5 | 280.0206 | 37 | 290.1069 | 69 | 427.7708 | 101 | 217.5647 | 133 | 281.6640 |
6 | 238.4301 | 38 | 240.0425 | 70 | 272.9907 | 102 | 211.9593 | 134 | 238.9676 |
7 | 288.2326 | 39 | 426.3102 | 71 | 218.5918 | 103 | 280.6578 | 135 | 276.5752 |
8 | 239.5051 | 40 | 275.6392 | 72 | 212.7020 | 104 | 239.2363 | 136 | 239.3707 |
9 | 425.6549 | 41 | 219.6195 | 73 | 281.6629 | 105 | 276.3263 | 137 | 287.7806 |
10 | 275.6903 | 42 | 210.9690 | 74 | 238.9676 | 106 | 240.7144 | 138 | 238.5645 |
11 | 217.5646 | 43 | 282.6711 | 75 | 279.3688 | 107 | 290.0715 | 139 | 430.7874 |
12 | 212.4544 | 44 | 240.3113 | 76 | 237.6239 | 108 | 238.8332 | 140 | 275.8606 |
13 | 280.6558 | 45 | 279.7868 | 77 | 289.9995 | 109 | 425.7918 | 141 | 218.6539 |
14 | 238.6988 | 46 | 237.4895 | 78 | 239.9082 | 110 | 275.2705 | 142 | 210.7215 |
15 | 279.9370 | 47 | 287.7274 | 79 | 425.2406 | 111 | 217.5671 | 143 | 281.6640 |
16 | 240.7144 | 48 | 240.0425 | 80 | 276.0112 | 112 | 212.2069 | 144 | 239.3707 |
17 | 287.6968 | 49 | 427.4497 | 81 | 218.5923 | 113 | 281.6664 | 145 | 276.3578 |
18 | 239.7738 | 50 | 275.6817 | 82 | 212.2069 | 114 | 239.6394 | 146 | 239.6394 |
19 | 427.4049 | 51 | 219.6197 | 83 | 282.7049 | 115 | 276.0940 | 147 | 287.7565 |
20 | 275.6990 | 52 | 213.4447 | 84 | 237.7582 | 116 | 240.3113 | 148 | 239.3707 |
21 | 217.5665 | 53 | 282.6717 | 85 | 279.7940 | 117 | 290.0972 | 149 | 426.3023 |
22 | 212.2069 | 54 | 237.8926 | 86 | 239.3707 | 118 | 239.5051 | 150 | 275.6371 |
23 | 283.6805 | 55 | 276.2856 | 87 | 290.0916 | 119 | 429.4367 | 151 | 217.5647 |
24 | 239.7738 | 56 | 239.5051 | 88 | 239.2363 | 120 | 275.6690 | 152 | 212.2069 |
25 | 279.9011 | 57 | 287.6883 | 89 | 427.0504 | 121 | 217.5656 | 153 | 279.6493 |
26 | 240.9831 | 58 | 238.5645 | 90 | 275.7937 | 122 | 210.2264 | 154 | 238.4301 |
27 | 290.0737 | 59 | 429.9489 | 91 | 217.5643 | 123 | 280.6617 | 155 | 279.9078 |
28 | 240.8488 | 60 | 275.5096 | 92 | 212.9496 | 124 | 239.7738 | 156 | 240.4457 |
29 | 427.1007 | 61 | 218.5915 | 93 | 282.6732 | 125 | 275.9409 | 157 | 287.7385 |
30 | 276.2995 | 62 | 212.9496 | 94 | 240.4457 | 126 | 240.1769 | 158 | 238.5645 |
31 | 219.6189 | 63 | 282.6705 | 95 | 279.4854 | 127 | 287.6965 | 159 | 426.9110 |
32 | 211.7117 | 64 | 239.9082 | 96 | 240.1769 | 128 | 238.4301 | 160 | 272.7775 |
Algorithms | SCmin ($) | SCavg ($) | SCmax ($) | SCstd |
ORCCRO [28] | 10004.20 | 10004.21 | 10004.45 | NA |
OIWO [32] | 9981.9834 | 9982.991 | 9983.998 | NA |
BBO [28] | 10008.71 | 10009.16 | 10010.59 | NA |
DE/BBO [28] | 10007.05 | 10007.56 | 10010.26 | NA |
CBA [31] | 10002.8596 | 10006.3251 | 10045.2265 | 9.5811 |
IWO | 9984.8409 | 9985.5127 | 9986.1947 | 0.3252 |
HIWO | 9981.7867 | 9982.0010 | 9982.1922 | 0.0934 |