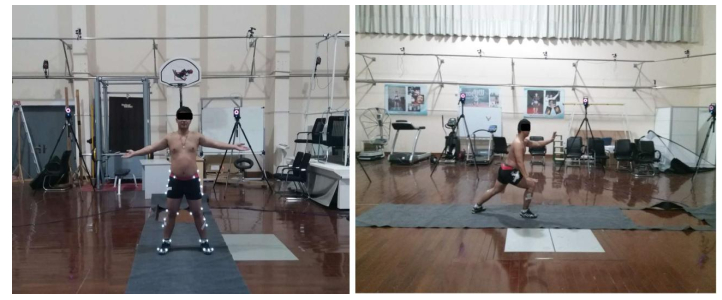
Citation: Yan Li, Kuan Wang, Lejun Wang, Tongbo Chang, Shengnian Zhang, Wenxin Niu. Biomechanical analysis of the meniscus and cartilage of the knee during a typical Tai Chi movement—brush-knee and twist-step[J]. Mathematical Biosciences and Engineering, 2019, 16(2): 898-908. doi: 10.3934/mbe.2019042
[1] | Lin Chen, Mingjun Wang, Zhanyu Wu, Jinbo Sun, Jianglong Li, Chun Chen, Chuan Ye . Advancements in health informatics: finite element insights into medial open-wedge high tibial osteotomy and lateral meniscal tears. Mathematical Biosciences and Engineering, 2024, 21(4): 5394-5410. doi: 10.3934/mbe.2024237 |
[2] | Teng Li, Xiang Chen, Shuai Cao, Xu Zhang, Xun Chen . Human hands-and-knees crawling movement analysis based on time-varying synergy and synchronous synergy theories. Mathematical Biosciences and Engineering, 2019, 16(4): 2492-2513. doi: 10.3934/mbe.2019125 |
[3] | Xuecheng Weng, Chang Mei, Farong Gao, Xudong Wu, Qizhong Zhang, Guangyu Liu . A gait stability evaluation method based on wearable acceleration sensors. Mathematical Biosciences and Engineering, 2023, 20(11): 20002-20024. doi: 10.3934/mbe.2023886 |
[4] | Viney Lohchab, Jaspreet Singh, Prasant Mahapatra, Vikas Bachhal, Aman Hooda, Karan Jindal, MS Dhillon . Thermal imaging in total knee replacement and its relation with inflammation markers. Mathematical Biosciences and Engineering, 2021, 18(6): 7759-7773. doi: 10.3934/mbe.2021385 |
[5] | Letícia Santos Cardoso, Luana Rocha Paulo, Cecília Ferreira Aquino, Isadora Gomes Alves Mariano, Gabriel Campos Souza, Gabriel Mader de Oliveira, Redha Taiar . Acute effects of foam rolling on ankle dorsiflexion and squat exercise patterns in extreme conditioning program practitioners: A randomized clinical trial. Mathematical Biosciences and Engineering, 2023, 20(10): 18761-18773. doi: 10.3934/mbe.2023831 |
[6] | Lingfei Mo, Lujie Zeng . Running gait pattern recognition based on cross-correlation analysis of single acceleration sensor. Mathematical Biosciences and Engineering, 2019, 16(6): 6242-6256. doi: 10.3934/mbe.2019311 |
[7] | Chengliang Wu, Weiya Hao, Wei He, Xiaofei Xiao, Xuhong Li, Wei Sun . Biomechanical and neuromuscular strategies on backward somersault landing in artistic gymnastics: A case study. Mathematical Biosciences and Engineering, 2019, 16(5): 5862-5876. doi: 10.3934/mbe.2019293 |
[8] | Xiuhan Li, Rui Feng, Funan Xiao, Yue Yin, Da Cao, Xiaoling Wu, Songsheng Zhu, Wei Wang . Sparse reconstruction of magnetic resonance image combined with two-step iteration and adaptive shrinkage factor. Mathematical Biosciences and Engineering, 2022, 19(12): 13214-13226. doi: 10.3934/mbe.2022618 |
[9] | Xiaobing Feng, Tingao Jiang . Mathematical and numerical analysis for PDE systems modeling intravascular drug release from arterial stents and transport in arterial tissue. Mathematical Biosciences and Engineering, 2024, 21(4): 5634-5657. doi: 10.3934/mbe.2024248 |
[10] | Huihao Wang, Kuan Wang, Zhen Deng, Xiaofei Li, Yi-Xian Qin, Hongsheng Zhan, Wenxin Niu . Effects of facet joint degeneration on stress alterations in cervical spine C5–C6: A finite element analysis. Mathematical Biosciences and Engineering, 2019, 16(6): 7447-7457. doi: 10.3934/mbe.2019373 |
Osteoarthritis (OA) is one of the most common causes of disability in the elderly, and brings a huge a burden to patients, family and even the wider community [1,2]. There is evidence for short-term improvements in pain, stiffness and physical function in patients with knee OA who practice Tai Chi (TC) on a regular basis [3,4]. It has also been indicated that TC can improve the gait performance of elderly OA patients [5]. Due to these findings, the American College of Rheumatology has recommended TC as a treatment option for patients with knee OA [6].
While the benefits of TC are known, the specific mechanism behind its action on the knee isn't clearly understood. The smooth and wide-ranging motions may allow the knee to profit from the biomechanical features of special TC movement patterns, as the loading and stress on the knee is closely linked with the development and progression of OA [7,8]. Previous studies in this area aimed to understand the kinematic and kinetic characteristics of TC movements. Mao et al. [9] found that TC movements, compared with normal walking, had a greater number of stages with 2-leg support and less with 1-leg support, and each support stage had a longer duration. Law and Li [10] recorded knee motion during two typical TC movements, Repulse Monkey and Wave-hand in Cloud. During both movements, the knees remained flexed and had greater abduction and adduction angle than during normal walking.
However, it is still unclear how TC acts to alleviate knee OA and how the movements affect the different structures of the knee joint. Although cartilage and meniscus play an important role in absorbing the shock between femur and tibia, and their degeneration has high relationship with knee OA [11], it is still poorly understood how these two structures respond to the loading conditions while practicing TC. Various finite element (FE) models have been constructed for biomechanical analysis [12,13]. Compared with in-vitro test and in-vivo sensors, the advantage of FE analysis is its non-invasiveness and is a powerfulness tool to in studying the internal stress/strain of the tissues under certain loading.
Therefore, the purpose of this study was to analyze the biomechanical response of the knee cartilage and meniscus to a typical TC movement, brush-knee and twist-step (BKTS) using FE analysis. BKTS involves basic TC movement of the lower extremity, in which the foot needs to be draw back and forth cyclically in coordination to the torso movement [14]. As reference, the results obtained from walking and jogging were also analyzed for comparison. It was hypothesized that the peak ground reaction force (GRF) and the contact stress on the knee joint of BKTS would be different to both walking or jogging because of the therapeutic effects reported from randomized controlled trials [15,16].
An experienced male TC practitioner (age: 26 years; body mass: 80 kg; height: 1.76 m) was recruited. An ethical approval was given by the Medicine and Life Sciences Ethics Committee of Tongji University (No. 2016yxy21). The subject had over ten years' experience in practicing TC and declared no knee injuries in the past 3 years. As shown in Figure 1, the BKTS movement was recorded at 100 Hz using a VICON Motion Analysis System (Vicon MX, Oxford Metrics, UK) with eight cameras. Two force plates (9287B, Kistler, Corporation, Switzerland) were used to record the ground reaction force (GRF) at 1000 Hz. The BKTS, jogging and walking were performed three times respectively, and the GRF data was averaged in terms of percentage of one stance phase for each movement. The results were then processed using Visual 3D software (C-Motion Inc., USA). Low-pass filtering at 10 Hz was used for kinematic data and 100 Hz was used for kinetic data [17,18].
The subject's knee joint was scanned using magnetic resonance imaging (MRI) in an upright position and when flexed. The MRI confirmed that the subject had no signs of knee joint disease. The images were saved in DICOM format and then imported into Mimics 15.0 (Materialise Inc., Leuven, Belgium). The femur, patella, tibia, fibula and soft tissue waswere segmented using a 2D mask in order to obtain three-dimensional point cloud images which could be further exported as STL files into the Geomagic software (Geomagic, Inc., Research Triangle Park, NC, USA) for further smoothing. The data was then converted into non-uniform rational B-spline surfaces.
Finally, ABAQUS (Simulia, Providence, USA) was used to mesh the femur, patella, tibia and fibula models with hybrid tetrahedral and hexahedral elements. Both the meniscus and cartilage were generated as hexahedral elements. The distal femur, proximal tibia and fibula, and meniscus were all assumed as isotropic and linearly elastic. The main ligaments of the knee joint (anterior cruciate ligament, ACL; posterior cruciate ligament, PCL; lateral collateral ligament, LCL; and medial collateral ligament, MCL) were all modeled as incompressible truss elements. Therefore, the incompressible truss elements could represent the feature that ligaments produced no force when they were compressed. The material properties of all components were sourced from literature [13,19,20,21,22] and are detailed in Table 1. To simulate the interaction between different parts of the knee, frictionless surface to surface contacts based on hard contact constraints were used.
Component | Element Type | Young's modulus (MPa) | Poisson's ratio |
Bone | Tetrahedral, hexahedral | 11000 | 0.3 |
Cartilage | Hexahedral | 5 | 0.35 |
Meniscus | Hexahedral | 59 | 0.45 |
Ligaments | Truss | 215 | 0.40 |
To determine an optimum mesh size for the FE model, contact areas distributions and contact pressures stress of the meniscus in the FE model were recorded under a 700 N axial load [23]. Three meshes were constructed on all model components with elements ranging from 0.5 mm to 1.5 mm in length through h-refinement method, and 1.0 mm was chosen to be the optimal mesh size. Convergence was considered to be reached if the relative change (contact areas and peak contact stress on the tibiofemoral joint in the validation step) between two adjacent meshes was less than 10%.
Fukubayashi and Kurosawa [24] recorded the contact area and stress pressure distribution on the tibiofemoral joint under various loads. This current study adopted these same boundary conditions to the FE model for model validation. The base of the tibia and fibula were constrained in six degrees of freedom, and a vertical load of 200,500 and 1000 N was sequentially applied to the proximal femur while the knee joint was held in a fully extended position. The contact areas on the tibial platform were recorded and compared with the experimental measurements by Fukubayashi and Kurosawa [24].
We simulated the condition when the peak GRF was produced in each of the three movements. To achieve this, the angular displacements of the knee joint at the moment of peak GRF were extracted and incorporated to the knee FE model through a reference point (RP). As shown in Figure 2, the lower part of the tibia and fibula were constrained in six degrees of freedom. Then, for the simulation of each movement, a vertical force equal to the peak GRF was applied as a concentrated force on the RP. It was assumed that the meniscus and cartilage sustained the greatest loading when subjected to the maximum GRF.
As shown in Figure 3, under vertical loads of 200,500 and 1000 N, the contact areas of the medial and lateral tibial cartilage in the FE model were found within the range of one standard deviation, and close to the mean values reported by Fukubayashi and Kurosawa [24].
The coordinate system was established with the subject in an upright standing posture. Extension, adduction and internal rotation of the knee joint were considered as positive motions in each anatomical plane. Compared with walking and jogging, the knee joint showed a greater ROM during the BKTS movement and the subject's pace was also slower. The overall ROM of the right knee joint during each movement is shown in Table 2.
Movements | Extension/Flexion (°) | Adduction/Abduction (°) | Internal/External rotation (°) |
Walking | −48~−8 | −6~2 | −9~8 |
Jogging | −32~−6 | −9~2 | −8~7 |
BKTS | −92~−1 | −16~12 | −15~14 |
Note: BKTS, Brush-knee and Twist-step. Negative angles indicate flexion/abduction/external rotation, while positive angles indicate extension/adduction/internal rotation. |
Walking and jogging were found to have a greater peak GRF than BKTS (Figure 4); peak GRF during walking was 121% body weight (BW), jogging was 187% BW and BKTS was 98% BW. From these results it is clearly evident that the GRF never exceeded 100% BW during the BKTS movement. Detailed angular displacements of the right knee at the point of peak GRF are shown in Table 3.
Movements | Extension/Flexion (°) | Adduction/Abduction (°) | Internal/External rotation (°) | Vertical load (% BW) |
Walking | −10.5 | −1.7 | −3.6 | 121 |
Jogging | −26 | −7.5 | 2.7 | 187 |
BKTS | −82 | −9.8 | 6.6 | 98 |
Note: BKTS, Brush-knee and Twist-step; BW, body weight. Negative angles indicate flexion/abduction/external rotation, while positive angles indicate extension/adduction/internal rotation. |
The angular displacements (Table 3) and peak vertical force (Figure 3) of each movement were incorporated into the finite element model. The peak contact stress pressure on the femoral cartilage was recorded as 4.2 MPa (walking), 6.4 MPa (jogging) and 3.2 MPa (BKTS). The peak contact stress on the meniscus was 4.2 MPa (walking), 6.5 MPa (jogging) and 3.2 MPa (BKTS). And the peak contact stress on the tibial cartilage was 3.2 MPa (walking), 4.7 MPa (jogging) and 2.4 MPa (BKTS).
Figure 5 shows the distribution of contact stress pressure on the knee joint during walking, jogging and BKTS. It can be seen that the stress pressure is much more concentrated during walking and jogging than BKTS, which is consistent with the lower peak contact stresses recorded on the cartilage and meniscus.
The purpose of this study was to explore the biomechanical effects of TC on the knee joint. In order to quantify the mechanical response, a typical TC movement, BKTS, was chosen and compared to typical daily movements (walking and jogging) in terms of kinematic and kinetic performance. The results showed that BKTS produced a greater ROM and lower stress on the knee joint, which is consistent with previous studies [9,25].
This study demonstrated how the BKTS movement produced a more uniformly distributed contact stress on the meniscus and cartilage than either walking or jogging in the healthy knee joint. The lower load and greater angular displacement were attributed to this. The peak vertical load on the knee joint during the BKTS movement was 98% BW, which was approximately half of the load experienced during jogging (187%); the peak load was 121% BW during walking. It was showed that the subject's movements tended to slow down once their foot touched the floor while performing the BKTS movement. This is a characteristic of TC movement. Such moving pattern of well-controlled coordination is required because TC movement should be performed slowly and smoothly with very small acceleration of center of mass [26]. At that moment, the subject would also shift the center of mass towards the other leg, thus balance the GRF on both feet. We simulated the condition of the knee joint when the peak GRF was produced during the BKTS movement, and the knee was flexed to 82° at that moment.
This FE analysis demonstrated that the position of the knee joint in a more extended position lead to a greater peak stress. Compared to walking and jogging, BKTS had a lower peak contact stress pressure on the femoral cartilage, meniscus and tibial cartilage at the point of peak vertical GRF. The gait analysis of BKTS also revealed that when the foot was close to the ground, the subject tended to slow their movements and reduce the contact speed, thereby reducing the peak stress pressure. In addition, when the movement was in the double-limb support phase, if the GRF of one foot was large, the subject's would partially shift their center of mass towards to the other leg, thus effectively reducing the effect of the reaction force on the meniscus and cartilage. This action of frequently shifting the center of mass is characteristic of TC movements. This result suggests that it may have a low risk of injury when performing TC.
Moderate contact stress pressure improves the generation of collagen and proteins in the cartilage polysaccharides and extracellular matrix, and increases modulus of the cartilage [27]. On the other hand, prolonged overloading leads to cartilage degradation [28]. The meniscus has the capability to transfer and redistribute loads from the femur onto the tibial plateau. This mechanism of load transfer and redistribution may be impaired under repetitive overloading, leading to stress concentrations and eventual injury to the knee joint [29]. Additional long-term effects including joint motion abnormalities and obesity would likely lead to further degradation of articular cartilage [28]. Therefore, the more uniform stress distribution and lower contact forces observed with the BKTS movement is a likely reason why TC is often recommended as a rehabilitation exercise for people suffering from osteoarthritis. However, whether the mechanical stimulation by this loading pattern may contribute to the improvement of the knee osteoarthritis observed in the clinical trial should be verified in future studies.
There are some limitations to this study that should be noted. Only one male subject and one TC movement was selected for testing. Wu et al. [30] reported the intra- and inter-subject variations of knee motion were 9° and 14° in TC gait, respectively. Therefore, a larger cohort of subjects including both male and female with different joint conditions examined through a series of TC movements would provide a more comprehensive biomechanical evaluation of the beneficial effects of the BKTS movement TC. The current study included only BKTS movement for the FE analysis because BKTS involves basic TC movement of lower extremity, which is cyclically performed in TC [14]. To further understand the biomechanics of whole TC movement on the knee joint, a series of TC movements should be investigated in the future studies. Also, a microscopic analysis of knee structures after undergoing simulated TC movements may also be useful to further explore the influence of TC movements on the knee joint. The present study is based on static analysis, but a dynamic study should be considered in future studies. Ultrasound provides high-quality images as well as timely spatial information of the scanned area [31]. The present study is based on static analysis. Future studies could investigate the dynamics of knee joint during TC movement through 3-D ultrasound scanning [32,33].
The simulated BKTS movement produced a more uniform stress pattern than walking and jogging on the meniscus and cartilage of the knee joint. Practicing TC may have a lower risk of knee joint injury compared to walking and jogging. Whether the mechanical stimulation by this loading pattern may contribute to the improvement of the knee osteoarthritis observed in the clinical trial should be verified in future studies.
This work was supported by the National Natural Science Foundation of China (No. 11672211/11732015) and the Fundamental Research Funds for the Central Universities.
All authors declare no conflicts of interest in this paper.
[1] | J. W. Bijlsma, F. Berenbaum and F. P. Lafeber, Osteoarthritis: An update with relevance for clinical practice, Lancet, 377 (2011), 2115–2126. |
[2] | G. Peat, R. McCarney and P. Croft, Knee pain and osteoarthritis in older adults: A review of community burden and current use of primary health care, Ann. Rheum. Dis., 60 (2011), 91–97. |
[3] | W. D. Chang, S. Chen, C. L. Lee, H. Y. Lin and P. T. Lai, The effects of tai chi chuan on improving mind-body health for knee osteoarthritis patients: A systematic review and meta-analysis, Evid. Based. Complement. Alternat. Med., 2016 (2016), 1813979. |
[4] | R. Lauche, J. Langhorst, G. Dobos and H. Cramer, A systematic review and meta-analysis of Tai Chi for osteoarthritis of the knee, Complement. Ther. Med., 21 (2013), 396–406. |
[5] | Q. G. Zhu, L. Y. Huang, X. Wu, L. Wang, Y. Zhang, M. Fang, Y. Liu and J. X. Li, Effects of Tai Ji Quan training on gait kinematics in older Chinese women with knee osteoarthritis: A randomized controlled trial. J. Sport Health Sci., 5 (2016), 297–303. |
[6] | M. C. Hochberg, R. D. Altman, K. T. April, M. Benkhalti, G. Guyatt, J. McGowan, T. Towheed, V. Welch, G. Wells and P. Tugwell, American college of rheumatology 2012 recommendations for the use of nonpharmacologic and pharmacologic therapies in osteoarthritis of the hand, hip, and knee, Arth. Care. Res., 64 (2012), 465–474. |
[7] | J. L. Astephen, K. J. Deluzio, G. E. Caldwell and M. J. Dunbar, Biomechanical changes at the hip, kneeand ankle joints during gait are associated with knee osteoarthritis severity, J. Orthop. Res., 26 (2008), 332–341. |
[8] | B. D. Jackson, A. E. Wluka, A. J. Teichtahl, M. E. Morris and F. M. Cicuttini, Reviewing knee osteoarthritis-a biomechanical perspective, J. Sci. Med. Sport, 7 (2004), 347–357. |
[9] | D. W. Mao, Y. L. Hong and J. X. Li, Characteristics of foot movement in Tai Chi exercise, Phys. Ther., 86 (2006), 215–222. |
[10] | N. Y. Law and J. X. Li, The temporospatial and kinematic characteristics of typical tai chi movements: Repulse monkey and wave-hand in cloud, Res. Sports Med., 22 (2014), 111–123. |
[11] | D. J. Hunter, Y. Q. Zhang, J. B. Niu, X. Tu, S. Amin, M. Clancy, A. Guermazi, M. Grigorian, D. Gale and D. T. Felson, The association of meniscal pathologic changes with cartilage loss in symptomatic knee osteoarthritis, Arthritis. Rheu., 54 (2010), 795–801. |
[12] | Z. Deng, K. Wang, H. H. Wang, T. Y. Lan, H. S. Zhan and W. X. Niu, A finite element study of traditional Chinese cervical manipulation, Eur. Spine J., 22 (2017), 2308–2317. |
[13] | J. Yao, G. M. Kuang, D. W. Wong, W. X. Niu, M. Zhang and Y. B. Fan, Influence of screw length and diameter on tibial strain energy density distribution after anterior cruciate ligament reconstruction, Acta. Mech. Sinica., 30 (2014), 241–249. |
[14] | G. Wu and D. Millon, Joint kinetics during Tai Chi gait and normal walking gait in young and elderly Tai Chi Chuan practitioners, Clin. Biomech., 23 (2008), 787–795. |
[15] | R. Song, B. L. Roberts, E. O. Lee, P. Lam and S. C. Bae, A randomized study of the effects of t'ai chi on muscle strength, bone mineral density, and fear of falling in women with osteoarthritis, J. Altern. Complem. Med., 16 (2010), 227–233. |
[16] | P. F. Tsai, J. Y. Chang, C. Beck, Y. F. Kuo and F. J. Keefe, A pilot cluster-randomized trial of a 20-week tai chi program in elders with cognitive impairment and osteoarthritic knee: Effects on pain and other health outcomes, J. Pain Symptom. Manage., 45 (2013), 660–669. |
[17] | K. N. Radzak, A. M. Putnam, K. Tamura, R. K. Hetzler and C. D. Stickley, Asymmetry between lower limbs during rested and fatigued state running gait in healthy individuals, Gait Posture, 51 (2017), 268–274. |
[18] | W. K. Lam, J. Ryue, K. K. Lee, S. K. Park, J. T. Cheung and J. Ryu, Does shoe heel design influence ground reaction forces and knee moments during maximum lunges in elite and intermediate badminton players? PloS One, 12 (2017), e0174604. |
[19] | G. C. Fanelli, C. J. Edson, K. N. Reinheimer and R. Garofalo, Posterior cruciate ligament and posterolateral corner reconstruction, Sports Med. Arthrosc., 15 (2007), 168–175. |
[20] | T. L. Donahue, M. B. Fisher and S. A. Maher, Meniscus mechanics and mechanobiology, J. Biomech., 48 (2015), 1341–1342. |
[21] | C. Waller, D. Hayes, J. E. Block and N. J. London, Unload it: The key to the treatment of knee osteoarthritis, Knee. Surg. Sport Tr. A., 19 (2011), 1823–1829. |
[22] | M. Taylor, K. E. Tanner and M. A. Freeman, Finite element analysis of the implanted proximal tibia: A relationship between the initial cancellous bone stresses and implant migration, J. Biomech., 31 (1998), 303–310. |
[23] | M. Kazemi, A viscoelastic poromechanical model of the knee joint in large compression, Med. Eng. Phys., 36 (2014), 998–1006. |
[24] | T. Fukubayashi and H. Kurosawa, The contact area and pressure distribution pattern of the knee. A study of normal and osteoarthrotic knee joints. Acta. Orthop. Scand., 51 (1980), 871–879. |
[25] | K. W. Chau and D. W. Mao, The characteristics of foot movements in tai chi chuan, Res. Sports Med., 14 (2006), 19–28. |
[26] | Y. Hong and J. X. Li, Biomechanics of Tai Chi: A review, Sports Biomech., 6 (2007), 453–464. |
[27] | S. D. Waldman, C. G. Spiteri, M. D. Grynpas, R. M. Pilliar and R. A. Kandel, Long-term intermittent compressive stimulation improves the composition and mechanical properties of tissue-engineered cartilage, Tissue. Eng., 10 (2004), 1323–1331. |
[28] | T. M. Griffin and F. Guilak, The role of mechanical loading in the onset and progression of osteoarthritis, Exerc. Sport Sci. Rev., 33 (2005), 195–200. |
[29] | L. E. Defrate, R. Papannagari, T. J. Gill, J. M. Moses, N. P. Pathare and G. Li, The 6 degrees of freedom kinematics of the knee after anterior cruciate ligament deficiency: An in vivo imaging analysis, Am. J. Sports Med., 34 (2006), 1240–1246. |
[30] | G. Wu, W. Liu, J. Hitt and D. Millon, Spatial, temporal and muscle action patterns of Tai Chi gait, J. Electromyogr. Kines., 14 (2004), 343–354. |
[31] | Q. H. Huang and Z. Z. Zeng, A Review on Real-Time 3D Ultrasound Imaging Technology, Biomed. Res. Int., 2017 (2017), 6027029. |
[32] | Q. H. Huang, B. W. Wu, J. L. Lan and X. L. Li, Fully Automatic Three-Dimensional Ultrasound Imaging Based on Conventional B-scan, IEEE. Trans. Biomed. Circuits. Syst., 12 (2018), 426–436. |
[33] | Q. H. Huang, J. L. Lan and X. L. Li, Robotic arm based automatic ultrasound scanning for three-dimensional imaging, IEEE. Trans. Ind. Inform. (in press). DOI: 10.1109/TII.2018.2871864. |
1. | Jianwei Duan, Kuan Wang, Tongbo Chang, Lejun Wang, Shengnian Zhang, Wenxin Niu, Tai Chi Is Safe and Effective for the Hip Joint: A Biomechanical Perspective, 2020, 28, 1063-8652, 415, 10.1123/japa.2019-0129 | |
2. | Yuze Zhang, Hao Lian, Yinghai Liu, Sandip K. Mishra, Deconstruction of Knee Cartilage Injury in Athletes Using MR Images Based on Artificial Intelligence Segmentation Algorithm, 2022, 2022, 1555-4317, 1, 10.1155/2022/4165232 | |
3. | Hao Yu, JiangNa Wang, Min Mao, QiPeng Song, Cui Zhang, Daniel T.P. Fong, Wei Sun, Muscle co-contraction and pre-activation in knee and ankle joint during a typical Tai Chi brush-knee twist-step, 2021, 1543-8627, 1, 10.1080/15438627.2021.2020788 | |
4. | Shu-zhao Zhuang, Pei-jie Chen, Jia Han, Wei-hua Xiao, Beneficial Effects and Potential Mechanisms of Tai Chi on Lower Limb Osteoarthritis: A Biopsychosocial Perspective, 2021, 1672-0415, 10.1007/s11655-021-3529-9 | |
5. | Yaoyong Zhang, Dan Zhang, Fuhao Mo, Biomechanical Analysis of Foot–Ankle Complex during Jogging with Rearfoot Strike versus Forefoot Strike, 2022, 2022, 1754-2103, 1, 10.1155/2022/2664856 | |
6. | Haojie Li, Fang Peng, Shaojun Lyu, Zhongqiu Ji, Xiongfeng Li, Mingyu Liu, Newly compiled Tai Chi (Bafa Wubu) promotes lower extremity exercise: a preliminary cross sectional study, 2023, 11, 2167-8359, e15036, 10.7717/peerj.15036 | |
7. | Patricia Huston, Why osteoarthritis of the knee is called “a wound that does not heal” and why Tai Chi is an effective treatment, 2023, 10, 2296-858X, 10.3389/fmed.2023.1208326 | |
8. | Hong Liu, Xiaolin Chen, Yaping Li, Zhen Gao, Wujie Huang, Zheng Jiang, Neuromuscular control strategies of the lower limb during a typical Tai Chi brush knee and twist step in practitioners with and without knee pain: a pilot study, 2024, 32, 1543-8627, 679, 10.1080/15438627.2023.2219799 | |
9. | T. Zahra, B. Abdelwahed, 2024, 9780323905978, 231, 10.1016/B978-0-323-90597-8.00006-2 | |
10. | Tao Tao, Ming-Peng Shi, Xian-Shuai Zhang, Bo-Yang Tan, Ya-Nan Xiao, Feng-Ling Sun, Shao-Jun Li, Zhen-Hua Li, Effects of different traditional Chinese exercise for knee osteoarthritis patients: A network meta-analysis of randomized controlled trials, 2024, 103, 0025-7974, e39660, 10.1097/MD.0000000000039660 | |
11. | Jianmin Zhao, Wei Han, Huiru Tang, Lower limbs inter-joint coordination and variability during typical Tai Chi movement in older female adults, 2023, 14, 1664-042X, 10.3389/fphys.2023.1164923 | |
12. | Samer A. Kokz, Ali M. Mohsen, Khaldoon Khalil Nile, Zainab B. Khaleel, Inductive 3D numerical modelling of the tibia bone using MRI to examine von Mises stress and overall deformation, 2024, 14, 2391-5439, 10.1515/eng-2022-0572 | |
13. | Haibo Liu, He Gong, Peng Chen, Le Zhang, Haipeng Cen, Yubo Fan, Biomechanical effects of typical lower limb movements of Chen-style Tai Chi on knee joint, 2023, 61, 0140-0118, 3087, 10.1007/s11517-023-02906-y | |
14. | Hua Xing, Xiaojie Su, Yushan Liu, Yang Chen, Yubin Ju, Zhiran Kang, Wuquan Sun, Fei Yao, Lijun Yao, Li Gong, Prediction of knee joint pain in Tai Chi practitioners: a cross-sectional machine learning approach, 2023, 13, 2044-6055, e067036, 10.1136/bmjopen-2022-067036 | |
15. | Qianhong Chen, Keren Cai, Shuyao Li, Xinlin Du, Fuqiang Wang, Yu Yang, Ming Cai, Navigating the Global Landscape of Exercise Interventions for Knee Osteoarthritis: Exploring Evolving Trends and Emerging Frontiers From a Bibliometric and Visualization Analysis Perspective (2011–2022), 2024, 25, 15258610, 105269, 10.1016/j.jamda.2024.105269 | |
16. | Lijun Hua, Gengchao Bi, Yanlong Zhang, Kai Wang, Jiao Liu, The impact of anterior knee displacement on knee joint load during the forward bow step in Tai Chi, 2024, 12, 2296-4185, 10.3389/fbioe.2024.1458737 | |
17. | Longcheng LIU, Feng ZHANG, Pengyi GAO, Tianlin ZHAO, Honghao WANG, LIU Yinuo LI, Jiaying WANG, Xiangyu ZHU, Mechanism of Tai Chi Chuan in Improving Knee Osteoarthritis from the Perspective of Sports Biomechanics, 2024, 34, 2096-0328, 411, 10.3724/SP.J.1329.2024.04015 | |
18. | Jiangbo Zhang, Bingpeng Chen, Bo Chen, Hao Wang, Qing Han, Xiongfeng Tang, Yanguo Qin, Clinical Application of Finite Element Analysis in Meniscus Diseases: A Comprehensive Review, 2025, 1134-3060, 10.1007/s11831-025-10265-0 |
Movements | Extension/Flexion (°) | Adduction/Abduction (°) | Internal/External rotation (°) |
Walking | −48~−8 | −6~2 | −9~8 |
Jogging | −32~−6 | −9~2 | −8~7 |
BKTS | −92~−1 | −16~12 | −15~14 |
Note: BKTS, Brush-knee and Twist-step. Negative angles indicate flexion/abduction/external rotation, while positive angles indicate extension/adduction/internal rotation. |
Movements | Extension/Flexion (°) | Adduction/Abduction (°) | Internal/External rotation (°) | Vertical load (% BW) |
Walking | −10.5 | −1.7 | −3.6 | 121 |
Jogging | −26 | −7.5 | 2.7 | 187 |
BKTS | −82 | −9.8 | 6.6 | 98 |
Note: BKTS, Brush-knee and Twist-step; BW, body weight. Negative angles indicate flexion/abduction/external rotation, while positive angles indicate extension/adduction/internal rotation. |
Component | Element Type | Young's modulus (MPa) | Poisson's ratio |
Bone | Tetrahedral, hexahedral | 11000 | 0.3 |
Cartilage | Hexahedral | 5 | 0.35 |
Meniscus | Hexahedral | 59 | 0.45 |
Ligaments | Truss | 215 | 0.40 |
Movements | Extension/Flexion (°) | Adduction/Abduction (°) | Internal/External rotation (°) |
Walking | −48~−8 | −6~2 | −9~8 |
Jogging | −32~−6 | −9~2 | −8~7 |
BKTS | −92~−1 | −16~12 | −15~14 |
Note: BKTS, Brush-knee and Twist-step. Negative angles indicate flexion/abduction/external rotation, while positive angles indicate extension/adduction/internal rotation. |
Movements | Extension/Flexion (°) | Adduction/Abduction (°) | Internal/External rotation (°) | Vertical load (% BW) |
Walking | −10.5 | −1.7 | −3.6 | 121 |
Jogging | −26 | −7.5 | 2.7 | 187 |
BKTS | −82 | −9.8 | 6.6 | 98 |
Note: BKTS, Brush-knee and Twist-step; BW, body weight. Negative angles indicate flexion/abduction/external rotation, while positive angles indicate extension/adduction/internal rotation. |