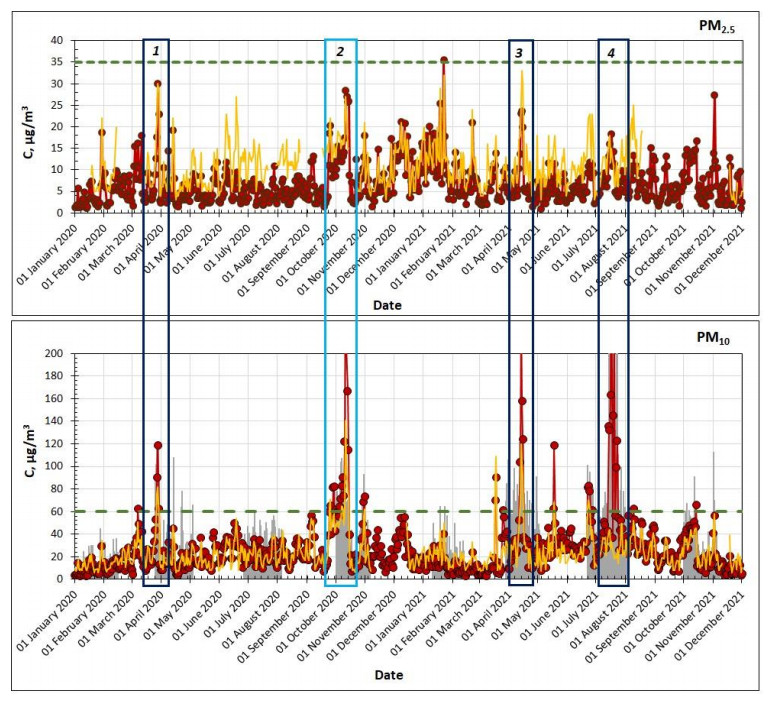
We analyzed the unique episode of long-range dust/sand atmospheric aerosol transport from the arid zones of the southern European territory of Russia, i.e. the Northern Caspian and Astrakhan regions and the Kalmykia Republic, to the Moscow region during fall 2020. Intensive complex experiments were carried out at the A.M. Obukhov Institute of Atmospheric Physics of the Russian Academy of Sciencesin the center of Moscow through different seasons during 2020–2021 to study the composition of near-surface aerosols in the Moscow megacity. The experimental data are considered to take into account the synoptic features and meteorological conditions. Abnormally high values of near-surface aerosol mass concentrations in Moscow were registered during the period from 6 to 14 of October 2020 under stable anti-cyclonic conditions, with calm or quiet/light wind (1–2 m/s), and under the conditions of the dominance of southeastern air mass transport. At the same time, the average daily mass concentration of aerosol particles PM10 exceeded the Maximum Permissible Concentration (MPC) value (60 µg/m3) by 1.5–4.5 times, and the number of large (micron) particles increased by an order or more. Comparative analysis of aerosol elemental composition in Moscow (during this episode) and in Kalmykia (according to observations in July 2020) showed high correlation for terrigenous elements' patterns. The aerosol origin for this episode was confirmed by performing long-range trajectory analysis of air mass transport (HYSPLIT model), and by using MERRA-2 reanalysis data for dust aerosol spatial distribution.
Citation: Dina Gubanova, Otto Chkhetiani, Anna Vinogradova, Andrey Skorokhod, Mikhail Iordanskii. Atmospheric transport of dust aerosol from arid zones to the Moscow region during fall 2020[J]. AIMS Geosciences, 2022, 8(2): 277-302. doi: 10.3934/geosci.2022017
[1] | Viviane Pierrard, David Bolsée, Alexandre Winant, Amer Al-Qaaod, Faton Krasniqi, Maximilien Péters de Bonhome, Edith Botek, Lionel Van Laeken, Danislav Sapundjiev, Roeland Van Malderen, Alexander Mangold, Iva Ambrozova, Marek Sommer, Jakub Slegl, Styliani A Geronikolou, Alexandros G Georgakilas, Alexander Dorn, Benjamin Rapp, Jaroslav Solc, Lukas Marek, Cristina Oancea, Lionel Doppler, Ronald Langer, Sarah Walsh, Marco Sabia, Marco Vuolo, Alex Papayannis, Carlos Granja . BIOSPHERE measurement campaign from January 2024 to March 2024 and in May 2024: Effects of the solar events on the radiation belts, UV radiation and ozone in the atmosphere. AIMS Geosciences, 2025, 11(1): 117-154. doi: 10.3934/geosci.2025007 |
[2] | Yang Sheng, Dehua Sun, Weizhong Liu . Study on the spatial variation of sensitivity of soil nutrient system in Xinjiang, China. AIMS Geosciences, 2023, 9(4): 632-651. doi: 10.3934/geosci.2023034 |
[3] | Jolanta Kwiatkowska-Malina, Andrzej Szymon Borkowski . Geostatistical modelling of soil contamination with arsenic, cadmium, lead, and nickel: the Silesian voivodeship, Poland case study. AIMS Geosciences, 2020, 6(2): 135-148. doi: 10.3934/geosci.2020009 |
[4] | David W. Schwartzman . Life’s Critical Role in the Long-term Carbon Cycle: the Biotic Enhancement of Weathering. AIMS Geosciences, 2017, 3(2): 216-238. doi: 10.3934/geosci.2017.2.216 |
[5] | Thomas Fauchez, Giada Arney, Ravi Kumar Kopparapu, Shawn Domagal Goldman . Explicit cloud representation in the Atmos 1D climate model for Earth and rocky planet applications. AIMS Geosciences, 2018, 4(4): 180-191. doi: 10.3934/geosci.2018.4.180 |
[6] | Aleksander S. Gundersen, Pasquale Carotenuto, Tom Lunne, Axel Walta, Per M. Sparrevik . Field verification tests of the newly developed flow cone tool—In-situ measurements of hydraulic soil properties. AIMS Geosciences, 2019, 5(4): 784-803. doi: 10.3934/geosci.2019.4.784 |
[7] | Chibuike Chiedozie Ibebuchi . Fuzzy time series clustering using autoencoders neural network. AIMS Geosciences, 2024, 10(3): 524-539. doi: 10.3934/geosci.2024027 |
[8] | Palavai Venkateswara Rao, Mangalampalli Subrahmanyam, Bakuru Anandagajapathi Raju . Groundwater exploration in hard rock terrains of East Godavari district, Andhra Pradesh, India using AHP and WIO analyses together with geoelectrical surveys. AIMS Geosciences, 2021, 7(2): 244-267. doi: 10.3934/geosci.2021015 |
[9] | Vadim Khomich, Svyatoslav Shcheka, Natalia Boriskina . Geodynamic factors in the formation of large gold-bearing provinces with Carlin-type deposits on continental margins in the North Pacific. AIMS Geosciences, 2023, 9(4): 672-696. doi: 10.3934/geosci.2023036 |
[10] | Attia Ahmed, Sandu Adrian . A Hybrid Monte Carlo Sampling Filter for Non-Gaussian Data Assimilation. AIMS Geosciences, 2015, 3(1): 41-78. doi: 10.3934/geosci.2015.1.41 |
We analyzed the unique episode of long-range dust/sand atmospheric aerosol transport from the arid zones of the southern European territory of Russia, i.e. the Northern Caspian and Astrakhan regions and the Kalmykia Republic, to the Moscow region during fall 2020. Intensive complex experiments were carried out at the A.M. Obukhov Institute of Atmospheric Physics of the Russian Academy of Sciencesin the center of Moscow through different seasons during 2020–2021 to study the composition of near-surface aerosols in the Moscow megacity. The experimental data are considered to take into account the synoptic features and meteorological conditions. Abnormally high values of near-surface aerosol mass concentrations in Moscow were registered during the period from 6 to 14 of October 2020 under stable anti-cyclonic conditions, with calm or quiet/light wind (1–2 m/s), and under the conditions of the dominance of southeastern air mass transport. At the same time, the average daily mass concentration of aerosol particles PM10 exceeded the Maximum Permissible Concentration (MPC) value (60 µg/m3) by 1.5–4.5 times, and the number of large (micron) particles increased by an order or more. Comparative analysis of aerosol elemental composition in Moscow (during this episode) and in Kalmykia (according to observations in July 2020) showed high correlation for terrigenous elements' patterns. The aerosol origin for this episode was confirmed by performing long-range trajectory analysis of air mass transport (HYSPLIT model), and by using MERRA-2 reanalysis data for dust aerosol spatial distribution.
Dust aerosol is one of the main polluting components of the city atmosphere, with a strong negative impact not only on the environment, but also on human health. It is the main contributor to aerosol particles in the atmosphere, and it annually forms from one-third to one-half of the total mass of aerosol emissions. Having direct and indirect effects, dust aerosol plays an active role in atmospheric processes regulating the radiation balance and climate of the earth [1,2,3,4]. According to the magnitude of the effects on the climate, dust aerosol is considered to be a natural analogue of smoke when assessing the climatic consequences of volcanic eruptions and fires [2,5,6]. In this regard, in recent decades, scientists and researchers of the world have paid special attention to assessing the radiation effect of dust aerosol in the atmosphere from various regions, and to studying the processes of its transfer, transformation and variability [4,6,7,8,9,10,11,12,13,14,15,16].
The main natural sources of dust aerosol are arid and semiarid regions of the globe, the largest of which are located in North Africa, the Arabian Peninsula, the Middle East and Central and East Asia [17,18,19]. The results of long-term ground-based and distant satellite studies and numerical calculations have shown that dust aerosol particles can be transported in the atmosphere over long distances. Therefore, spatial and temporal variabilities of the compositional, microphysical and optical characteristics of dust aerosol are being studied [17,18]. Experimental and model investigations of transboundary, long-range and regional dust aerosol transport from desert and arid zones are of great interest. A number of works are devoted to the transatlantic transport of Saharan dust, which is the most powerful global source of dust aerosol, to North and South America, for example [19,20,21,22]. Many publications describe the characteristics and conditions of dust aerosol transport from North Africa to Europe [23,24,25,26,27,28,29].
Other powerful global sources of dust aerosol are located in Central and Eastern Asia (primarily the Taklamakan and Gobi deserts) [8,9,12,30,31,32]. Over the past decades, the conditions and features of trans-Pacific transport of Asian dust to the Americas have been studied using ground-based and satellite observation tools and global numerical models [33,34,35,36,37]. The vertical structure and composition and optical and microphysical characteristics of dust aerosol in various regions of Asia were determined in [8,9,10,11,12,13]. The probability of Asian dust atmospheric transport to the Arctic was studied in [38]. The composition of the precipitation resulting from anomalous dust transport from arid zones of the Asian continent to the south of Primorsky Krai during late March to early April 2002 was studied in [39].
However, despite the great interest in dust aerosol and numerous studies of its atmospheric transport to various regions of the world, there are almost no publications on dust transport from arid and semiarid regions to the temperate latitudes of Eastern Europe and the European part of Russia. We will name only two works of this kind. The sources and parameters of dust aerosol over the Black Sea and the Crimean Peninsula were investigated in [40], and the ways of long-range dust transport from the Middle East and North Africa to mountainous regions of the Caucasus were considered in [41].
The problem of pollution and quality control of the city's air is very relevant for all countries and regions [42]. The mixing of emissions (gases and aerosols) in the surface air from various industrial enterprises, transport and life support services with emissions from natural sources (plants, soils) create a non-uniform spatial structure of air pollution in the city that rapidly changes due to the mobility of air masses and chemical activity of the constituents.
Local sources of atmospheric aerosols in the city and its suburbs, the globally distributed atmospheric components and the sink processes for aerosol particles to the surface form the background composition of the surface aerosol, which has intra-annual variability. However, in some periods, specific air mass transport is possible, leading to aerosol pollution transport from other remote regions. These episodes can significantly change the composition and radiation properties of the surface air in the city, affecting the quality of the environment and the living conditions of the population.
Moscow is the largest metropolis in Europe; it is characterized by specific features that affect the distribution of atmospheric pollutants: a special wind regime, a special orography, heterogeneity of urban landscapes, local anthropogenic sources, the influence of "heat islands", etc. They cause spatial and temporal heterogeneity and strong variability of the composition of the near-surface atmosphere in the city, including the aerosol characteristics. This has been confirmed by the data of the Budgetary environmental protection institution Mosecomonitoring (MEM) [43], which regularly monitors the air quality in different parts of Moscow, as well as by the results of episodic experimental studies of the near-surface aerosol composition in Moscow [44,45,46,47,48,49,50].
The purpose of this work was to study one unique episode from 2 years of continuous observations of near-surface aerosol composition in Moscow at the A.M. Obukhov Institute of Atmospheric Physics of the Russian Academy of Sciences (IAP RAS). During this episode, the extreme increase in the mass concentration of near-surface aerosol PM10 was registered at different sites in Moscow, according to MEM and the IAP RAS observations. We associate this phenomenon with the long-range transport of dust aerosol to the Moscow region and have made an assumption about the location of the sources of this aerosol in the semiarid zones located in the south of the European territory of Russia (ETR). The probability of long-range air mass transport from these areas to Moscow does not exceed 5% according to the estimates of previous works [51,52,53]. This hypothesis was confirmed by conducting an analysis of the distributions of aerosol mass and particle number by size, and the meteorological conditions, as well as by comparison of the elemental compositions for the studied aerosol in Moscow and the aerosol in the proposed source region. Moreover, we compared the ways of air mass transport in Moscow (trajectories calculated using the HYSPLIT 4 model [54]) and the spatial distributions of dust in the surface atmosphere (according to the MERRA-2 reanalysis [55]) on the days of excessive air pollution; the results also confirmed our assumption.
The Moscow megacity is located in the central part of the ETR. Intensive complex experiments were carried out at the IAP RAS through different seasons during 2020–2021 to study the composition of near-surface aerosol in Moscow. The observation point (55.74 °N; 37.62 °E) was located in the central part of Moscow in a zone of dense urban development, corresponding to the courtyard of IAP RAS. The instruments and equipment for aerosol sampling included the following: (1) aspiration aerosol samplers with analytical filters for aerosols (AFA-type) for subsequent gravimetric and chemical analysis; (2) 6-stage impactors with hydrophobic filters to determine the aerosol mass and elemental composition of particles with the following size ranges: < 0.5 µm, 0.5–1.5 µm, 1.5–2.5 µm, 2.5–4.0 µm, 4.0–6.5 µm and > 6.5 µm; (3) a laser aerosol spectrometer (LAS-P, Karpov Institute of Physical Chemistry, Russia) to determine the number of aerosol particles in the range of 0.15–2.0 µm passing through 10 channels every 5 min, with the total number of particles equaling up to 3000 per cubic centimeter; (4) optoelectronic aerosol spectrometers (OEAS-05) to determine the number of aerosol particles in the range of 0.2–10.0 µm passing through 10 channels every 5 min, with the total number of particles equaling up to 2000 per cubic centimeter.
Our equipment acquired aerosol samples daily at a height of about 2 m above the surface; the filter was replaced at 9 a.m. Moscow time.
We analyzed the different aerosol parameters by using the following methods and equipment: (1) distribution of the number of aerosol particles by size—with the help of laser and electron spectroscopy methods; (2) aerosol mass concentration—via gravimetry and/or by numerical calculation using particle size distribution data; (3) elemental composition—via atomic emission spectrometry (ICP-AES), inductively coupled plasma mass spectrometry (ICP-MS) and X-ray fluorescence analysis (XFA) [56,57,58]; and (4) statistics and correlation of data obtained—by using standard statistical software for automated numerical processing, and to obtain graphical representation of information.
We interpreted our data using the following resources and databases: (1) meteorological parameters for the station Balchug, which is nearest to the IAP RAS (from relevant websites) [59,60,61]; (2) backward trajectories of air mass transport to the observation point, calculated with the help of a HYSPLIT model [54]; (3) spatial distributions of dust mass concentration and AOT 550 in the near-surface atmosphere according to MERRA-2 reanalysis data (version 2) generated from satellite and measurement archives [55]; and (4) air pollution monitoring data in Moscow from the network of MEM [43].
The experiment was organized in a way that continuous daily data series were obtained for aerosol particle number and mass distributions by size in the range of 0.25–10 µm in the near-surface Moscow atmosphere over two years of observations. Furthermore, intensive complex experiments were conducted throughout 35–40 days during every season; the mass concentration of all aerosol particles (up to 30–40 µm in size) and the aerosol elemental composition were determined daily from the samples collected by using an aspiration method (the first results are in [49,50,62]).
For two years, we controlled the quality of the data obtained at the IAP RAS based on the results of observations at MEM stations, in particular, at Spiridonovka station, further called as SpMEM (Figures 1, 2). This station is located 2900 m in a NW direction from IAP RAS, in the courtyard of a multi-storey residential building, close to roads with medium and low traffic. It is the closest MEM stations to IAP RAS station and similar in terms of urban development and infrastructure.
The correlation coefficients for the daily concentrations of aerosol masses PM2.5 and PM10 between these observation points were 0.79 and 0.74, respectively (through all daily data for 2 years).
Figure 1 shows that extreme concentration splashes (above the Maximum Permissible Concentration (MPC) value for PM10) were observed rarely [63]. These are the periods when polluted air masses were brought into the Moscow atmosphere as a result of long-range air mass transport. There were the smoke aerosols from the regions with extensive fires during Episode 1, and the sand/dust aerosols from the deserted areas of the ETR during Episodes 2 and 3. The fourth episode was caused by emissions from construction and repairing works in the city itself, not far from the IAP RAS [64].
In this work, we analyzed the second episode of high aerosol concentration (HighAC), which occurred during October 6–14, 2020 (see in detail in Figure 2). During this episode, high values of aerosol PM10 concentrations were measured at all observation stations in Moscow, i.e., at the IAP RAS and 16 MEM stations. Figure 2a shows the comparison with the nearest SpMEM station during October 2019, as well as the daily averaged PM10 concentrations for October from 2007 to 2019 with standard deviations (STD). So, the 2020 event was undoubtedly unusual for this month. Figure 2b demonstrates how this episode was manifested in the data of all of the MEM observation stations, so it was not a local source of aerosol pollution.
Further, we will consider the episode of HighAC through October 1–14, 2020 in comparison with the conditionally background values of aerosol concentration (BgAC) for the second half of October (i.e., October 15–30, 2020)
The meteorological conditions of October 2020 in Moscow (Figure 3) were analyzed, and the variations of the meteorological parameters (temperature T, pressure P and relative humidity U) for near-surface air in Moscow [59,60,61] in October 2020 were compared with the ones for October 2019, as well as with the long-term (2007–2019) averages of these parameters (Figure 3). During the first half of October 2020, the Moscow atmosphere was characterized by stable anticyclone conditions, i.e., the air pressure was high and the relative humidity was atypically low in comparison with the long-term average values. Also, there was no precipitation in Moscow on these days, and the air temperature was extremely high.
Atypical weather conditions through October 1–14, 2020 were also manifested in wind characteristics; calm or quiet southeastern winds were observed in 65% of cases (Figure 4d). In contrast, in the second half of October (October 15–31, 2020), westerly winds prevailed (Figure 4e), which is similar to the wind conditions in October 2019 (Figure 4b) and to the long-term average wind rose in Moscow in October (Figure 4c). In October as a whole (Figure 4c), westerly winds with 24% and 13% calm conditions (Figure 4e and 4c, respectively) dominated in the second half of the month, and in October, on average, respectively.
The atypical wind directions from the 1st to the 4th of October 2020 were accompanied by the long-range atmospheric transport of dust/sand aerosols with warm air masses from the Caspian and Kalmykia regions to Moscow (see more detailed analysis in the next section). At the same time, high pressure, low relative humidity and a lack of precipitation could have contributed to the accumulation of pollution in the near-surface air of the city.
Air mass transport to the IAP RAS observation point was studied using the 3-day back-trajectories calculated by the NOAA HYSPLIT three-dimensional model [54] for a height of 100 m (8 trajectories per day, every 3 hours).
Figure 5 shows these trajectories for several days from October 8–15, 2020 on the maps showing the distribution of the total dust mass concentrations and AOT 550 calculated according to MERRA-2 reanalysis data [55] (model: M2T1NXAER v.5.12.4). Each map covers the region within the coordinates [(40 °N – 60 °N) × (30 °E – 50 °E)], with hourly averaged reanalysis data in geographical coordinate cells 0.5° × 0.625°.
Figure 5 presents the paths of air mass transport carrying pollution from the areas of dust storms in the northwestern zone of the Caspian coast (Kalmykia region) to the center of the ETR during October 10–13. One can see that the maximal values of the reanalyzed dust concentration in Moscow were, on October 11–12, in agreement with the results in Figure 2. The important atmospheric processes had not yet developed on October 8, and had ended on October 15 due to changes in the air mass and direction of the long-range air transport. So, we registered the aerosol from Kalmykia in the atmosphere of Moscow during the HighAC period. According to the MERRA-2 reanalysis estimates, AOT (550 nm) increased by 10 times or more during the HighAC episode under consideration relative to the BgAC period; this could bring corrections to the radiation properties of the atmosphere in the region.
Figure 6 shows the changes in the composition of the near-surface aerosol in Moscow during October 2020—on the scales of microns (diameter, D > 1 µm) and submicrons (D < 1 µm)—according to the IAP RAS measurements. We can see a dramatic increase in the particle number concentration for larger (micron) particles in Moscow (Figure 6a) precisely during the days of the increase in aerosol mass concentration (Figure 2); this is in agreement with our hypothesis about the aerosol origin from arid areas with coarse aerosols. The number of smaller particles was practically the same as in previous days, and obviously unrelated to the air mass changing.
Figure 6a and b show the changes in aerosol size distribution in Moscow for the HighAC and BgAC periods. Figure 6b also includes the data for the aerosol in Kalmykia that were acquired from the expedition data at the end of July 2020. There was good accordance between the Kalmykia aerosol and Moscow one during the HighAC episode; in contrast, the BgAC Moscow aerosol had a significantly smaller number of micron particles. This result also confirms the assumption of long-range aerosol transport from Kalmykia to Moscow during the discussed period of high aerosol air pollution in Moscow.
The mass concentrations of more than 60 chemical elements of various origins, such as the elements related to global distribution, rare earth and radioactive elements, heavy metals and metalloids, were studied daily for the Moscow aerosol analysis, as well as on average over several days at the end of July 2020 for the aerosol in Kalmykia. For more detailed analysis, we chose 33 elements of different origins. Further, we compared the aerosol elemental compositions during HighAC and BgAC periods in Moscow and that for Kalmykia (Figure 7).
We not only analyzed the elemental concentrations, but also the enrichment factors (EFs) relative to the earth's crust elemental composition (according to [65]). The EF shows how the ratio of any element concentration (СEl) to the reference element (CLa) in an aerosol sample differs from the ratio of the same element concentrations in the earth's crust: EF = (СEl / CLa)aer/(СEl / CLa)cr, where the reference element is lanthanum. Level EF = 10 (dashed line in Figure 7b) divides elements by origin into predominantly terrigenous elements (EF < 10) or anthropogenic/technogenic ones (EF > 10). Figure 7a and b present geochemical patterns (on logarithmic scales) of the selected 33 elements in terms of their concentrations or EFs during the periods of HighAC and BgAC in Moscow, as well as in Kalmykia.
We can see in Figure 7b that the near-surface Moscow aerosol, even during the undisturbed BgAC period, was enriched with heavy metals, metalloids and sulfur. The sources of these elements in a large city are primarily associated with transport or fuel and energy complexes [66], particularly, the emissions of engine oil and particles of fuel combustion products (Sb, Zn, Cu, Pb, Mo), abrasion of road surfaces (As, Zn, As, W, Cr, V, Co), tires (Sb, Cd, Zn, Pb, Cu, Co, Ni, Cr) and brake pads (Sb, As, Zn, Cu, Pb, Ni, W, Cr).
On the contrary, the elements Li, Be, B, Mg, Al, P, K, Ca, Sc, Ti, V, Cr, Mn, Fe, Co, Ga, As, Se, Rb, Sr, Y, Zr, Nb, Cs, Ba, La, Hf, Tl, Th and U have predominantly terrigenous origins, even in the city (Figure 7b), and the geochemical pattern of their concentrations during a period of HighAC in Moscow is close to the pattern of concentrations in the aerosol in Kalmykia (Figure 7a).
Table 1 shows the mass concentrations of chemical elements in the surface aerosol in Moscow. The correlation coefficients for the aerosol element concentration profiles for Kalmykia and Moscow were equal to 0.89 outside of the episode of HighAC in Moscow (IAP RAS_BgAC period), 0.97 during the episode of HighAC (IAP RAS_HighAC period) and 0.99 for the values in excess of the element concentrations in Moscow during the episode above the background level (IAP RAS_HighAC—IAP RAS_BgAC). This confirms the similarity of the compositions of the Kalmykia aerosol and the aerosol added to the background one during the days of increasing concentration of near-surface aerosol in Moscow.
Concentration, μg/m3 | |||
IAP RAS_BgAC | IAP RAS_HighAC | Kalmykia | |
Li | 0.00038 | 0.00188 | 0.00269 |
Na | 0.11048 | 0.33595 | 0.10146 |
Mg | 0.21502 | 0.76953 | 1.11110 |
Al | 0.40447 | 2.27913 | 4.02859 |
P | 0.01415 | 0.07306 | 0.08044 |
S | 0.53039 | 1.18803 | 0.49865 |
K | 0.19561 | 0.91432 | 1.12341 |
Ca | 1.93637 | 4.64651 | 5.08880 |
Fe | 0.99467 | 2.88129 | 2.90630 |
Cs | 0.00007 | 0.00032 | 0.00035 |
Ba | 0.01526 | 0.03768 | 0.01918 |
Sr | 0.00511 | 0.01754 | 0.01678 |
V | 0.00187 | 0.00640 | 0.00796 |
Cr | 0.00341 | 0.00821 | 0.00725 |
Mn | 0.01842 | 0.06016 | 0.05902 |
Co | 0.00039 | 0.00103 | 0.00136 |
Ni | 0.00571 | 0.00860 | 0.00276 |
Cu | 0.01473 | 0.02618 | 0.00464 |
Zn | 0.03199 | 0.06720 | 0.00913 |
Mo | 0.00054 | 0.00082 | 0.00007 |
Cd | 0.00018 | 0.00072 | 0.00008 |
W | 0.00037 | 0.00015 | 0.00002 |
Hg | 0.00001 | 0.00002 | 0.00001 |
Pb | 0.01259 | 0.03688 | 0.00253 |
Hf | 0.00003 | 0.00007 | 0.00008 |
As | 0.00029 | 0.00171 | 0.00080 |
Se | 0.00020 | 0.00038 | 0.00031 |
Sn | 0.00272 | 0.00424 | 0.00024 |
Sb | 0.00186 | 0.00345 | 0.00012 |
Bi | 0.00014 | 0.00027 | 0.00003 |
La | 0.00039 | 0.00175 | 0.00245 |
Th | 0.00009 | 0.00042 | 0.00058 |
U | 0.00004 | 0.00010 | 0.00009 |
The results in Table 1 gave us additional information to roughly divide all 33 elements under consideration into the following three groups:
(1) Li, Mg, Al, P, K, Ca, Fe Cs, Sr, V, Cr, Mn, Co, Hf, La, Th and U (highlighted in yellow)—the terrigenous elements brought from Kalmykia (Note that the concentrations of these elements in the aerosols of Kalmykia were higher or close to the concentrations in Moscow aerosols during the episode and when there were significantly higher background values.); (2) Na, S, Ba, Hg and Se (highlighted in gray)—elements related to global distribution (Note that their concentrations were approximately equal in Moscow and in Kalmykia.); (3) Ni, Cu, Zn, Mo, Cd, W, Pb, As, Sn, Sb and Bi—other elements of predominantly local origin in Moscow and/or in its suburbs (Note that their concentrations in Moscow during the episode were noticeably higher than the background values outside of the episode, which, in turn, were higher or close to the concentrations in the aerosol of Kalmykia.).
Thus, for the first time, a unique phenomenon of stable (5 days) atmospheric transport of aerosols (mainly dust and sand) from the arid zones of the ETR, i.e., approximately from the area inside the coordinates (45–50) °N, (45–50) °E, to the Moscow region (nearby 57 °N, 35 °E) have been recorded from background measurements taken in the real urban atmosphere. As it was estimated in [51,52,53], the probability of such an event in the autumn period (according to statistics through 1986–2016) does not exceed 5%.
According to the daily monitoring data on near-surface aerosol composition in Moscow (at the IAP RAS station), extremely high values of aerosol mass and particle number concentrations were detected on October 9–14, 2020. At the same time, the mass concentration of PM10 aerosol exceeded the MPC value for the surface air of residential areas (60 μg/m3 according to Russian standards).
During this episode, the remote sources of the aerosol were dust storms in the desert and arid areas of the Southern ETR and the northern coast of the Caspian Sea, as confirmed by analysis of the air mass transport trajectories to the Moscow region (NOAA HYSPLIT 4 model), as well as the dust and AOT 550 spatial fields from the MERRA-2 reanalysis data. This is a rare phenomenon for Moscow; the probability of it is no more than 5%.
The analysis of the microphysical properties and elemental composition of the Moscow aerosol during the episode of high atmospheric pollution (October 9–14) and outside it (October 15–31), as well as the analysis of aerosol from Kalmykia (July 2020), confirmed the origin of the episode under consideration A good correspondence was established in aerosol particle size distributions (in the range of diameter from 0.8 to 5 μm) for the Kalmykia and Moscow aerosols during the period of atmospheric transport from the arid areas to the city.
The aerosol elemental compositions for Kalmykia and Moscow during the period of maximum aerosol pollution were found to include elements with mineral origins (i.e., Na, Mg, Al, K, Ca, Fe and Mn), as well as for a number of other elements with origins related to global and regional distribution, which were mainly of terrigenous nature (i.e., Be, B, Sc, Ti, V, Cr, Co, Ga, Rb, Sr, Y, Zr, Ba, La, Th and U).
The analysis of the EFs of chemical elements compared to the composition of the earth's crust showed that, on background days, in the absence of transport of desert aerosol to the city, the surface aerosol in Moscow is most enriched (EF > 10) with heavy metals, metalloids and sulfur, particularly the following anthropogenic elements: S, Co, Ni, Cu, Zn, Se, Mo, Cd, Sn, Sb, Te, Pb and Bi. Their sources in a large city are mainly connected to transport and energy enterprises. In the case of the aerosol of Kalmykia, heavy metals and metalloids of anthropogenic origin accumulated to the least extent.
Thus, for the first time, a unique phenomenon of stable atmospheric transport of aerosols (mainly dust and sand) from the arid zones of the ETR, i.e., approximately from the area inside the coordinates (45–50) °N, (45–50) °E, to the Moscow region (nearby 57 °N, 35 °E) was registered by performing direct measurements in Moscow.
Since high concentrations of aerosol particles change the optical properties of the atmosphere, the registration of such phenomena and the subsequent assessment of their significance in relation to the radiation properties of the atmosphere are necessary in order to understand the influence of remote arid southern regions on the climatic parameters of the atmosphere in the middle latitudes of the Northern Hemisphere.
The reported study was funded by the Russian Foundation for Basic Research (project number 19–05–50088: Study of near-surface aerosol composition in Moscow), by the Russian Science Foundation (project number 20–17–00214: Study of arid aerosol composition in Kalmykia) and by the Ministry of Science and Higher Education within the State (assignment number 129–2022–0012: Modeling changes in the gas and aerosol composition of the atmosphere based on ground-based and satellite monitoring methods). The authors thank V.A. Lebedev, Y.V. Zhulanov and A.A. Khapaev for help in organizing and carrying out the field observations of near-surface aerosol parameters in Moscow and Kalmykia.
The authors declare no conflict of interests.
[1] | Seinfeld J, Pandis S (2006) Atmospheric Chemistry and Physics: From Air Pollution to Climate Change, 2nd Ed., New York: Wiley. |
[2] |
Cess R, Potter G, Ghan S, et al. (1985) The climatic effects of large injections of atmospheric smoke and dust: A study of climate feedback mechanisms with one- and three-dimensional climate models. J Geophys Res 90: 12937–12950. https://doi.org/10.1029/JD090iD07p12937 doi: 10.1029/JD090iD07p12937
![]() |
[3] |
Schepanski K (2018) Transport of mineral dust and its impact on climate. Geosciences 8: 151. https://doi.org/10.3390/geosciences8050151 doi: 10.3390/geosciences8050151
![]() |
[4] |
Chaibou S, Ma A, Sha T (2020) Dust radiative forcing and its impact on surface energy budget over West Africa. Sci Rep 10: 12236. https://doi.org/10.1038/s41598-020-69223-4 doi: 10.1038/s41598-020-69223-4
![]() |
[5] | Budyko M, Golitsyn G, Izrael Y (1988) Global climatic catastrophes, Berlin, New York: Springer-Verlag. |
[6] |
Brinkman A, McGregor J (1983) Solar radiation in dense Saharan aerosol in northern Nigeria. Quart J Roy Met Soc 109: 831–847. https://doi.org/10.1256/smsqj.46209 doi: 10.1256/smsqj.46209
![]() |
[7] | Gorchakova I, Mokhov I, Rublev A (2015) Radiation and temperature effects of the intensive injection of dust aerosol into the atmosphere. Izv Atmos Ocean Phys 51: 113–126. |
[8] |
Hu ZY, Huang JP, Zhao C, et al. (2020) Modeling dust sources, transport, and radiative effects at different altitudes over the Tibetan Plateau. Atmos Chem Phys 20: 1507–1529. https://doi.org/10.5194/acp-20-1507-2020 doi: 10.5194/acp-20-1507-2020
![]() |
[9] |
Huang JP, Minnis P, Chen B, et al. (2008) Long-range transport and vertical structure of Asian dust from CALIPSO and surface measurements during PACDEX. J Geophys Res 113. https://doi.org/10.1029/2008JD010620 doi: 10.1029/2008JD010620
![]() |
[10] |
Yang L, Shi Z, Sun H, et al. (2021) Distinct effects of winter monsoon and westerly circulation on dust aerosol transport over East Asia. Theor Appl Climatol 144: 1031–1042. https://doi.org/10.1007/s00704-021-03579-z doi: 10.1007/s00704-021-03579-z
![]() |
[11] |
Vijayakumara K, Devara PCS, Vijaya Bhaskara Rao S, et al. (2016) Dust aerosol characterization and transport features based on combined ground-based, satellite and model-simulated data. Aeolian Res 21: 75–85. https://doi.org/10.1016/j.aeolia.2016.03.003 doi: 10.1016/j.aeolia.2016.03.003
![]() |
[12] |
Zayakhanov A, Zhamsueva G, Naguslaev S, et al. (2012) Spatiotemporal characteristics of the atmospheric AOD in the Gobi Desert according to data of the ground-based observations. Atmos Ocean Opt 25: 346–354. https://doi.org/10.1134/S1024856012050119 doi: 10.1134/S1024856012050119
![]() |
[13] |
Abdullaev S, Maslov V, Nazarov B, et al. (2015) The elemental composition of soils and dust aerosol in the south-central part of Tajikistan. Atmos Ocean Opt 28: 347–358. https://doi.org/10.1134/S1024856015040028 doi: 10.1134/S1024856015040028
![]() |
[14] |
Xiong J, Zhao T, Bai Y, et al. (2020) Climate characteristics of dust aerosol and its transport in major global dust source regions. J Atmos Sol-Terr Phys 209: 105415. https://doi.org/10.1016/j.jastp.2020.105415 doi: 10.1016/j.jastp.2020.105415
![]() |
[15] |
Ginoux P, Prospero J, Gill T, et al. (2012) Global-scale attribution of anthropogenic and natural dust sources and their emission rates based on MODIS Deep Blue aerosol products. Rev Geophys 50: RG3005. https://doi.org/10.1029/2012RG000388 doi: 10.1029/2012RG000388
![]() |
[16] |
Prospero J (1999) Long-range transport of mineral dust in the global atmosphere: Impact of African dust on the environment of the southeastern United States. PNAS 96: 3396–3403. https://doi.org/10.1073/pnas.96.7.3396 doi: 10.1073/pnas.96.7.3396
![]() |
[17] |
Maring H, Savoie D, Izaguirre M, et al. (2003) Mineral dust aerosol size distribution change during atmospheric transport. J Geophys Res 108: 8592. https://doi.org/10.1029/2002JD002536 doi: 10.1029/2002JD002536
![]() |
[18] |
Zhang YD, Cai YJ, Yu FQ, et al (2021) Seasonal variations and long-term trend of mineral dust aerosols over the Taiwan Region. Aerosol Air Qual Res 21: 200433. https://doi.org/10.4209/aaqr.2020.07.0433 doi: 10.4209/aaqr.2020.07.0433
![]() |
[19] |
Guedes A, Landulfo E, Montilla-Rosero E, et al. (2018) Detection of Saharan mineral dust aerosol transport over Brazilian northeast through a depolarization lidar. EPJ Web Conf 176: 05036. https://doi.org/10.1051/epjconf/201817605036 doi: 10.1051/epjconf/201817605036
![]() |
[20] |
Weinzierl B, Ansmann A, Prospero JM, et al. (2017) The Saharan aerosol long-range transport and aerosol–cloud-interaction experiment: Overview and selected highlights. BAMS 98: 1427–1451. https://doi.org/10.1175/BAMS-D-15-00142.1 doi: 10.1175/BAMS-D-15-00142.1
![]() |
[21] |
Does M, Knippertz P, Zschenderlein P, et al. (2018) The mysterious long-range transport of giant mineral dust particles. Sci Adv 4. https://doi.org/10.1126/sciadv.aau2768 doi: 10.1126/sciadv.aau2768
![]() |
[22] |
Asher EC, Christensen JN, Post A, et al. (2018) The transport of Asian dust and combustion aerosols and associated ozone to North America as observed from a mountaintop monitoring site in the California coast range. JGR Atmosphere 123: 4890–4909. https://doi.org/10.1029/2017JD028075 doi: 10.1029/2017JD028075
![]() |
[23] |
Akritidis D, Katragkou E, Georgoulias AK, et al. (2020) A complex aerosol transport event over Europe during the 2017 Storm Ophelia in CAMS forecast systems: Analysis and evaluation. Atmos Chem Phys 20: 13557–13578. https://doi.org/10.5194/acp-20-13557-2020 doi: 10.5194/acp-20-13557-2020
![]() |
[24] |
Ansmann A, Bösenberg J, Chaikovsky A, et al. (2003) Long-range transport of Saharan dust to northern Europe: The 11–16 October 2001 outbreak observed with EARLINET. J Geophys Res 108: 4783. https://doi.org/10.1029/2003JD003757 doi: 10.1029/2003JD003757
![]() |
[25] |
Conceição R, Silva HG, Mirão SJ, et al. (2018) Saharan dust transport to Europe and its impact on photovoltaic performance: A case study of soiling in Portugal. Sol Energy 160: 94–102. https://doi.org/10.1016/j.solener.2017.11.059 doi: 10.1016/j.solener.2017.11.059
![]() |
[26] |
Israelevich P, Ganor E, Alpert P, et al. (2012) Predominant transport paths of Saharan dust over the Mediterranean Sea to Europe. JGR Atmosphere 117: 2205. https://doi.org/10.1029/2011JD016482 doi: 10.1029/2011JD016482
![]() |
[27] |
Kaskaoutis D, Kosmopoulos G, Nastos P, et al. (2012) Transport pathways of Sahara dust over Athens, Greece as detected by MODIS and TOMS. Geomathematics Nat Hazards Risk 3: 35–54. https://doi.org/10.1080/19475705.2011.574296 doi: 10.1080/19475705.2011.574296
![]() |
[28] |
Pey J, Querol X, Alastuey A, et al. (2013) African dust outbreaks over the Mediterranean Basin during 2001–2011: PM10 concentrations, phenomenology and trends, and its relation with synoptic and mesoscale meteorology. Atmos Chem Phys 13: 1395–1410. https://doi.org/10.5194/acp-13-1395-2013 doi: 10.5194/acp-13-1395-2013
![]() |
[29] |
Salvador P, Artíñano B, Molero F, et al. (2013) African dust contribution to ambient aerosol levels across central Spain: Characterization of long-range transport episodes of desert dust. Atmos Res 127: 117–129. https://doi.org/10.1016/j.atmosres.2011.12.011 doi: 10.1016/j.atmosres.2011.12.011
![]() |
[30] |
Filonchyk M (2022) Characteristics of the severe March 2021 Gobi Desert dust storm and its impact on air pollution in China. Chemosphere 287: 132219. https://doi.org/10.1016/j.chemosphere.2021.132219 doi: 10.1016/j.chemosphere.2021.132219
![]() |
[31] |
Filonchyk M, Peterson M (2022) Development, progression, and impact on urban air quality of the dust storm in Asia in March 15–18, 2021. Urban Clim 41: 101080. https://doi.org/10.1016/j.uclim.2021.101080 doi: 10.1016/j.uclim.2021.101080
![]() |
[32] |
Filonchyk M, Hurynovich V (2020) Spatial distribution and temporal variation of atmospheric pollution in the South Gobi Desert, China, during 2016–2019. Environ Sci Pollut Res 27: 26579–26593. https://doi.org/10.1007/s11356-020-09000-y doi: 10.1007/s11356-020-09000-y
![]() |
[33] |
Guo JP, Lou MY, Miao YC, et al. (2017) Trans-Pacific transport of dust aerosols from East Asia: Insights gained from multiple observations and modeling. Environ Pollut 230: 1030–1039. https://doi.org/10.1016/j.envpol.2017.07.062 doi: 10.1016/j.envpol.2017.07.062
![]() |
[34] | Wu M, Liu X, Luo T, et al. (2017) Trans-Pacific transport of Asian dust: The CESM model analysis and comparison with satellite observations. Amer Geophys Union. Available from: https://ui.adsabs.harvard.edu/abs/2017AGUFM.A33F2441W/abstract |
[35] |
Yumimoto K, Eguchi K, Uno IR, et al. (2010) Summertime trans-Pacific transport of Asian dust. Geophys Res Lett 37. https://doi.org/10.1029/2010GL043995 doi: 10.1029/2010GL043995
![]() |
[36] |
Han Y, Fang X, Zhao T, et al. (2008) Long range trans-Pacific transport and deposition of Asian dust aerosols. J Environ Sci 20: 424–428. https://doi.org/10.1016/s1001-0742(08)62074-4 doi: 10.1016/s1001-0742(08)62074-4
![]() |
[37] |
Wuebbles DJ, Lei H, Lin JT (2007) Intercontinental transport of aerosols and photochemical oxidants from Asia and its consequences. Environ Pollut 150: 65–84. https://doi.org/10.1016/j.envpol.2007.06.066 doi: 10.1016/j.envpol.2007.06.066
![]() |
[38] |
Huang Z, Huang J, Hayasaka T, et al. (2015) Short-cut transport path for Asian dust directly to the Arctic: A case study. Environ Res Lett 10: 114018. https://doi.org/10.1088/1748-9326/10/11/114018 doi: 10.1088/1748-9326/10/11/114018
![]() |
[39] | Kondratyev I, Kachur A, Yurchenko S, et al. (2005) Synoptic and geochemical aspects of abnormal dust transfer in south Primorskii krai. Vestn Far East Branch Russ Acad Sci 3: 55–65. (In Russian) |
[40] |
Kalinskaya D, Papkova A, Varenik A (2021) The case of absorbing aerosol anomalous transport over the Black Sea in the spring of 2020. Sovr Probl DZZ Kosm 18: 287–298. (In Russian). https://doi.org/10.21046/2070-7401-2021-18-2-287-298 doi: 10.21046/2070-7401-2021-18-2-287-298
![]() |
[41] |
Kutuzov S, Mikhalenko V, Shahgedanova M, et al. (2014) Ways of far-distance dust transport onto Caucasian glaciers and chemical composition of snow on the Western plateau of Elbrus. Ice Snow 54: 5–15. (In Russian). https://doi.org/10.15356/2076-6734-2014-3-5-15 doi: 10.15356/2076-6734-2014-3-5-15
![]() |
[42] |
Sokhi R, Singh V, Querolet X, et al. (2021) A global observational analysis to understand changes in air quality during exceptionally low anthropogenic emission conditions. Environ Intern 157: 106818. https://doi.org/10.1016/j.envint.2021.106818 doi: 10.1016/j.envint.2021.106818
![]() |
[43] | Mosecomonitoring. Measuring stations. Available from: https://mosecom.mos.ru/measuring-stations/ |
[44] | Ogorodnikov B (1996) Parameters of aerosols in the atmospheric boundary layer over Moscow. Izv RAN Fizika Atmosfery i Okeana 32: 163–171. (In Russian) |
[45] |
Gubanova DP, Belikov IB, Elansky NF, et al. (2018) Variations in PM2.5 surface concentration in Moscow according to observations at MSU meteorological observatory. Atmos Ocean Opt 31: 290–299. https://doi.org/10.1134/S1024856018030065 doi: 10.1134/S1024856018030065
![]() |
[46] |
Trefilova AV, Artamonova MS, Kuderina TM, et al. (2013) Chemical composition and microphysical characteristics of atmospheric aerosol over Moscow and its vicinity in June 2009 and during the fire peak of 2010. Izv Atmos Ocean Phys 49: 765–778. https://doi.org/10.1134/S0001433813070062 doi: 10.1134/S0001433813070062
![]() |
[47] |
Kasimov N, Vlasov DV, Kosheleva NE (2020) Enrichment of road dust particles and adjacent environments with metals and metalloids in eastern Moscow. Urban Clim 32: 100638. https://doi.org/10.1016/j.uclim.2020.100638 doi: 10.1016/j.uclim.2020.100638
![]() |
[48] |
Chubarova N, Androsova Y, Lezina Y (2021) The dynamics of the atmospheric pollutants during the Covid-19 pandemic 2020 and their relationship with meteorological conditions in Moscow. GES 14: 168–182. https://doi.org/10.24057/2071-9388-2021-012 doi: 10.24057/2071-9388-2021-012
![]() |
[49] |
Gubanova DP, Elansky NF, Skorokhod AI, et al. (2020) Physical and chemical properties of atmospheric aerosols in Moscow and its suburb for climate assessments. IOP Conf Ser Earth Environ Sci 606: 012019. https://doi.org/10.1088/1755-1315/606/1/012019 doi: 10.1088/1755-1315/606/1/012019
![]() |
[50] |
Gubanova DP, Iordanskii MA, Kuderina TM, et al. (2021) Elemental composition of aerosols in the ground air of Moscow: Seasonal changes in 2019 and 2020. Atmos Ocean Opt 34: 475–482. https://doi.org/10.1134/S1024856021050122 doi: 10.1134/S1024856021050122
![]() |
[51] |
Shukurov K, Shukurova L (2019) Aral's potential sources of dust for Moscow region. E3S Web Conf 99: 02015. https://doi.org/10.1051/e3sconf/20199902015 doi: 10.1051/e3sconf/20199902015
![]() |
[52] |
Shukurov KA, Shukurova LM (2017) Source regions of ammonium nitrate, ammonium sulfate, and natural silicates in the surface aerosols of Moscow oblast. Izv Atmos Ocean Phys 53: 316–325. https://doi.org/10.1134/S0001433817030136 doi: 10.1134/S0001433817030136
![]() |
[53] |
Shukurov K, Chkhetiani O (2017) Probability of transport of air parcels from the arid lands in the southern Russia to Moscow region. Proc SPIE 10466. https://doi.org/10.1117/12.2287932 doi: 10.1117/12.2287932
![]() |
[54] | HYSPLIT. Available from: www.arl.noaa.gov |
[55] | MERRA-2. Available from: https://giovanni.gsfc.nasa.gov/giovanni/ |
[56] | Erhardt H (1985) Rentgenofluorescentnyj Analiz. Primenenie v Zavodskih Laboratorijah, Moscow: Metallurgija. (In Russian). |
[57] | Kudryashov V (1997) Analysis of the elemental composition atmospheric aerosols by physical methods. In Problemy Fiziki Atmosfery: Mezhvuzovskii Sbornik[Problems of Atmospheric Physics: Interuniversity Transactions], Saint-Petersburg: SPbGU, 97–130. (In Russian) |
[58] | Karandashev V, Turanov A, Orlova T, et al. (2007) Use of mass spectrometry with inductively coupled plasma method for element analysis of surrounding medium objects. Russ Zavod Lab Diagn Mater 73: 12–22. (In Russian). Available from: https://www.elibrary.ru/item.asp?id=9470754 |
[59] | Reliable prognosis. (In Russian). Available from: https://rp5.ru |
[60] | Windy. (In Russian). Available from: https://www.windy.com/ru. |
[61] | Weatherarchive. (In Russian). Available from: http://weatherarchive.ru/Pogoda/Moscow. |
[62] |
Gubanova DP, Vinogradova AA, Iordanskii MA, et al. (2021) Time variations in the composition of atmospheric aerosol in Moscow in spring 2020. Izv Atmos Ocean Phys 57: 297–309. https://doi.org/10.1134/S0001433821030051 doi: 10.1134/S0001433821030051
![]() |
[63] |
Gubanova DP, Vinogradova AA, Iordanskii MA, et al. (2022) Variability of near-surface aerosol composition in Moscow in 2020–2021: Episodes of extreme air pollution of different genesis. Atmosphere 13: 574. https://doi.org/10.3390/atmos13040574 doi: 10.3390/atmos13040574
![]() |
[64] |
Gubanova DP, Vinogradova AA, Skorokhod A, et al. (2021) Abnormal aerosol air pollution in Moscow near the local anthropogenic source in July 2021. Hydrometeorological Res Forecast 4: 134–148. https://doi.org/10.37162/2618-9631-2021-4-134-148 doi: 10.37162/2618-9631-2021-4-134-148
![]() |
[65] | Vinogradov A (1962) Srednie soderzhaniya khimicheskikh elementov v glavnykh tipakh izverzhennykh gornykh porod zemnoy kory[Average content of chemical elements in major types of erupted rocks of the Earth crust]. Geokhimiya [Geochemistry] 7: 555–571. |
[66] | Kasimov NS, Vlasov DV, Kosheleva NE, et al. (2016) Geochemistry of landscapes of eastern Moscow, Moscow: APR. (In Russian). |
1. | A. A. Vinogradova, D. P. Gubanova, M. A. Iordanskii, A. I. Skorokhod, Effect of Meteorological Conditions and Long-Range Air Mass Transport on Surface Aerosol Composition in Winter Moscow, 2022, 35, 1024-8560, 758, 10.1134/S1024856022060276 | |
2. | Dina P. Gubanova, Anna A. Vinogradova, Nataliya V. Sadovskaya, Brochosomes and Other Bioaerosols in the Surface Layer of the Atmosphere of Moscow Metropolis, 2023, 14, 2073-4433, 504, 10.3390/atmos14030504 | |
3. | Karim Abdukhakimovich Shukurov, Denis Valentinovich Simonenkov, Aleksei Viktorovich Nevzorov, Alireza Rashki, Nasim Hossein Hamzeh, Sabur Fuzaylovich Abdullaev, Lyudmila Mihailovna Shukurova, Otto Guramovich Chkhetiani, CALIOP-Based Evaluation of Dust Emissions and Long-Range Transport of the Dust from the Aral−Caspian Arid Region by 3D-Source Potential Impact (3D-SPI) Method, 2023, 15, 2072-4292, 2819, 10.3390/rs15112819 | |
4. | D. P. Gubanova, A. A. Vinogradova, E. I. Kotova, Long-Range Atmospheric Transport of Dust from the Caspian Sea Region to the Arctic Zone of the European Part of Russia in December 2023, 2024, 1028-334X, 10.1134/S1028334X24602852 | |
5. | D. P. Gubanova, A. A. Vinogradova, E. A. Lezina, M. A. Iordanskii, A. A. Isakov, Conditionally Background Level of Aerosol Pollution of Near-Surface Air in Moscow and One of Its Suburbs: Seasonal Variations, 2023, 59, 0002-3515, 754, 10.31857/S0002351523060056 | |
6. | A. A. Vinogradova, D. P. Gubanova, V. M. Kopeikin, Variability of Black Carbon, PM10 and PM2.5 in the Near-Surface Aerosol in Moscow Megalopolis, 2024, 60, 0001-4338, 272, 10.1134/S0001433824700324 | |
7. | D. P. Gubanova, A. A. Vinogradova, E. A. Lezina, M. A. Iordanskii, A. A. Isakov, Conditional Background Level of Aerosol Pollution in Surface Air in Moscow and One of its Suburbs: Seasonal Variations, 2023, 59, 0001-4338, 667, 10.1134/S0001433823060051 | |
8. | D. P. Gubanova, N. V. Sadovskaya, M. A. Iordanskii, A. S. Avilov, V. M. Minashkin, Morphology of surface aerosol particles in Moscow via scanning electron microscopy, 2023, 87, 0367-6765, 1374, 10.31857/S036767652370240X | |
9. | D. P. Gubanova, N. V. Sadovskaya, M. A. Iordanskii, A. S. Avilov, V. M. Minashkin, Morphology of Surface Aerosol Particles in Moscow via Scanning Electron Microscopy, 2023, 87, 1062-8738, 1411, 10.3103/S1062873823703409 | |
10. | A. A. Vinogradova, D. P. Gubanova, E. A. Lezina, Yu. A. Ivanova, Dust Aerosol from the Northern Caspian Sea Regions in Near-Surface Air in the Center of European Russia, 2024, 37, 1024-8560, 620, 10.1134/S1024856024700842 | |
11. | A. A. Vinogradova, D. P. Gubanova, V. M. Kopeykin, Variability of black carbon and aerosols РМ10 and РМ2.5 in the near-surface air of the megalopolis, 2024, 60, 0002-3515, 320, 10.31857/S0002351524030054 | |
12. | D. P. Gubanova, A. A. Vinogradova, E. A. Lezina, Spatial and Temporal Distribution of Near-Surface Aerosol Concentration as a Factor of Diversity of Living Conditions in a Megapolis, 2024, 37, 1024-8560, S105, 10.1134/S1024856024701458 | |
13. | A. A. Vinogradova, D. P. Gubanova, V. M. Antonova, A. V. Talovskaya, Elemental Composition of Near-Surface Aerosols in Moscow and Its Suburb: Common Features and Differences throughout a Year, 2024, 37, 1024-8560, S94, 10.1134/S1024856024701653 | |
14. | D. P. Gubanova, A. S. Ginzburg, A. A. Vinogradova, O. G. Chkhetiani, V. A. Semenov, The “Dust Haze” Effect in the Atmosphere of a Megapolis, 2025, 522, 1028-334X, 10.1134/S1028334X25605681 |
Concentration, μg/m3 | |||
IAP RAS_BgAC | IAP RAS_HighAC | Kalmykia | |
Li | 0.00038 | 0.00188 | 0.00269 |
Na | 0.11048 | 0.33595 | 0.10146 |
Mg | 0.21502 | 0.76953 | 1.11110 |
Al | 0.40447 | 2.27913 | 4.02859 |
P | 0.01415 | 0.07306 | 0.08044 |
S | 0.53039 | 1.18803 | 0.49865 |
K | 0.19561 | 0.91432 | 1.12341 |
Ca | 1.93637 | 4.64651 | 5.08880 |
Fe | 0.99467 | 2.88129 | 2.90630 |
Cs | 0.00007 | 0.00032 | 0.00035 |
Ba | 0.01526 | 0.03768 | 0.01918 |
Sr | 0.00511 | 0.01754 | 0.01678 |
V | 0.00187 | 0.00640 | 0.00796 |
Cr | 0.00341 | 0.00821 | 0.00725 |
Mn | 0.01842 | 0.06016 | 0.05902 |
Co | 0.00039 | 0.00103 | 0.00136 |
Ni | 0.00571 | 0.00860 | 0.00276 |
Cu | 0.01473 | 0.02618 | 0.00464 |
Zn | 0.03199 | 0.06720 | 0.00913 |
Mo | 0.00054 | 0.00082 | 0.00007 |
Cd | 0.00018 | 0.00072 | 0.00008 |
W | 0.00037 | 0.00015 | 0.00002 |
Hg | 0.00001 | 0.00002 | 0.00001 |
Pb | 0.01259 | 0.03688 | 0.00253 |
Hf | 0.00003 | 0.00007 | 0.00008 |
As | 0.00029 | 0.00171 | 0.00080 |
Se | 0.00020 | 0.00038 | 0.00031 |
Sn | 0.00272 | 0.00424 | 0.00024 |
Sb | 0.00186 | 0.00345 | 0.00012 |
Bi | 0.00014 | 0.00027 | 0.00003 |
La | 0.00039 | 0.00175 | 0.00245 |
Th | 0.00009 | 0.00042 | 0.00058 |
U | 0.00004 | 0.00010 | 0.00009 |
Concentration, μg/m3 | |||
IAP RAS_BgAC | IAP RAS_HighAC | Kalmykia | |
Li | 0.00038 | 0.00188 | 0.00269 |
Na | 0.11048 | 0.33595 | 0.10146 |
Mg | 0.21502 | 0.76953 | 1.11110 |
Al | 0.40447 | 2.27913 | 4.02859 |
P | 0.01415 | 0.07306 | 0.08044 |
S | 0.53039 | 1.18803 | 0.49865 |
K | 0.19561 | 0.91432 | 1.12341 |
Ca | 1.93637 | 4.64651 | 5.08880 |
Fe | 0.99467 | 2.88129 | 2.90630 |
Cs | 0.00007 | 0.00032 | 0.00035 |
Ba | 0.01526 | 0.03768 | 0.01918 |
Sr | 0.00511 | 0.01754 | 0.01678 |
V | 0.00187 | 0.00640 | 0.00796 |
Cr | 0.00341 | 0.00821 | 0.00725 |
Mn | 0.01842 | 0.06016 | 0.05902 |
Co | 0.00039 | 0.00103 | 0.00136 |
Ni | 0.00571 | 0.00860 | 0.00276 |
Cu | 0.01473 | 0.02618 | 0.00464 |
Zn | 0.03199 | 0.06720 | 0.00913 |
Mo | 0.00054 | 0.00082 | 0.00007 |
Cd | 0.00018 | 0.00072 | 0.00008 |
W | 0.00037 | 0.00015 | 0.00002 |
Hg | 0.00001 | 0.00002 | 0.00001 |
Pb | 0.01259 | 0.03688 | 0.00253 |
Hf | 0.00003 | 0.00007 | 0.00008 |
As | 0.00029 | 0.00171 | 0.00080 |
Se | 0.00020 | 0.00038 | 0.00031 |
Sn | 0.00272 | 0.00424 | 0.00024 |
Sb | 0.00186 | 0.00345 | 0.00012 |
Bi | 0.00014 | 0.00027 | 0.00003 |
La | 0.00039 | 0.00175 | 0.00245 |
Th | 0.00009 | 0.00042 | 0.00058 |
U | 0.00004 | 0.00010 | 0.00009 |