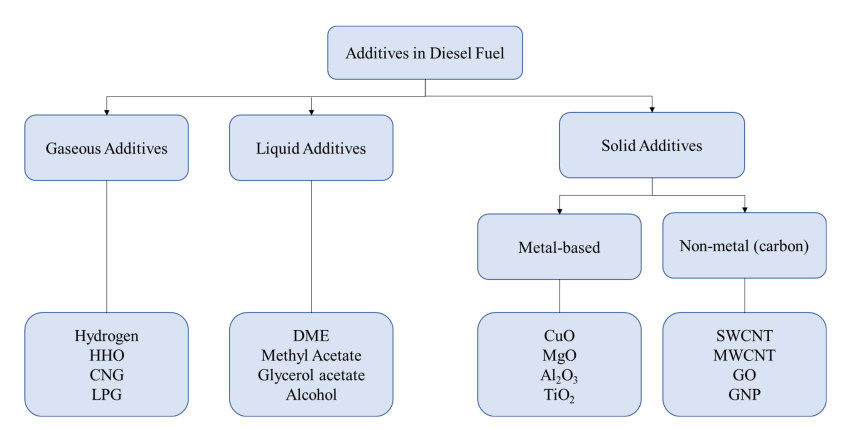
In recent years, there has been an increasing interest in additives for fuel research in the field of internal-combustion engines. Many studies have been conducted to improve the performance and emissions of the engine. Many kinds of additives in the form of solids, liquids, and gases have been used. The objective of this review is to examine the effects of having additives on the performance and emissions of an internal combustion engine. Additives such as alcohol, hydrogen, and metal oxides are proven to be successful in improving performance or reducing emissions. Results from selected papers are discussed and summarised in a table. With the new developments in nanotechnology, many researchers have shown an increased interest in carbon-based nanoparticles such as multi-walled carbon nanotubes (MWCNT) and single-walled carbon nanotubes (SWCNT). Lately, with the discovery of graphene production techniques, graphene nanoplatelets (GNP) have also been applied as fuel additives. In addition to understanding the effects of the additives on the engine performance and emissions, researchers extended the research to predict the outcome of the performance and emissions. The experiments involving the predictions efforts are summarised in a table. From the summary, it is found that the prediction of the GNP as fuel additive effects to the performance and emissions has not yet been explored. This gap is an opportunity for researchers to explore further.
Citation: Sarbani Daud, Mohd Adnin Hamidi, Rizalman Mamat. A review of fuel additives' effects and predictions on internal combustion engine performance and emissions[J]. AIMS Energy, 2022, 10(1): 1-22. doi: 10.3934/energy.2022001
[1] | Saad S. Alrwashdeh . Investigation of the effect of the injection pressure on the direct-ignition diesel engine performance. AIMS Energy, 2022, 10(2): 340-355. doi: 10.3934/energy.2022018 |
[2] | Sunbong Lee, Shaku Tei, Kunio Yoshikawa . Properties of chicken manure pyrolysis bio-oil blended with diesel and its combustion characteristics in RCEM, Rapid Compression and Expansion Machine. AIMS Energy, 2014, 2(3): 210-218. doi: 10.3934/energy.2014.3.210 |
[3] | Simona Silvia Merola, Luca Marchitto, Cinzia Tornatore, Gerardo Valentino . Spray-combustion process characterization in a common rail diesel engine fuelled with butanol-diesel blends by conventional methods and optical diagnostics. AIMS Energy, 2014, 2(2): 116-132. doi: 10.3934/energy.2014.2.116 |
[4] | Quoc Dang Tran, Thanh Nhu Nguyen, Vinh Nguyen Duy . Effect of piston geometry design and spark plug position on the engine performance and emission characteristics. AIMS Energy, 2023, 11(1): 156-170. doi: 10.3934/energy.2023009 |
[5] | Husam Al-Mashhadani, Sandun Fernando . Properties, performance, and applications of biofuel blends: a review. AIMS Energy, 2017, 5(4): 735-767. doi: 10.3934/energy.2017.4.735 |
[6] | Hussein A. Mahmood, Ali O. Al-Sulttani, Hayder A. Alrazen, Osam H. Attia . The impact of different compression ratios on emissions, and combustion characteristics of a biodiesel engine. AIMS Energy, 2024, 12(5): 924-945. doi: 10.3934/energy.2024043 |
[7] | Tien Duy Nguyen, Trung Tran Anh, Vinh Tran Quang, Huy Bui Nhat, Vinh Nguyen Duy . An experimental evaluation of engine performance and emissions characteristics of a modified direct injection diesel engine operated in RCCI mode. AIMS Energy, 2020, 8(6): 1069-1087. doi: 10.3934/energy.2020.6.1069 |
[8] | Thang Nguyen Minh, Hieu Pham Minh, Vinh Nguyen Duy . A review of internal combustion engines powered by renewable energy based on ethanol fuel and HCCI technology. AIMS Energy, 2022, 10(5): 1005-1025. doi: 10.3934/energy.2022046 |
[9] | Charalambos Chasos, George Karagiorgis, Chris Christodoulou . Technical and Feasibility Analysis of Gasoline and Natural Gas Fuelled Vehicles. AIMS Energy, 2014, 2(1): 71-88. doi: 10.3934/energy.2014.1.71 |
[10] | Nang Xuan Ho, Hoa Binh Pham, Vinh Nguyen Duy . Experimental study on characteristics of the test engine fueled by biodiesel based Jatropha oil and traditional diesel. AIMS Energy, 2020, 8(6): 1143-1155. doi: 10.3934/energy.2020.6.1143 |
In recent years, there has been an increasing interest in additives for fuel research in the field of internal-combustion engines. Many studies have been conducted to improve the performance and emissions of the engine. Many kinds of additives in the form of solids, liquids, and gases have been used. The objective of this review is to examine the effects of having additives on the performance and emissions of an internal combustion engine. Additives such as alcohol, hydrogen, and metal oxides are proven to be successful in improving performance or reducing emissions. Results from selected papers are discussed and summarised in a table. With the new developments in nanotechnology, many researchers have shown an increased interest in carbon-based nanoparticles such as multi-walled carbon nanotubes (MWCNT) and single-walled carbon nanotubes (SWCNT). Lately, with the discovery of graphene production techniques, graphene nanoplatelets (GNP) have also been applied as fuel additives. In addition to understanding the effects of the additives on the engine performance and emissions, researchers extended the research to predict the outcome of the performance and emissions. The experiments involving the predictions efforts are summarised in a table. From the summary, it is found that the prediction of the GNP as fuel additive effects to the performance and emissions has not yet been explored. This gap is an opportunity for researchers to explore further.
The internal combustion engine has become the foundation for vehicles, agriculture, and military operations as well as electricity generation. Acting as the heart of the vehicle, the internal combustion (IC) engine's function is to give the power that moves the vehicle. The engine itself is comprised of many elements and components, each of which has its own specific role. The primary component of the fuel is hydrocarbons. The composition and properties of it will determine its performance and emission results in the combustion process.
An internal combustion engine that runs on diesel fuel is known as a diesel engine. Diesel engines are commonly found in heavy-duty vehicles such as trucks, cars, and industrial transport that are used on the road. In comparison to S.I engines, C.I diesel engines have better fuel economy and reliability [1]. The excellent thermal efficiency of diesel engines is the primary reason for their vast range of applications, which include transportation, power generation, agricultural forms, and development domains [2].
In any case, compression ignition diesel engines emit massive volumes of pollutants (NOx, soot, CO, UHC, and particulate matter), posing serious health and environmental dangers [3]. The majority of these discharges result from non-ideal combustion cycles, such as fragmented fuel ignition, high temperature and pressing blending product responses, ignition of engine lubricating oil and additives, and burning of non-hydrocarbon diesel fuel components such as sulphur compounds and fuel added substances [4]. In terms of the environment, smoke emissions produced by diesel engines can cause both pollution of water and air, which significantly reduces visibility and contributes to global climate change [5]. It's a different scenario when it comes to health. Dizziness, headaches, and irritation of the eyes, nose, and mouth can occur after a brief exposure to high diesel exhaust emission levels [6]. Long-term exposure raises the risk of cardiovascular illness, cardiopulmonary disease, and respiratory disease, as well as lung cancer [7]. As a result of this, smoke emissions from diesel engines constitute a severe challenge that requires constant forecasting in order to keep them at a minimum, as well as other tactics such as fuel composition alteration, fuel additives, and other alternatives to manage smoke emissions [8].
Asia's rapid development has resulted in extremely high oil demand [9]. It is estimated that the world will consume 105 million barrels of oil per day in 2025 [10]. At this rate, the world is likely to run out of fossil fuels in the next 100 to 50 years [11]. Aside from that, the fuel price is extremely volatile [12]. Furthermore, fuel price fluctuations have a significant impact on economic, fiscal, and income patterns [13]. This is due to the fact that the fuel consumed in goods transportation has an impact on the price of goods [14].
With the conditions listed above, which include a mix of high emissions, high petroleum demand, volatile petroleum prices, and dwindling petroleum resources [15], it is well acknowledged that internal combustion engines must be more efficient [16]. To put it another way, the engine needs to consume less gasoline while producing more power [17]. The engine must also emit fewer pollutants. With these facts in mind, it's clear that we need to limit emissions from combustion engines [18]. As a result, a variety of studies have been done to improve performance and minimise emissions [19,20,21].
Alternative vehicle concepts such as electric vehicles and fuel cells are one way to cut emissions [22]. The less radical option involves employing a combustion engine but with alternative fuels such as biodiesel instead of pure diesel [23]. Another alternative is to add additives to the fuel that is currently being used [24]. The first application of fuel additives was to eliminate knocking in gasoline engines in the early twentieth century [25]. Combustion modifiers, antioxidants, corrosion inhibitors, and deposit control detergents are all examples of fuel additives [26]. Furthermore, gasoline additives can be utilised for a variety of applications, including octane boosters, fuel injector cleaners, and fuel line antifreezes [27]. This study aims to assess the current state of fuel additives in diesel and gasoline engines. In addition to observing the impacts, the study's scope has been expanded to include predicting the outcomes of additive effects on engine performance and emissions.
Currently, the transportation sector is primarily reliant on internal combustion engines, such as diesel and spark-ignition engines. Combustion engines are likely to remain the most important engine for the next 30 years. Every year, the global transportation sector consumes more and more energy. The use of fossil fuels is a major contribution to this fact. Internal combustion engines that run on fossil fuels provide a lot of power to the transportation sector. Despite advancements in alternative fuels, around 90% of people still use traditional fossil fuels, with only a small percentage using renewable fuels [28]. The demand for transportation, industrial, and research services is increasing every day as the world's population grows, resulting in a petroleum fuel shortage. The rapid depletion rate of petroleum fuel, as well as the impact of its emission gases on the environment, has become a major issue for people all over the world.
The literature suggested some popular additives that are in use. Hydrogen [29], CNG [30], LPG, ZnO [31], alcohols, methyl-esters [32], Mn2O3, Al2O3 [33], MWCNT [34], GO, and GNP are the latest additions to the list of materials used as additives. These additives can be classified into gaseous additives, liquid additives, and solid additives. These solid additives can be divided into metal and non-metal additives. This classification can be represented in Figure 1 below:
To understand the subject, the properties of the fuel and additives are observed in detail. The properties of the base fuel (diesel or biodiesel) can be summarised in Table 1, while the properties of gaseous and liquid additives are presented in Table 2.
![]() |
CV [MJ/kg] | Density [kg/m3] | Kinematic Viscosity [mm2/s] | Flash point [℃] |
pure diesel | 44 | 825.96 | 3.96 | 72 |
Biodiesel Chlorella Vulgaris | 38.7 | 860 | 3.7 | 124 |
honge biodiesel HB20 | 42.123 | 835 | 4.12 | 104 |
![]() |
CV [MJ/kg] | Density [kg/m3] | Kinematic Viscosity [mm2/s] |
Flash point [℃] |
hydrogen | 119.93 | 0.0838 | 110 | - |
methanol | 20.30 | 790 | 0.59 | 12 |
ethanol | 27 | 785 | 1.1 | 14 |
CNG | 45.76 | 720 | - | 81.6 |
The carbon-based additives used are carbon nanotubes and graphene. The properties of the materials as in the Table 3 below:
![]() |
CV [MJ/kg] | Density [g/cm3] | Specific Surface Area (m2g-1) | Thermal conductivity (kW/mK) |
SWCNT | 1.3–1.4 | 1315 | 1750–3800 | |
Graphene | 31.82 | 2.267 | 1598 | 5300 |
Many parameters can be used to measure the performance and emissions of a diesel engine. However, after researching many articles in the field, standard parameters used to evaluate engine performance are brake torque, brake power, brake specific fuel consumption (BSFC), and brake thermal efficiency (BTE). Additionally, the emission parameters being observed are carbon monoxide (CO), carbon dioxide (CO2), nitrogen oxides (NOx), and unburned hydrocarbons (HC).
In a study to determine the effect of hydrogen on diesel-biodiesel blend, Tayari [37] added different amounts of hydrogen mixtures to the engine. It was found that hydrogen improved the power performance. Torques in B20H10 and B10H10 were 10.6% and 8.4% higher than the biodiesel blends without hydrogen induction (B20H0 and B10H0). The engine's low power and torque are caused by biodiesel's low heating value and high viscosity. Additional hydrogen to biodiesel improved the power and torque of the engine due to the faster rate of combustion caused by hydrogen. In another research by Ngang [38], the authors conclude that the torque of the diesel-LPG engine increases as the quantity of LPG fuel also increases. The findings strongly support the directly proportional relationship between the amount of LPG fuel introduced and the output torque of the diesel-LPG engine. The power output in the diesel-LPG mode of operation can be controlled by the amount of LPG introduced to the engine. Generally, increasing the LPG mass fraction yields more power. For example, the power of 60% LPG is higher than the power of 50% LPG.
In an experiment by Surya [29], hydrogen enrichment of biodiesel was observed. The fuel used was a honge biodiesel blend (HB20). The hydrogen enrichment was between 10–13 l/min. H-B20-A (Hydrogen 5 l/min + B20 + Al2O3 produced higher power of 6.4%. This combination also reduced specific fuel consumption by 2.72%. In a study to determine the effect of hydrogen on diesel-biodiesel blend, Tayari [37] added different amounts of hydrogen mixtures to the engine. It was found that hydrogen improved the BSFC performance. The average reductions of BSFC for B20H5 and B10H5 were 3.4% and 5.1% compared to the biodiesel blends without hydrogen (B20H0 and B10H0). Biodiesel with hydrogen led to more power generation and increased the combustion efficiency of the engine due to high calorific value and mass flow rate of hydrogen. An example experiment by Akcay [39] indicated that hydrogen addition reduced the BSFC. The result showed With H40 (hydrogen induction of 40 l/min), the BSFCs of diesel fuel tests were decreased by 11.1%, 12.5%, and 7.9%, respectively, for the engine loads of 40, 60, and 80 Nm, as compared with the pure diesel fuel tests. It is seen that BSFC generally exhibits a decrease with the increasing load for each fuel and hydrogen addition. As a result of the increase in the cylinder wall temperatures, the decrease in the ignition delay and, accordingly, the progress of the combustion, BSFCs showed decreasing tendencies [40]. For example, in an experiment using spark-ignition engine, Yilmaz [41] found that the BSFC of alcohol (methanol and ethanol) had been reduced after the induction of hydrogen. The addition of hydrogen reduced the BSFC by around 4% compared to the alcohol-gasoline blend. This is because the lower heating value of hydrogen is very high compared to gasoline. Hydrogen addition increases thermal efficiency and decreases BSFC [42].
The effect of hydrogen addition on thermal efficiency was carried out by Yilmaz [41] in an experiment with alcohol-gasoline in a spark-ignition engine. It was found that the BTE increased with the hydrogen addition. Experimentally, Gnanamoorthi [43] also shows a better BTE (increased by 30.65%) with the addition of hydrogen. Another finding from Tabar [44] is that the BMEP of CNG is 4–14% less than that of gasoline throughout all the engine speeds. CNG has a 10% lower indicated power. When compared to gasoline, CNG has a remarkable 11–39% lower fuel consumption [44]. Sonachalam [45] demonstrated that the brake thermal efficiency (BTE) is increased by about 3.7% at a flow rate of 4 liters per minute (LPM) of acetylene injection in RCCI combustion mode.
An analysis of fuel additive effects on engine emissions was carried out by Gnanamoorthi [43], and it indicated that the emissions of HC, CO, and CO2 were reduced by 22.3%, 14% and 32.74% respectively with the addition of hydrogen. Similarly, another experiment by Selahaddin Orhan Akansu [46] pointed out that hydrogen produced fewer emissions, especially CO, CO2, and HC. This happens because of the chemical composition of hydrogen, which does not have carbon and has high thermal efficiency. However, the emission of NOx increased. The reason for that is the high temperature of the combustion. As argued by Yilmaz [41], the NOx emission has been reduced when hydrogen is introduced into the combustion. The reason for that is the low adiabatic flame temperature of the methanol. The addition of hydrogen by Surya [29] was successful in reducing the CO by 15.91%. However, this experiment produced higher CO2 and NOx by 22.37% and 37.68% respectively.
It was also reported by Yilmaz [41] that CO has been reduced with the addition of hydrogen. The fuel with the lowest CO emission was found to be G85M15H15, with a CO emission of 0.094%. On the other hand, CO2 has been reduced with the addition of hydrogen. The lowest CO2 emission was found to be 12.06% for G85M15H15 fuel. Another example, Sonachalam [45] found that acetylene addition to a diesel engine running with biodiesel shows significant decreases in polluting components such as smoke opacity, oxides of nitrogen (NOx), hydrocarbons (HC) and carbon monoxide (CO) are reduced by about 10%, 7.6%, 13.4% and 28.7%. As reported by [38], LPG addition reduced NOx and HC emission pollutants, despite the fact that the overall in-cylinder temperature increased. This demonstrates that adding LPG to a specific value allows for decreased emissions as long as the mixture remains lean. The ensuing increase in temperature and pressure is compensated for by the mixture becoming richer, cutting emissions and enhancing combustion efficiency.
Figure 2 is a bar chart depicting the various effects of gaseous fuel additives on engine emissions. In the graphic, the percentage value of hydrogen, CNG, and acetylene additions are compared to their base fuels, which are gasoline-ethanol blend, gasoline, and biodiesel, respectively.
Several studies have been undertaken to investigate the impact of liquid additives on engine performance and emissions. Najafi [47] conducted an experiment to estimate the performance and exhaust emissions of a four-cylinder SI engine. The engine operates on the different mixing ratios of ethanol and gasoline blends. A mixture of gasoline and bioethanol derived from potato peels has been employed. 0%, 5%, 10%, 15%, and 20% have been employed. The mixture was called E0, E5, E10, E15, and E20. The engine performance and emissions from the mixture have been compared with the performance and emissions from 100% gasoline. The experiment found that using ethanol blends improved brake power, brake thermal efficiency (BTE), engine torque, and volumetric efficiency. On the other hand, the brake specific fuel consumption (BSFC) decreased. BSFC is defined as the energy consumed per unit power produced considering the calorific value of the fuel. Mohamed Nour [48] identifies the effects of pentanol/hydrous ethanol/diesel blend and octanol/hydrous ethanol/diesel have on compressed-ignition engine. The finding suggests that the addition of octanol to the hydrous ethanol/diesel blends achieves the lower BSFC at all loads.
A deeper investigation by Yesilyurt [49] was performed to compare the engine performance, combustion, and emissions of direct-injected diesel engines fuelled with diesel, with diesel-butanol blend, and diesel-pentanol blend. Yesilyurt reported that the blended fuels produced lower EGT and CO2. However, more O2 is produced. The reason for the observation was the higher oxygen content in the alcohol.
As highlighted by Godwin [50], gasoline blended with alcohol produced lower EGT compared to 100% gasoline. This is because gasoline has the highest calorific value and the lowest latent heat of vaporization, lean mixture formation, and flame propagation. This leads to an increase in cylinder temperature and more gasoline being used.
Another experiment using alcohol was conducted by S. Phuangwongtrakul [51] to study the engine performance. Brake torque and brake specific fuel consumption while a different mixing ratio of ethanol was investigated. The experiment proved that a proper ethanol-gasoline mixing ratio can improve engine torque output while reducing emissions. By adding pentanol to biodiesel, Yesilyurt [52] found the maximum BTE values of the diesel fuel and B20, and B20P5 were found to be at 22.75%, 21.82%, and 20.96% under 750 W engine load condition, respectively. This little change, however, can be considered insignificant.
From the experiment by Najafi [47], the result showed that the amount of CO and HC had been reduced, but CO2 and NOx emissions were higher. This is supported by Dogan in 2017 [53] to identify irreversible processes using energy and exergy analyses. The fuels were blended in various proportions. E0 refers to 100 percent unleaded gasoline, while E10 refers to gasoline blended with 10% ethanol. E20 is ethanol with a concentration of 20%, and E30 is ethanol with a concentration of 30%. The fuel blends were then tested in gasoline engines at various speeds, loads, and under identical conditions for each fuel type. NOx levels were reduced as a result of the experiments and theoretical calculations.
One of the new exploratory investigations by Yesilyurt [49] is the exhaust emission characteristics of a solitary chamber, direct-infusion diesel motor running on biodiesel/diesel/1-butanol and biodiesel/diesel/n-pentanol fuel blends. Yesilyurt found that alcohol blended fuels reduced NOx by 0.56%–2.65%, CO by 6.90%–32.40%, and smoke by 10.47%–44.33%. The authors expressed that higher alcohols, particularly n-pentanol, could be an efficient additive to diminish NOx emissions by thinking about the acquired outcomes.
In another major study, Uslu and Celik [54] utilised diethyl ether (DEE) blends with pure diesel to study the exhaust emission characteristics of a single-cylinder, direct-injection, and air-cooled diesel engine. An experiment was carried out at four engine speeds ranging from 1000 to 1600 RPM with 200 RPM intervals and four engine loads, which were 150,300,500, and 1000-watt halogen lamps. Diesel fuel was mixed with DEE0, DEE2.5, DEE5, DEE7.5, and DEE10, which contained 2.5%, 5%, 7.5%, and 10% by volume of DEE, respectively. According to the author's findings, DEE10 allows NOx emissions to be reduced by 56%.
Another experiment was conducted by Dogan [53] to identify irreversible processes using energy and exergy analyses. The fuels were blended at different ratios. 100% unleaded gasoline is called E0, and gasoline blended with 10% ethanol is called E10. 20% ethanol is called E20 and 30% ethanol is called E30. The fuel blends then applied into gasoline engine with different speeds, under different loads and under the same conditions for each fuel type. The result from the experiments and theoretical calculations showed a reduction in carbon monoxide (CO).
In another major study done by Uslu and Celik [54], it was identified that DEE10 allows NOx emissions to be reduced by 56% compared with diesel fuel, while CO emissions are reduced by about 45%. Dogan [53] conducted another experiment that focused on identifying irreversible processes using energy and exergy analyses. The fuels were mixed in various proportions. E0 refers to 100 percent unleaded gasoline, while E10 refers to gasoline blended with 10% ethanol. E20 is ethanol with a concentration of 20%, and E30 is ethanol with a concentration of 30%. The fuel blends are then applied to gasoline engines at varying speeds, under varying loads, and under the same conditions for each fuel type. Carbon dioxide levels were reduced as a result of the experiments and theoretical calculations (CO2).
In the same vein, the experiments by Chao Chen [55] and Pinzi [56] found that the diesel/alcohol blend was able to reduce soot formation. This phenomenon might happen due to the high oxygen content in alcohol that helps in the burning of soot during combustion. A bar chart is plotted in Figure 3 to visualise the different effects of the liquid fuel additives on the engine emissions.
Solid fuel additives are another important type of additive. Amirabedi [57] went above and beyond by incorporating Mn2O3 into the gasoline-alcohol mixture. She used gasoline that had been diluted with 10% ethanol. She then added 10 ppm and 20 ppm Mn2O3 as additional samples. The outcomes were very promising. In terms of efficiency, performance has improved. This is supported by Jayaraman's [58] findings, which investigate the effects of adding titanium oxide TiO2 to a B20 blend in a CI engine. As a result, specific consumption has declined. At 25% load, B20 + 50 produces the lowest BSFC of 0.46 kg/kw-hr. At 18.16 kg load, the maximum BTE for B20 + 50 + EGR was 32.64 percent. The BTE of B20 with TiO2 is higher than that of normal B20 because the TiO2 improved combustion and released a high heat rate.
Jiangjun Wei [59] investigated the emission of CI engine operation with diesel-methanol blends using Aluminium Oxide Al2O3 as an additive. Among the highlights are reductions in BTE and BSFC of 3.6 and 3.7 percent, respectively. According to Örs [60], adding TiO2 to biodiesel-butanol blends increased brake engine torque and power by 10.20 percent and 9.74 percent, respectively. BSFC, on the other hand, can be reduced by 27.73%. Amirabedi [57] discovered that the performance in terms of power and efficiency has improved. This finding is supported by Soner's findings [61]. In his experiment, adding 50 ppm of Al2O3 and CuO to diesel fuel resulted in increased engine power.
The research by Örs [60] showed that CO and HC have been reduced by around 14.96% and 34.39%, respectively. with the TiO2 addition. However, the emission of CO2 and NO was increased by 5.75% and 3.94%, respectively. Research by Amirabedi [57] achieved a 51.83% reduction of HC by using gasoline with 10% ethanol and 20 ppm Mn2O3 compared to that of baseline gasoline. This is achieved when the engine runs at full load and the speed is set at 2800 rpm. The CO was reduced by 24.09% with respect to the baseline gasoline fuel. However, CO2 emissions increased by 13.27% in this experiment. Using aluminium oxide (Al2O3) as an additive, Jiangjun Wei [62] tested the emissions of a CI engine run with diesel-methanol blends. The results were lower CO and HC of 83.3% and 40.9%. However, the engine emitted a marginally higher NOX of 14.4%. A bar chart is plotted in Figure 4 to visualise the different effects of the solid fuel additives on the engine's emissions.
The section that follows discusses carbon-based fuel additives. This review focuses on carbon nanotubes and graphene. Heydari-Melaney [63] improved the performance of biodiesel-bioethanol mixtures by adding carbon nanotubes (CNT) and increasing torque, power, and BTE by 15.52%, 15.52%, and 13.97%, respectively. BSFC, on the other hand, fell 11.73%. Similarly, Hosseini [64] used CNT as an additive in a CI engine that was powered by a diesel-biodiesel blend. The procedure for the experiment was carried out under full load with various blends and speeds. In his experiment, he discovered that the addition of CNT produced more torque than pure diesel fuel. He also discovered that power increased by 21.84% overall. BTE, on the other hand, was discovered to be decreasing from 1800 to 2800 rpm. This was caused by a decrease in volumetric efficiency. The addition of CNT reduced BSFC as well.
Gad [65] used carbon nanotubes and graphene nanosheets as fuel additives in B20 blends. CNTs and graphene nanosheets are mixed into B20 at concentrations of 25, 50, and 100 ppm to create fuel blends. Thermal efficiency for B20CNT100 and B20CNS100 improved by 8% and 19%, respectively, according to the results. In another significant study, Razzaq [66] investigated the use of graphene and dimethyl carbonate (DMC) as additives in biodiesel. The goal is to investigate the effects on performance and emissions. According to his observations, the BTE increased with a small amount of GNP. However, increasing the amount reduces the BTE. The reason for this could be due to the higher viscosity.
In Hosseini's experiment [64], he discovered that under various conditions, the emission of a fuel blend with CNT additives was reduced by 40.38 percent when compared to neat diesel. In the case of CO2, the average emission increased by 9.88 percent when all fuel blends with CNTs were compared to straight diesel fuel. The HC emissions of a fuel blend containing a CNT additive were reduced in general. In Gad's experiment [65], extremely large reductions in smoke thickness, CO, NOx, HC, and with B20CNT100 are observed, which are 28 percent, 27 percent, 22 percent, and 28 percent, respectively. For smoke thickness, CO, NOx, and HC, B20CNS100 obtained 54%, 47%, 44%, and 52%, respectively.
Tomar and Kumar [67] conducted a similar investigation using a single-cylinder four-stroke diesel engine (Kirloskar) and diesel-Schleichera Oleosa Biodiesel mixes with alumina nanoparticles and multi-walled carbon nanotubes. Based on their findings, the authors believe that the use of alumina and MWCNT-added substances could be an effective fuel reformulation strategy. NOx emissions decrease as a result of the presence of nano-added substances in D80B20C100 fuel, owing to the amazing spongy inclination of MWCNT nanoparticles.
Mei et al. [68] proposed a single-cylinder four-stroke air-cooled common-rail diesel engine operating at 3000 rpm with changing loads of 100%, 75%, 50%, and 25%. The authors used two different nanoparticles, CNTs and molybdenum trioxide (MoO3), and mixed them with clean diesel at concentrations of 50 mg/L and 100 mg/L for each nanoparticle. According to the authors, CNTs have a greater potential than MoO3 to reduce the entire estimated exhaust emission. CO, HC, NOx, and smoke discharge from CNT100 were reduced by 9.6%, 11.4%, 8.9%, and 15.25%, respectively, when compared to the exhaust emission produced by Mo100, which is 6.8%, 7.5%, 5.2%, and 8.3%. The authors also stated that the results could be attributed to CNTs' superior heat conductivity and surface deficiency when compared to MoO3.
In Heydari-Melaney [63] experiment where CNT was mixed into biodiesel-bioethanol mixtures, The CO and UHC reduced by 5.47% and 31.72%, respectively. However, NOx emission increased by 12.22%. According to Razzaq [66], an increase in HC emission was observed compared to that of B30 with the addition of GNPs in biodiesel blends. However, if the concentration in GNP is increased further, the HC emissions are reduced significantly. The CO emission was also increased with the increasing engine speeds. This is valid for all tested blends. The reason for this observation is caused by the difference in air-fuel quantity entering the combustion chamber for different engine operating conditions. Normally, NOx emissions contributed to the high temperature in the cylinder. The high temperature oxidized the nitrogen which is the main component of air sucked by the engine. In Razzaq's experiment, the addition of GNPs in biodiesel blends reduced the NOx emission. This happens because GNP's presence improved the ID period.
Nivin Chacko [69] used GNP in an experiment that also showed a reduction in emissions, particularly NO. El-Seesy [70] discovered a reduction in emissions after using graphene nanoplatelets as an additive in jatropha biodiesel-diesel fuel. NOx, CO, and UHC emissions were reduced by 45 percent, 55 percent, and 50 percent, respectively. Another study by Debbarma discovered that adding 75 ppm of GNP to biodiesel improved engine performance, particularly BTE, BSFC, and combustion behaviour, especially at higher loads [71]. Figure 5 depicts a bar chart that depicts the various effects of carbon-based fuel additives on engine emissions.
In addition, a table to summarise the results from different experiments is depicted in Table 4 below:
Gaseous Additives | ||||||
Ref. | Engine | Fuel | Additive | Method | Performance | Emissions |
[29] | 4-cylinder Diesel | B20 | Al2O3 + H2 | Full load between 1200–2800 rpm | Power ↑ BTE ↑ BSFC ↓ |
CO ↓ CO2 ↑ NOx ↑ |
[37] | 1-cylinder Diesel | B20 |
H2 | Full load. Various speed |
Torque ↑ Power ↑ BTE ↑ |
CO ↓ CO2 ↓ HC ↓ NOx ↑ |
[43] | 1-cylinder Diesel | Diesel | H2 | Constant speed 1500 rpm | BTE ↑ |
CO ↓ CO2 ↓ HC ↓ NOx ↑ |
[45] | 1-cylinder Diesel | Biodiesel | Acetylene | 1500 rpm Different loads |
BTE ↑ |
CO ↓ HC ↓ NOx ↓ |
[38] | 4-cylinder diesel | diesel | LPG | different Speeds and loads | Torque ↑ Power ↑ |
HC ↓ NOx ↓ |
Liquid Additives | ||||||
Ref. | Engine | Fuel | Additive | Method | Performance | Emissions |
[48] | 1-cylinder Diesel | Hydrous ethanol-Diesel | Pentanol and Octanol | Different blends at different loads | Power ↓ BTE ↑ BSFC ↑ |
CO ↓ CO2 ↓ NOx ↓ |
[72] | 1-cylinder Diesel | Diesel | Alcohol | Constant speed 2500 rpm. Different alcohol percentage. | BTE ↓ |
CO ↓ HC ↑ NOx ↑ |
[52] | 1-cylinder Diesel | Biodiesel | Pentanol | 3000 rpm Different engine loads |
BSFC ↑ BTE ↓ |
CO ↓ CO2 ↑ NOx ↓ |
Solid Additives (Metal-based) | ||||||
Ref. | Engine | Fuel | Additive | Method | Performance | Emissions |
[73] | 1-cylinder Diesel |
biodiesel | CuO | Various loads | BTE ↑ BSFC ↓ |
CO ↓ HC ↓ NOx ↑ |
[62] | 1-cylinder Diesel |
Diesel-methanol | Al2O3 | Constant speed 1400 rpm. Different loads | BTE ↑ BSFC ↓ |
CO ↓ HC ↓ NOx ↑ |
[60] | 1-cylinder Diesel |
Waste Cooking Biodiesel | TiO2 | Various engine speeds. Full load | Torque ↑ Power ↑ BSFC ↓ |
CO ↓ CO2 ↑ HC ↓ NO ↑ |
Solid Additives (Carbon-based) | ||||||
Ref. | Engine | Fuel | Additive | Method | Performance | Emissions |
[63] | 1-cylinder Diesel | Diesohol (diesel + alcohol) | CNT | Full load at different rpm | Torque ↑ Power ↑ BTE ↑ BSFC ↓ |
CO ↓ CO2 ↓ HC ↓ NOx ↑ |
[67] | 1-cylinder Diesel | Diesel & Diesel-biodiesel blend | MWCNT | Constant speed 1500 rpm. Different loads | BTE ↑ BSFC ↓ |
CO ↓ NOX↓ |
[64] | 1-cylinder Diesel | Diesel-biodiesel blend | CNT | Full load. Different blends and speed | Power ↑ Torque ↑ BTE ↓ BSFC ↓ |
CO ↓ CO2 ↑ HC ↓ NOX ↑ |
[66] | 1-cylinder Diesel | Palm oil biodiesel | Graphene Oxide Nanoplatelets (GNP) | Varying engine speed. Different blends | BTE ↑ BSFC ↑ |
HC ↓ CO ↑ NOX↓ |
[74] | 1-cylinder Diesel | Jatropha biodiesel | Graphene Nanoplatelets (GNP) | Different Speeds, different loads, different fuel blends | BSFC ↓ | CO ↓ HC ↓ NOX ↓ |
Further, the study extends to the prediction of the effects on the performance and emissions of a diesel engine. The articles with predictions or simulations of the effects are being studied. Different research articles with additional predictions on the additive effects on the performance and emissions are selected. The following is a table summary of the effects that additives have on performance and emissions.
In addition to understanding the effects the additive has on the fuels, the researchers go on further to predict the engine performance and emissions. The most popular method to predict the response of different factors in a study is called response surface methodology (RSM).
According to Table 5, no prediction research has been conducted on the effects of graphene additives on the performance and emissions of diesel engines. As a result, this is an opportunity for research to fill this gap.
No | Base fuel | Additives/blends | Parameters | Prediction Method | Software/Tool | Reference |
Liquid Additives | ||||||
1 | Waste plastic oil | ethanol | HC, CO, SFC | DOE with Full factorial design | Minitab | [75] |
2 | diesel | DME | BTE, BSEC | Double Weibe | - | [76] |
3 | diesel | higher alcohol additive (Decanol) | BSFC, BTE, CO, CO2, HC, NOx | ANN and RSM | Design Expert | [77] |
4 | diesel | pongamia methyl esters | BTE, UHC, NOx | RSM | Minitab | [78] |
Gaseous Additives | ||||||
5 | diesel | CNG | BSFC, BTE, CO, CO2, HC, NOx | RSM | Minitab and Design Expert | [35] |
6 | diesel | LPG | BSEC, BTE | Fuzzy Logic | ANFIS | [79] |
7 | diesel | Hydrogen |
CO, CO2, HC, NOx | DOE with Taguchi | Minitab | [80] |
Solid Additives (Metal) | ||||||
8 | biodiesel | NiO | BSFC NOx, HC, CO |
RSM | Design Expert | [81] |
9 | biodiesel | Alumina nanoparticles | BSFC, BTE, CO, NOx | RSM | Design Expert | [82] |
10 | biodiesel | TiO2, and Al2O3 nanoparticles | BSFC, BTE, CO, NOx | RSM | Design Expert | [83] |
Solid Additives (Carbon based) | ||||||
11 | diesel and biodiesel | MWCNT | BSFC, CO, NOx | RSM | Design Expert | [83] |
12 | diesel and biodiesel | MWCNT | BTE, BSFC, UHC, CO, NOx | RSM | Design Expert | [84] |
13 | fusel oil | nano- biochar | Torque, Power, BSFC, BTE, CO, NOx | RSM | Design Expert | [85] |
According to the review, many different types of fuel additives have been developed. Many additives were available in gaseous, liquid, and solid forms. Many solid materials are being considered as fuel additives as nanotechnology advances. Carbon-based nanomaterials have shown a lot of promise in terms of improved performance and lower emissions. New graphene materials have also been discovered and added to the list of additives; however, the depth of research is still insufficient. More information on various types of engines and configurations would aid us in establishing greater accuracy on this subject.
The financial assistance and laboratory use provided by the RACER Grant, RDU192617, through the Automotive Engineering Centre, Universiti Malaysia Pahang is gratefully acknowledged.
The authors declare no conflicts.
[1] |
Raghuvaran S, Ashok B, Veluchamy B, et al. (2020) Evaluation of performance and exhaust emission of C.I diesel engine fuel with palm oil biodiesel using an artificial neural network. Mater Today: Proc 37: 1107-1111. https://doi.org/10.1016/j.matpr.2020.06.344 doi: 10.1016/j.matpr.2020.06.344
![]() |
[2] |
Sunil Kumar M, Rajasekar R, Ganesan S, et al. (2020) Evaluation of metal oxide nano particles in lemongrass biodiesel for engine performance, emission and combustion characteristics. Mater Today: Proc 44: 3657-3665. https://doi.org/10.1016/j.matpr.2020.10.796. doi: 10.1016/j.matpr.2020.10.796
![]() |
[3] |
El-Seesy AI, Hassan H, Ookawara S (2018) Performance, combustion, and emission characteristics of a diesel engine fueled with Jatropha methyl ester and graphene oxide additives. Energy Convers Manage 166: 674-686. https://doi.org/10.1016/j.enconman.2018.04.049 doi: 10.1016/j.enconman.2018.04.049
![]() |
[4] |
Ershov MA, Grigorieva EV, Abdellatief TM, et al. (2021) Hybrid low-carbon high-octane oxygenated gasoline based on low-octane hydrocarbon fractions. Sci Total Environ 756: 142715. https://doi.org/10.1016/j.scitotenv.2020.142715 doi: 10.1016/j.scitotenv.2020.142715
![]() |
[5] |
Sharma P, Dhar A (2019) Particulate emissions from hydrogen diesel fuelled CI engines. Engine Exhaust Part, 199-211. https://doi.org/10.1007/978-981-13-3299-9_10 doi: 10.1007/978-981-13-3299-9_10
![]() |
[6] |
Chuvashev A, Chuprakov A (2019) Investigation of environmental indicators of diesel engine when working on methanol. J Phys: Conference Series 1399: 055085. https://doi.org/10.1088/1742-6596/1399/5/055085 doi: 10.1088/1742-6596/1399/5/055085
![]() |
[7] |
Wong JY, Vermeulen R, Dai Y, et al. (2021) Elevated urinary mutagenicity among those exposed to bituminous coal combustion emissions or diesel engine exhaust. Environ Mol Mutagen 62: 458-470. https://doi.org/10.1002/em.22455 doi: 10.1002/em.22455
![]() |
[8] |
Jeevahan J, Mageshwaran G, Joseph GB, et al. (2017) Various strategies for reducing Nox emissions of biodiesel fuel used in conventional diesel engines: A review. Chem Eng Commun 204: 1202-1223. https://doi.org/10.1080/00986445.2017.1353500 doi: 10.1080/00986445.2017.1353500
![]() |
[9] |
Day C, Day G (2017) Climate change, fossil fuel prices and depletion: The rationale for a falling export tax. Econ Modell 63: 153-160. https://doi.org/10.1016/j.econmod.2017.01.006 doi: 10.1016/j.econmod.2017.01.006
![]() |
[10] | Kaplan RS (2018) A Perspective on Oil, in: Federal Reserve Bank of Dallas. |
[11] |
Raugei M, Leccisi E (2016) A comprehensive assessment of the energy performance of the full range of electricity generation technologies deployed in the United Kingdom. Energy Policy 90: 46-59. https://doi.org/10.1016/j.enpol.2015.12.011 doi: 10.1016/j.enpol.2015.12.011
![]() |
[12] |
Mattioli G, Philips I, Anable J, et al. (2019) Vulnerability to motor fuel price increases: Socio-spatial patterns in England. J Trans Geogr 78: 98-114. https://doi.org/10.1016/j.jtrangeo.2019.05.009 doi: 10.1016/j.jtrangeo.2019.05.009
![]() |
[13] |
Kpodar K, Abdallah C (2017) Dynamic fuel price pass-through: Evidence from a new global retail fuel price database. Energy Econ 66: 303-312. https://doi.org/10.1016/j.eneco.2017.06.017 doi: 10.1016/j.eneco.2017.06.017
![]() |
[14] |
Cavalcanti EJ, Carvalho M, Ochoa AA (2019) Exergoeconomic and exergoenvironmental comparison of diesel-biodiesel blends in a direct injection engine at variable loads. Energy Convers Manage 183: 450-461. https://doi.org/10.1016/j.enconman.2018.12.113 doi: 10.1016/j.enconman.2018.12.113
![]() |
[15] |
Zahan KA, Kano M (2018) Biodiesel production from palm oil, its by-products, and mill effluent: A review. Energies 11: 2132. https://doi.org/10.3390/en11082132 doi: 10.3390/en11082132
![]() |
[16] |
Han J, Vahidi A, Sciarretta A (2019) Fundamentals of energy efficient driving for combustion engine and electric vehicles: An optimal control perspective. Automatica 103: 558-572. https://doi.org/10.1016/j.automatica.2019.02.031 doi: 10.1016/j.automatica.2019.02.031
![]() |
[17] |
Krishania N, Rajak U, Verma TN, et al. (2020) Effect of microalgae, tyre pyrolysis oil and Jatropha biodiesel enriched with diesel fuel on performance and emission characteristics of CI engine. Fuel 278: 118252. https://doi.org/10.1016/j.fuel.2020.118252 doi: 10.1016/j.fuel.2020.118252
![]() |
[18] |
Krishnamoorthi M, Malayalamurthi R, He Z, et al. (2019) A review on low temperature combustion engines: Performance, combustion and emission characteristics. Renewable Sustainable Energy Rev 116: 109404. https://doi.org/10.1016/j.rser.2019.109404 doi: 10.1016/j.rser.2019.109404
![]() |
[19] |
Mohiddin AASMN, Reddy ANR, Hamdan S (2018) A study on chicken fat as an alternative feedstock: Biodiesel production, fuel characterisation, and diesel engine performance analysis. Int J Automot Mech Eng 15. https://doi.org/10.15282/ijame.15.3.2018.10.0425 doi: 10.15282/ijame.15.3.2018.10.0425
![]() |
[20] |
Nayak SK, Mishra PC (2017) Analysis of a Diesel Engine fuelled with jojoba blend and coir pith producer gas. Int J Automot Mech Eng 14: 4675-4689. https://doi.org/10.15282/ijame.14.4.2017.7.0368 doi: 10.15282/ijame.14.4.2017.7.0368
![]() |
[21] |
Pattanaik BP, Jena J, Misra RD (2017) The effect of oxygen content is soapnut biodiesel-diesel blends on performance of a diesel engine. Int J Automot Mech Eng 14: 4574-4588. https://doi.org/10.15282/ijame.14.3.2017.14.0361 doi: 10.15282/ijame.14.3.2017.14.0361
![]() |
[22] |
Cano ZP, Banham D, Ye S, et al. (2018) Batteries and fuel cells for emerging electric vehicle markets. Nat Energy 3: 279-289. https://doi.org/10.1038/s41560-018-0108-1 doi: 10.1038/s41560-018-0108-1
![]() |
[23] |
Anderson A, Al-Mohaimeed AM, Elshikh MS, et al. (2021) Exergy and Energy Analysis of α-Fe2O3-Doped Al2O3 Nanocatalyst-Based biodiesel blends—Performance and emission characteristics. J Energy Resour Technol 143. https://doi.org/10.1115/1.4050488 doi: 10.1115/1.4050488
![]() |
[24] |
Ge S, Ganesan R, Sekar M, et al. (2022) Blending and emission characteristics of biogasoline produced using CaO/SBA-15 catalyst by cracking used cooking oil. Fuel 307: 121861. https://doi.org/10.1016/j.fuel.2021.121861 doi: 10.1016/j.fuel.2021.121861
![]() |
[25] |
Nadim F, Zack P, Hoag GE, et al. (2001) United States experience with gasoline additives. Energy Policy 29: 1-5. https://doi.org/10.1016/S0301-4215(00)00099-9 doi: 10.1016/S0301-4215(00)00099-9
![]() |
[26] |
Ershov MA, Savelenko VD, Makhova UA, et al. (2021) New insights on introducing modern multifunctional additives into motor gasoline. Sci Total Environ 152034. https://doi.org/10.1016/j.scitotenv.2021.152034 doi: 10.1016/j.scitotenv.2021.152034
![]() |
[27] |
Wu G, Chen S, Meng X, et al. (2021) Development of antifreeze fracturing fluid systems for tight petroleum reservoir stimulations. Energy Fuels 35: 12119-12131. https://doi.org/10.1021/acs.energyfuels.1c01819 doi: 10.1021/acs.energyfuels.1c01819
![]() |
[28] |
Kalghatgi GT (2015) Developments in internal combustion engines and implications for combustion science and future transport fuels. Proc Combust Inst 35: 101-115. https://doi.org/10.1016/j.proci.2014.10.002 doi: 10.1016/j.proci.2014.10.002
![]() |
[29] |
Kanth S, Debbarma S, Das B (2020) Effect of hydrogen enrichment in the intake air of diesel engine fuelled with honge biodiesel blend and diesel. Int J Hydrogen Energy 45: 32521-32533. https://doi.org/10.1016/j.ijhydene.2020.08.152 doi: 10.1016/j.ijhydene.2020.08.152
![]() |
[30] |
Wategave SP, Banapurmath NR, Sawant MS, et al. (2021) Clean combustion and emissions strategy using reactivity controlled compression ignition (RCCI) mode engine powered with CNG-Karanja biodiesel. J Taiwan Inst Chem Eng 124: 116-131. https://doi.org/10.1016/j.jtice.2021.04.055 doi: 10.1016/j.jtice.2021.04.055
![]() |
[31] |
Gavhane RS, Kate AM, Pawar A, et al. (2020) Effect of zinc oxide nano-additives and soybean biodiesel at varying loads and compression ratios on VCR diesel engine characteristics. Symmetry 12: 1042. https://doi.org/10.3390/sym12061042 doi: 10.3390/sym12061042
![]() |
[32] |
Banapurmath N, Chandrashekar T, Soudagar MEM, et al. (2021) Effect of parameters behavior of simarouba methyl ester operated diesel engine. Energies 14: 4973. https://doi.org/10.3390/en14164973 doi: 10.3390/en14164973
![]() |
[33] |
Sateesh K, Yaliwal V, Soudagar MEM, et al. (2021) Utilization of biodiesel/Al2O3 nanoparticles for combustion behavior enhancement of a diesel engine operated on dual fuel mode. J Therm Anal Calorim 1-15. https://doi.org/10.1007/s10973-021-10928-7 doi: 10.1007/s10973-021-10928-7
![]() |
[34] |
Soudagar MEM, Afzal A, Safaei MR, et al. (2020) Investigation on the effect of cottonseed oil blended with different percentages of octanol and suspended MWCNT nanoparticles on diesel engine characteristics. J Therm Anal Calorim 1-18. https://doi.org/10.1007/s10973-020-10293-x doi: 10.1007/s10973-020-10293-x
![]() |
[35] |
Ramachander J, Gugulothu SK, Sastry GR, et al. (2021) Statistical and experimental investigation of the influence of fuel injection strategies on CRDI engine assisted CNG dual fuel diesel engine. Int J Hydrogen Energy 46: 22149-22164. https://doi.org/10.1016/j.ijhydene.2021.04.010 doi: 10.1016/j.ijhydene.2021.04.010
![]() |
[36] | American Elements. Available from: https://www.americanelements.com/graphene-1034343-98-0 |
[37] |
Tayari S, Abedi R (2019) Effect of Chlorella vulgaris methyl ester enriched with hydrogen on performance and emission characteristics of CI engine. Fuel 256: 115906. https://doi.org/10.1016/j.fuel.2019.115906 doi: 10.1016/j.fuel.2019.115906
![]() |
[38] |
Anye Ngang E, Ngayihi Abbe CV (2018) Experimental and numerical analysis of the performance of a diesel engine retrofitted to use LPG as secondary fuel. Appl Therm Eng 136: 462-474. https://doi.org/10.1016/j.applthermaleng.2018.03.022 doi: 10.1016/j.applthermaleng.2018.03.022
![]() |
[39] |
Akcay M, Yilmaz IT, Feyzioglu A (2020) Effect of hydrogen addition on performance and emission characteristics of a common-rail CI engine fueled with diesel/waste cooking oil biodiesel blends. Energy 212: 118538. https://doi.org/10.1016/j.energy.2020.118538 doi: 10.1016/j.energy.2020.118538
![]() |
[40] |
Dharmaraja J, Nguyen DD, Shobana S, et al. (2019) Engine performance, emission and bio characteristics of rice bran oil derived biodiesel blends. Fuel 239: 153-161. https://doi.org/10.1016/j.fuel.2018.10.123 doi: 10.1016/j.fuel.2018.10.123
![]() |
[41] |
Yilmaz İ, Taştan M (2018) Investigation of hydrogen addition to methanol-gasoline blends in an SI engine. Int J Hydrogen Energy 43: 20252-20261. https://doi.org/10.1016/j.ijhydene.2018.07.088 doi: 10.1016/j.ijhydene.2018.07.088
![]() |
[42] |
Su T, Ji C, Wang S, et al. (2017) Improving idle performance of a hydrogen-gasoline rotary engine at stoichiometric condition. Int J Hydrogen Energy 42: 11893-11901. https://doi.org/10.1016/j.ijhydene.2017.01.220 doi: 10.1016/j.ijhydene.2017.01.220
![]() |
[43] |
Gnanamoorthi V, Vimalananth VT (2020) Effect of hydrogen fuel at higher flow rate under dual fuel mode in CRDI diesel engine. Int J Hydrogen Energy 45: 16874-16889. https://doi.org/10.1016/j.ijhydene.2020.04.145 doi: 10.1016/j.ijhydene.2020.04.145
![]() |
[44] |
Tabar AR, Hamidi AA, Ghadamian H (2017) Experimental investigation of CNG and gasoline fuels combination on a 1.7 L bi-fuel turbocharged engine. Int J Energy Environ Eng 8: 37-45. https://doi.org/10.1007/s40095-016-0223-3 doi: 10.1007/s40095-016-0223-3
![]() |
[45] |
Sonachalam M, PaulPandian P, Manieniyan V (2020) Emission reduction in diesel engine with acetylene gas and biodiesel using inlet manifold injection. Clean Technol Environ Policy 22: 2177-2191. https://doi.org/10.1007/s10098-020-01968-y doi: 10.1007/s10098-020-01968-y
![]() |
[46] |
Akansu SO, Tangoz S, Kahraman N, et al. (2017) Experimental study of gasoline-ethanol-hydrogen blends combustion in an SI engine. Int J Hydrogen Energy. https://doi.org/10.1016/j.ijhydene.2017.07.014 doi: 10.1016/j.ijhydene.2017.07.014
![]() |
[47] |
Najafi G, Ghobadian B, Moosavian A, et al. (2016) SVM and ANFIS for prediction of performance and exhaust emissions of a SI engine with gasoline-ethanol blended fuels. Appl Therm Eng 95: 186-203. https://doi.org/10.1016/j.applthermaleng.2015.11.009 doi: 10.1016/j.applthermaleng.2015.11.009
![]() |
[48] |
Nour M, Attia AMA, Nada SA (2019) Improvement of CI engine combustion and performance running on ternary blends of higher alcohol (Pentanol and Octanol)/hydrous ethanol/diesel. Fuel 251: 10-22. https://doi.org/10.1016/j.fuel.2019.04.026 doi: 10.1016/j.fuel.2019.04.026
![]() |
[49] |
Yesilyurt MK, Eryilmaz T, Arslan M (2018) A comparative analysis of the engine performance, exhaust emissions and combustion behaviors of a compression ignition engine fuelled with biodiesel/diesel/1-butanol (C4 alcohol) and biodiesel/diesel/n-pentanol (C5 alcohol) fuel blends. Energy 165: 1332-1351. https://doi.org/10.1016/j.energy.2018.10.100 doi: 10.1016/j.energy.2018.10.100
![]() |
[50] |
Edwin Geo V, Jesu Godwin D, Thiyagarajan S, et al. (2019) Effect of higher and lower order alcohol blending with gasoline on performance, emission and combustion characteristics of SI engine. Fuel 256: 115806. https://doi.org/10.1016/j.fuel.2019.115806 doi: 10.1016/j.fuel.2019.115806
![]() |
[51] |
Phuangwongtrakul S, Wechsatol W, Sethaput T, et al. (2016) Experimental study on sparking ignition engine performance for optimal mixing ratio of ethanol-gasoline blended fuels. Appl Therm Eng 100: 869-879. https://doi.org/10.1016/j.applthermaleng.2016.02.084 doi: 10.1016/j.applthermaleng.2016.02.084
![]() |
[52] |
Yesilyurt MK, Yilbasi Z, Aydin M (2020) The performance, emissions, and combustion characteristics of an unmodified diesel engine running on the ternary blends of pentanol/safflower oil biodiesel/diesel fuel. J Therm Anal Calorim 140: 2903-2942. https://doi.org/10.1007/s10973-020-09376-6 doi: 10.1007/s10973-020-09376-6
![]() |
[53] |
Doğan B, Erol D, Yaman H, et al. (2017) The effect of ethanol-gasoline blends on performance and exhaust emissions of a spark ignition engine through exergy analysis. Appl Therm Eng 120: 433-443. https://doi.org/10.1016/j.applthermaleng.2017.04.012 doi: 10.1016/j.applthermaleng.2017.04.012
![]() |
[54] |
Uslu S, Celik MB (2018) Prediction of engine emissions and performance with artificial neural networks in a single cylinder diesel engine using diethyl ether. Eng Sci Technol, Int J 21: 1194-1201. https://doi.org/10.1016/j.jestch.2018.08.017 doi: 10.1016/j.jestch.2018.08.017
![]() |
[55] |
Chen C, Yao A, Yao C, et al. (2019) Study of the characteristics of PM and the correlation of soot and smoke opacity on the diesel methanol dual fuel engine. Appl Therm Eng 148: 391-403. https://doi.org/10.1016/j.applthermaleng.2018.11.062 doi: 10.1016/j.applthermaleng.2018.11.062
![]() |
[56] |
Pinzi S, Redel-Macías MD, Leiva-Candia DE, et al. (2017) Influence of ethanol/diesel fuel and propanol/diesel fuel blends over exhaust and noise emissions. Energy Procedia 142: 849-854. https://doi.org/10.1016/j.egypro.2017.12.136 doi: 10.1016/j.egypro.2017.12.136
![]() |
[57] |
Amirabedi M, Jafarmadar S, Khalilarya S (2019) Experimental investigation the effect of Mn2O3 nanoparticle on the performance and emission of SI gasoline fueled with mixture of ethanol and gasoline. Appl Therm Eng 149: 512-519. https://doi.org/10.1016/j.applthermaleng.2018.12.058 doi: 10.1016/j.applthermaleng.2018.12.058
![]() |
[58] |
Jayaraman J, Karthik M, Krishna BM, et al. (2020) Impact of titanium oxide nano additives on the performance characteristics of a CI engine fuelled with manilkarazapota methyl ester blends. Mater Today: Proc. https://doi.org/10.1016/j.matpr.2020.09.497 doi: 10.1016/j.matpr.2020.09.497
![]() |
[59] |
Wei J, He C, Fan C, et al. (2021) Comparison in the effects of alumina, ceria and silica nanoparticle additives on the combustion and emission characteristics of a modern methanol-diesel dual-fuel CI engine. Energy Convers Manage 238: 114121. https://doi.org/10.1016/j.enconman.2021.114121 doi: 10.1016/j.enconman.2021.114121
![]() |
[60] |
Örs I, Sarıkoç S, Atabani AE, et al. (2018) The effects on performance, combustion and emission characteristics of DICI engine fuelled with TiO2 nanoparticles addition in diesel/biodiesel/n-butanol blends. Fuel 234: 177-188. https://doi.org/10.1016/j.fuel.2018.07.024 doi: 10.1016/j.fuel.2018.07.024
![]() |
[61] |
Gumus S, Ozcan H, Ozbey M, et al. (2016) Aluminum oxide and copper oxide nanodiesel fuel properties and usage in a compression ignition engine. Fuel 163: 80-87. https://doi.org/10.1016/j.fuel.2015.09.048 doi: 10.1016/j.fuel.2015.09.048
![]() |
[62] |
Wei J, Yin Z, Wang C, et al. (2021) Impact of aluminium oxide nanoparticles as an additive in diesel-methanol blends on a modern DI diesel engine. Appl Therm Eng 185: 116372. https://doi.org/10.1016/j.applthermaleng.2020.116372 doi: 10.1016/j.applthermaleng.2020.116372
![]() |
[63] |
Heydari-Maleney K, Taghizadeh-Alisaraei A, Ghobadian B, et al. (2017) Analyzing and evaluation of carbon nanotubes additives to diesohol-B2 fuels on performance and emission of diesel engines. Fuel 196: 110-123. https://doi.org/10.1016/j.fuel.2017.01.091 doi: 10.1016/j.fuel.2017.01.091
![]() |
[64] |
Hosseini SH, Taghizadeh-Alisaraei A, Ghobadian B, et al. (2017) Performance and emission characteristics of a CI engine fuelled with carbon nanotubes and diesel-biodiesel blends. Renewable Energy 111: 201-213. https://doi.org/10.1016/j.renene.2017.04.013 doi: 10.1016/j.renene.2017.04.013
![]() |
[65] |
Gad MS, Kamel BM, Anjum Badruddin I (2021) Improving the diesel engine performance, emissions and combustion characteristics using biodiesel with carbon nanomaterials. Fuel 288: 119665. https://doi.org/10.1016/j.fuel.2020.119665 doi: 10.1016/j.fuel.2020.119665
![]() |
[66] |
Razzaq L, Mujtaba MA, Soudagar MEM, et al. (2021) Engine performance and emission characteristics of palm biodiesel blends with graphene oxide nanoplatelets and dimethyl carbonate additives. J Environ Manage 282: 111917. https://doi.org/10.1016/j.jenvman.2020.111917 doi: 10.1016/j.jenvman.2020.111917
![]() |
[67] |
Tomar M, Kumar N (2020) Effect of multi-walled carbon nanotubes and alumina nano-additives in a light duty diesel engine fuelled with schleichera oleosa biodiesel blends. Sustainable Energy Technol Assess 42: 100833. https://doi.org/10.1016/j.seta.2020.100833 doi: 10.1016/j.seta.2020.100833
![]() |
[68] |
Mei D, Zuo L, Adu-Mensah D, et al. (2019) Combustion characteristics and emissions of a common rail diesel engine using nanoparticle-diesel blends with carbon nanotube and molybdenum trioxide. Appl Therm Eng 162: 114238. https://doi.org/10.1016/j.applthermaleng.2019.114238 doi: 10.1016/j.applthermaleng.2019.114238
![]() |
[69] |
Chacko N, Jeyaseelan T (2020) Comparative evaluation of graphene oxide and graphene nanoplatelets as fuel additives on the combustion and emission characteristics of a diesel engine fuelled with diesel and biodiesel blend. Fuel Process Technol 204: 106406. https://doi.org/10.1016/j.fuproc.2020.106406 doi: 10.1016/j.fuproc.2020.106406
![]() |
[70] |
El-Seesy AI, Hassan H (2019) Investigation of the effect of adding graphene oxide, graphene nanoplatelet, and multiwalled carbon nanotube additives with n-butanol-Jatropha methyl ester on a diesel engine performance. Renewable Energy 132: 558-574. https://doi.org/10.1016/j.renene.2018.08.026 doi: 10.1016/j.renene.2018.08.026
![]() |
[71] |
Debbarma S, Misra RD, Das B (2020) Performance of graphene-added palm biodiesel in a diesel engine. Clean Technol Environ Policy 22: 523-534. https://doi.org/10.1007/s10098-019-01800-2 doi: 10.1007/s10098-019-01800-2
![]() |
[72] |
Ning L, Duan Q, Chen Z, et al. (2020) A comparative study on the combustion and emissions of a non-road common rail diesel engine fueled with primary alcohol fuels (methanol, ethanol, and n-butanol)/diesel dual fuel. Fuel 266: 117034. https://doi.org/10.1016/j.fuel.2020.117034 doi: 10.1016/j.fuel.2020.117034
![]() |
[73] |
Rastogi PM, Sharma A, Kumar N (2021) Effect of CuO nanoparticles concentration on the performance and emission characteristics of the diesel engine running on jojoba (Simmondsia Chinensis) biodiesel. Fuel 286: 119358. https://doi.org/10.1016/j.fuel.2020.119358 doi: 10.1016/j.fuel.2020.119358
![]() |
[74] |
El-Seesy AI, Hassan H, Ookawara S (2018) Effects of graphene nanoplatelet addition to jatropha Biodiesel-Diesel mixture on the performance and emission characteristics of a diesel engine. Energy 147: 1129-1152. https://doi.org/10.1016/j.energy.2018.01.108 doi: 10.1016/j.energy.2018.01.108
![]() |
[75] |
Bhargavi M, Vinod Kumar T, Ali Azmath Shaik R, et al. (2021) Effective utilization and optimization of waste plastic oil with ethanol additive in diesel engine using full factorial design. Mater Today: Proc. https://doi.org/10.1016/j.matpr.2021.10.310 doi: 10.1016/j.matpr.2021.10.310
![]() |
[76] |
Loganathan S, Leenus Jesu Martin M, Nagalingam B, et al. (2018) Heat release rate and performance simulation of DME fuelled diesel engine using oxygenate correction factor and load correction factor in double Wiebe function. Energy 150: 77-91. https://doi.org/10.1016/j.energy.2018.02.112 doi: 10.1016/j.energy.2018.02.112
![]() |
[77] |
Subramani S, Govindasamy R, Rao GLN (2020) Predictive correlations for NOx and smoke emission of DI CI engine fuelled with diesel-biodiesel-higher alcohol blends-response surface methodology approach. Fuel 269: 117304. https://doi.org/10.1016/j.fuel.2020.117304 doi: 10.1016/j.fuel.2020.117304
![]() |
[78] |
Singh Y, Sharma A, Kumar Singh G, et al. (2018) Optimization of performance and emission parameters of direct injection diesel engine fuelled with pongamia methyl esters-response surface methodology approach. Ind Crops Prod 126: 218-226. https://doi.org/10.1016/j.indcrop.2018.10.035 doi: 10.1016/j.indcrop.2018.10.035
![]() |
[79] |
Rai A, Kumar NS, Srinivasa Pai P, et al. (2012) Fuzzy Logic Based Prediction of Performance and Emission Parameters of a LPG-Diesel Dual Fuel Engine. Procedia Eng 38: 280-292. https://doi.org/10.1016/j.proeng.2012.06.036 doi: 10.1016/j.proeng.2012.06.036
![]() |
[80] |
Manigandan S, Atabani AE, Ponnusamy VK, et al. (2020) Effect of hydrogen and multiwall carbon nanotubes blends on combustion performance and emission of diesel engine using Taguchi approach. Fuel 276: 118120. https://doi.org/10.1016/j.fuel.2020.118120 doi: 10.1016/j.fuel.2020.118120
![]() |
[81] |
Srinidhi C, Madhusudhan A, Channapattana SV, et al. (2021) RSM based parameter optimization of CI engine fuelled with nickel oxide dosed Azadirachta indica methyl ester. Energy 234: 121282. https://doi.org/10.1016/j.energy.2021.121282 doi: 10.1016/j.energy.2021.121282
![]() |
[82] |
Ghanbari M, Mozafari-Vanani L, Dehghani-Soufi M, et al. (2021) Effect of alumina nanoparticles as additive with diesel-biodiesel blends on performance and emission characteristic of a six-cylinder diesel engine using response surface methodology (RSM). Energy Convers Manage: X 11: 100091. https://doi.org/10.1016/j.ecmx.2021.100091 doi: 10.1016/j.ecmx.2021.100091
![]() |
[83] |
Fayaz H, Mujtaba MA, Soudagar MEM, et al. (2021) Collective effect of ternary nano fuel blends on the diesel engine performance and emissions characteristics. Fuel 293: 120420. https://doi.org/10.1016/j.fuel.2021.120420 doi: 10.1016/j.fuel.2021.120420
![]() |
[84] |
Solmaz H, Ardebili SMS, Calam A, et al. (2021) Prediction of performance and exhaust emissions of a CI engine fueled with multi-wall carbon nanotube doped biodiesel-diesel blends using response surface method. Energy 227: 120518. https://doi.org/10.1016/j.energy.2021.120518 doi: 10.1016/j.energy.2021.120518
![]() |
[85] |
Safieddin Ardebili SM, Taghipoor A, Solmaz H, et al. (2020) The effect of nano-biochar on the performance and emissions of a diesel engine fueled with fusel oil-diesel fuel. Fuel 268: 117356. https://doi.org/10.1016/j.fuel.2020.117356 doi: 10.1016/j.fuel.2020.117356
![]() |
1. | Gude Naga Venkata Siddartha, Ch. Siva Ramakrishna, Praveen Kumar Kujur, Y. Anupam Rao, Nishit Dalela, Anil Singh Yadav, Abhishek Sharma, Effect of fuel additives on internal combustion engine performance and emissions, 2022, 63, 22147853, A9, 10.1016/j.matpr.2022.06.307 | |
2. | S. Vignesh, R. Rathish, S. Johnpriyan, K. Nandha kumar, I. Sujin, Study on emission reduction techniques by in-cylinder reduction method for compression ignition engines, 2022, 66, 22147853, 1267, 10.1016/j.matpr.2022.05.125 | |
3. | A. Devaraj, M. Nagappan, D. Yogaraj, Om Prakash, Y Anupam Rao, Abhishek Sharma, Influence of nano-additives on engine behaviour using diesel-biodiesel blend, 2022, 62, 22147853, 2266, 10.1016/j.matpr.2022.03.598 | |
4. | Sanjesh Kumar, Geetesh Goga, Review analysis on the performance & emission characteristics of a diesel engine fuelled with various gaseous & bio fuels, 2023, 22147853, 10.1016/j.matpr.2023.01.189 | |
5. | Adem Siraj Mohammed, Samson Mekbib Atnaw, Ancha Venkata Ramaya, Getachew Alemayehu, A comprehensive review on the effect of ethers, antioxidants, and cetane improver additives on biodiesel-diesel blend in CI engine performance and emission characteristics, 2023, 108, 17439671, 101227, 10.1016/j.joei.2023.101227 | |
6. | Ameer Hasan Hamzah, Abdulrazzak Akroot, Hasanain A. Abdul Wahhab, Rabeea M. Ghazal, Abdulrahman E.J. Alhamd, Mothana Bdaiwi, Effects of nano-additives in developing alternative fuel strategy for CI engines: A critical review with a focus on the performance and emission characteristics, 2024, 22, 25901230, 102248, 10.1016/j.rineng.2024.102248 | |
7. | Shresht Kohli, B. Pavani Srikavya, Nishikant Kishor Dhapekar, Rajesh Tiwari, Rahul Kumar, Anil Singh Yadav, Neeraj Sharma, Abhishek Sharma, Impact of nano materials on engine performance run on biofuels, 2023, 22147853, 10.1016/j.matpr.2023.07.133 | |
8. | J. Senthilkumar, K. Dharun, T. Sanchit Kumar, R. Suresh Kumar, N. Jayanthi, S. Venkatesh, Impact of nano catalyst in the biodiesel production for direct injection diesel engine: A review, 2024, 33, 1088-1913, 257, 10.1002/tqem.22057 | |
9. | E. E. Onojowho, A. A. Asere, Effects of the fuel blend flow rate on engine combustion performance, 2024, 12, 2296-598X, 10.3389/fenrg.2024.1335507 | |
10. | Nur Ayshah Rosli, Teh Ubaidah Noh, 2024, 9780124095472, 10.1016/B978-0-443-15740-0.00079-3 | |
11. | A S M Sazzad Parveg, Albert Ratner, A comprehensive review of liquid fuel droplet evaporation and combustion behavior with carbon-based nanoparticles, 2025, 106, 03601285, 101198, 10.1016/j.pecs.2024.101198 | |
12. | Soroush Gholami Ghanati, Battal Doğan, Murat Kadir Yeşilyurt, The effects of the usage of silicon dioxide (SiO 2 ) and titanium dioxide (TiO 2 ) as nano-sized fuel additives on the engine characteristics in diesel engines: a review , 2024, 15, 1759-7269, 229, 10.1080/17597269.2023.2221882 | |
13. | Rongshuo Zhang, Rencheng Zhu, Ming Jia, Yujie Pang, Bowen Zhang, Xiaofeng Bao, Yunjing Wang, Improvement of a Rapid Method of Detecting Gasoline Detergency Based on the Image Recognition, 2023, 8, 2470-1343, 34134, 10.1021/acsomega.3c05350 | |
14. | Sertaç COŞMAN, Experimental Research of the Effects of Benzoylthiourea Derivative Fuel and Gasoline Mixtures on Engine Performance and Emissions, 2023, 7, 2587-0963, 403, 10.30939/ijastech..1397506 | |
15. | Treza Wambui, Meshack Hawi, Francis Njoka, Joseph Kamau, Performance enhancement and emissions reduction in a diesel engine using oleander and croton biodiesel doped with graphene nanoparticles, 2023, 12, 2252-4940, 635, 10.14710/ijred.2023.51785 | |
16. | Sanjesh Kumar, Geetesh Goga, 2024, Chapter 10, 978-981-97-6351-1, 149, 10.1007/978-981-97-6352-8_10 | |
17. | Khwaja Alamgir Ahmad, Shariq Farhan Elahi, Hussein Znad, Ejaz Ahmad, Kinetic and mechanistic investigation of Butyl Levulinate synthesis on ZSM-5 supported phosphomolybdic acid, 2025, 15, 2045-2322, 10.1038/s41598-025-89953-7 | |
18. | Arundhathi Racha, Shivanand Pai, Chanchal Samanta, Bharat L. Newalkar, Sustainable Fuel Additives Derived from Renewable Resources: Promising Strategies for a Greener Future, 2025, 2470-1343, 10.1021/acsomega.4c11343 |
![]() |
CV [MJ/kg] | Density [kg/m3] | Kinematic Viscosity [mm2/s] | Flash point [℃] |
pure diesel | 44 | 825.96 | 3.96 | 72 |
Biodiesel Chlorella Vulgaris | 38.7 | 860 | 3.7 | 124 |
honge biodiesel HB20 | 42.123 | 835 | 4.12 | 104 |
![]() |
CV [MJ/kg] | Density [kg/m3] | Kinematic Viscosity [mm2/s] |
Flash point [℃] |
hydrogen | 119.93 | 0.0838 | 110 | - |
methanol | 20.30 | 790 | 0.59 | 12 |
ethanol | 27 | 785 | 1.1 | 14 |
CNG | 45.76 | 720 | - | 81.6 |
![]() |
CV [MJ/kg] | Density [g/cm3] | Specific Surface Area (m2g-1) | Thermal conductivity (kW/mK) |
SWCNT | 1.3–1.4 | 1315 | 1750–3800 | |
Graphene | 31.82 | 2.267 | 1598 | 5300 |
Gaseous Additives | ||||||
Ref. | Engine | Fuel | Additive | Method | Performance | Emissions |
[29] | 4-cylinder Diesel | B20 | Al2O3 + H2 | Full load between 1200–2800 rpm | Power ↑ BTE ↑ BSFC ↓ |
CO ↓ CO2 ↑ NOx ↑ |
[37] | 1-cylinder Diesel | B20 |
H2 | Full load. Various speed |
Torque ↑ Power ↑ BTE ↑ |
CO ↓ CO2 ↓ HC ↓ NOx ↑ |
[43] | 1-cylinder Diesel | Diesel | H2 | Constant speed 1500 rpm | BTE ↑ |
CO ↓ CO2 ↓ HC ↓ NOx ↑ |
[45] | 1-cylinder Diesel | Biodiesel | Acetylene | 1500 rpm Different loads |
BTE ↑ |
CO ↓ HC ↓ NOx ↓ |
[38] | 4-cylinder diesel | diesel | LPG | different Speeds and loads | Torque ↑ Power ↑ |
HC ↓ NOx ↓ |
Liquid Additives | ||||||
Ref. | Engine | Fuel | Additive | Method | Performance | Emissions |
[48] | 1-cylinder Diesel | Hydrous ethanol-Diesel | Pentanol and Octanol | Different blends at different loads | Power ↓ BTE ↑ BSFC ↑ |
CO ↓ CO2 ↓ NOx ↓ |
[72] | 1-cylinder Diesel | Diesel | Alcohol | Constant speed 2500 rpm. Different alcohol percentage. | BTE ↓ |
CO ↓ HC ↑ NOx ↑ |
[52] | 1-cylinder Diesel | Biodiesel | Pentanol | 3000 rpm Different engine loads |
BSFC ↑ BTE ↓ |
CO ↓ CO2 ↑ NOx ↓ |
Solid Additives (Metal-based) | ||||||
Ref. | Engine | Fuel | Additive | Method | Performance | Emissions |
[73] | 1-cylinder Diesel |
biodiesel | CuO | Various loads | BTE ↑ BSFC ↓ |
CO ↓ HC ↓ NOx ↑ |
[62] | 1-cylinder Diesel |
Diesel-methanol | Al2O3 | Constant speed 1400 rpm. Different loads | BTE ↑ BSFC ↓ |
CO ↓ HC ↓ NOx ↑ |
[60] | 1-cylinder Diesel |
Waste Cooking Biodiesel | TiO2 | Various engine speeds. Full load | Torque ↑ Power ↑ BSFC ↓ |
CO ↓ CO2 ↑ HC ↓ NO ↑ |
Solid Additives (Carbon-based) | ||||||
Ref. | Engine | Fuel | Additive | Method | Performance | Emissions |
[63] | 1-cylinder Diesel | Diesohol (diesel + alcohol) | CNT | Full load at different rpm | Torque ↑ Power ↑ BTE ↑ BSFC ↓ |
CO ↓ CO2 ↓ HC ↓ NOx ↑ |
[67] | 1-cylinder Diesel | Diesel & Diesel-biodiesel blend | MWCNT | Constant speed 1500 rpm. Different loads | BTE ↑ BSFC ↓ |
CO ↓ NOX↓ |
[64] | 1-cylinder Diesel | Diesel-biodiesel blend | CNT | Full load. Different blends and speed | Power ↑ Torque ↑ BTE ↓ BSFC ↓ |
CO ↓ CO2 ↑ HC ↓ NOX ↑ |
[66] | 1-cylinder Diesel | Palm oil biodiesel | Graphene Oxide Nanoplatelets (GNP) | Varying engine speed. Different blends | BTE ↑ BSFC ↑ |
HC ↓ CO ↑ NOX↓ |
[74] | 1-cylinder Diesel | Jatropha biodiesel | Graphene Nanoplatelets (GNP) | Different Speeds, different loads, different fuel blends | BSFC ↓ | CO ↓ HC ↓ NOX ↓ |
No | Base fuel | Additives/blends | Parameters | Prediction Method | Software/Tool | Reference |
Liquid Additives | ||||||
1 | Waste plastic oil | ethanol | HC, CO, SFC | DOE with Full factorial design | Minitab | [75] |
2 | diesel | DME | BTE, BSEC | Double Weibe | - | [76] |
3 | diesel | higher alcohol additive (Decanol) | BSFC, BTE, CO, CO2, HC, NOx | ANN and RSM | Design Expert | [77] |
4 | diesel | pongamia methyl esters | BTE, UHC, NOx | RSM | Minitab | [78] |
Gaseous Additives | ||||||
5 | diesel | CNG | BSFC, BTE, CO, CO2, HC, NOx | RSM | Minitab and Design Expert | [35] |
6 | diesel | LPG | BSEC, BTE | Fuzzy Logic | ANFIS | [79] |
7 | diesel | Hydrogen |
CO, CO2, HC, NOx | DOE with Taguchi | Minitab | [80] |
Solid Additives (Metal) | ||||||
8 | biodiesel | NiO | BSFC NOx, HC, CO |
RSM | Design Expert | [81] |
9 | biodiesel | Alumina nanoparticles | BSFC, BTE, CO, NOx | RSM | Design Expert | [82] |
10 | biodiesel | TiO2, and Al2O3 nanoparticles | BSFC, BTE, CO, NOx | RSM | Design Expert | [83] |
Solid Additives (Carbon based) | ||||||
11 | diesel and biodiesel | MWCNT | BSFC, CO, NOx | RSM | Design Expert | [83] |
12 | diesel and biodiesel | MWCNT | BTE, BSFC, UHC, CO, NOx | RSM | Design Expert | [84] |
13 | fusel oil | nano- biochar | Torque, Power, BSFC, BTE, CO, NOx | RSM | Design Expert | [85] |
![]() |
CV [MJ/kg] | Density [kg/m3] | Kinematic Viscosity [mm2/s] | Flash point [℃] |
pure diesel | 44 | 825.96 | 3.96 | 72 |
Biodiesel Chlorella Vulgaris | 38.7 | 860 | 3.7 | 124 |
honge biodiesel HB20 | 42.123 | 835 | 4.12 | 104 |
![]() |
CV [MJ/kg] | Density [kg/m3] | Kinematic Viscosity [mm2/s] |
Flash point [℃] |
hydrogen | 119.93 | 0.0838 | 110 | - |
methanol | 20.30 | 790 | 0.59 | 12 |
ethanol | 27 | 785 | 1.1 | 14 |
CNG | 45.76 | 720 | - | 81.6 |
![]() |
CV [MJ/kg] | Density [g/cm3] | Specific Surface Area (m2g-1) | Thermal conductivity (kW/mK) |
SWCNT | 1.3–1.4 | 1315 | 1750–3800 | |
Graphene | 31.82 | 2.267 | 1598 | 5300 |
Gaseous Additives | ||||||
Ref. | Engine | Fuel | Additive | Method | Performance | Emissions |
[29] | 4-cylinder Diesel | B20 | Al2O3 + H2 | Full load between 1200–2800 rpm | Power ↑ BTE ↑ BSFC ↓ |
CO ↓ CO2 ↑ NOx ↑ |
[37] | 1-cylinder Diesel | B20 |
H2 | Full load. Various speed |
Torque ↑ Power ↑ BTE ↑ |
CO ↓ CO2 ↓ HC ↓ NOx ↑ |
[43] | 1-cylinder Diesel | Diesel | H2 | Constant speed 1500 rpm | BTE ↑ |
CO ↓ CO2 ↓ HC ↓ NOx ↑ |
[45] | 1-cylinder Diesel | Biodiesel | Acetylene | 1500 rpm Different loads |
BTE ↑ |
CO ↓ HC ↓ NOx ↓ |
[38] | 4-cylinder diesel | diesel | LPG | different Speeds and loads | Torque ↑ Power ↑ |
HC ↓ NOx ↓ |
Liquid Additives | ||||||
Ref. | Engine | Fuel | Additive | Method | Performance | Emissions |
[48] | 1-cylinder Diesel | Hydrous ethanol-Diesel | Pentanol and Octanol | Different blends at different loads | Power ↓ BTE ↑ BSFC ↑ |
CO ↓ CO2 ↓ NOx ↓ |
[72] | 1-cylinder Diesel | Diesel | Alcohol | Constant speed 2500 rpm. Different alcohol percentage. | BTE ↓ |
CO ↓ HC ↑ NOx ↑ |
[52] | 1-cylinder Diesel | Biodiesel | Pentanol | 3000 rpm Different engine loads |
BSFC ↑ BTE ↓ |
CO ↓ CO2 ↑ NOx ↓ |
Solid Additives (Metal-based) | ||||||
Ref. | Engine | Fuel | Additive | Method | Performance | Emissions |
[73] | 1-cylinder Diesel |
biodiesel | CuO | Various loads | BTE ↑ BSFC ↓ |
CO ↓ HC ↓ NOx ↑ |
[62] | 1-cylinder Diesel |
Diesel-methanol | Al2O3 | Constant speed 1400 rpm. Different loads | BTE ↑ BSFC ↓ |
CO ↓ HC ↓ NOx ↑ |
[60] | 1-cylinder Diesel |
Waste Cooking Biodiesel | TiO2 | Various engine speeds. Full load | Torque ↑ Power ↑ BSFC ↓ |
CO ↓ CO2 ↑ HC ↓ NO ↑ |
Solid Additives (Carbon-based) | ||||||
Ref. | Engine | Fuel | Additive | Method | Performance | Emissions |
[63] | 1-cylinder Diesel | Diesohol (diesel + alcohol) | CNT | Full load at different rpm | Torque ↑ Power ↑ BTE ↑ BSFC ↓ |
CO ↓ CO2 ↓ HC ↓ NOx ↑ |
[67] | 1-cylinder Diesel | Diesel & Diesel-biodiesel blend | MWCNT | Constant speed 1500 rpm. Different loads | BTE ↑ BSFC ↓ |
CO ↓ NOX↓ |
[64] | 1-cylinder Diesel | Diesel-biodiesel blend | CNT | Full load. Different blends and speed | Power ↑ Torque ↑ BTE ↓ BSFC ↓ |
CO ↓ CO2 ↑ HC ↓ NOX ↑ |
[66] | 1-cylinder Diesel | Palm oil biodiesel | Graphene Oxide Nanoplatelets (GNP) | Varying engine speed. Different blends | BTE ↑ BSFC ↑ |
HC ↓ CO ↑ NOX↓ |
[74] | 1-cylinder Diesel | Jatropha biodiesel | Graphene Nanoplatelets (GNP) | Different Speeds, different loads, different fuel blends | BSFC ↓ | CO ↓ HC ↓ NOX ↓ |
No | Base fuel | Additives/blends | Parameters | Prediction Method | Software/Tool | Reference |
Liquid Additives | ||||||
1 | Waste plastic oil | ethanol | HC, CO, SFC | DOE with Full factorial design | Minitab | [75] |
2 | diesel | DME | BTE, BSEC | Double Weibe | - | [76] |
3 | diesel | higher alcohol additive (Decanol) | BSFC, BTE, CO, CO2, HC, NOx | ANN and RSM | Design Expert | [77] |
4 | diesel | pongamia methyl esters | BTE, UHC, NOx | RSM | Minitab | [78] |
Gaseous Additives | ||||||
5 | diesel | CNG | BSFC, BTE, CO, CO2, HC, NOx | RSM | Minitab and Design Expert | [35] |
6 | diesel | LPG | BSEC, BTE | Fuzzy Logic | ANFIS | [79] |
7 | diesel | Hydrogen |
CO, CO2, HC, NOx | DOE with Taguchi | Minitab | [80] |
Solid Additives (Metal) | ||||||
8 | biodiesel | NiO | BSFC NOx, HC, CO |
RSM | Design Expert | [81] |
9 | biodiesel | Alumina nanoparticles | BSFC, BTE, CO, NOx | RSM | Design Expert | [82] |
10 | biodiesel | TiO2, and Al2O3 nanoparticles | BSFC, BTE, CO, NOx | RSM | Design Expert | [83] |
Solid Additives (Carbon based) | ||||||
11 | diesel and biodiesel | MWCNT | BSFC, CO, NOx | RSM | Design Expert | [83] |
12 | diesel and biodiesel | MWCNT | BTE, BSFC, UHC, CO, NOx | RSM | Design Expert | [84] |
13 | fusel oil | nano- biochar | Torque, Power, BSFC, BTE, CO, NOx | RSM | Design Expert | [85] |