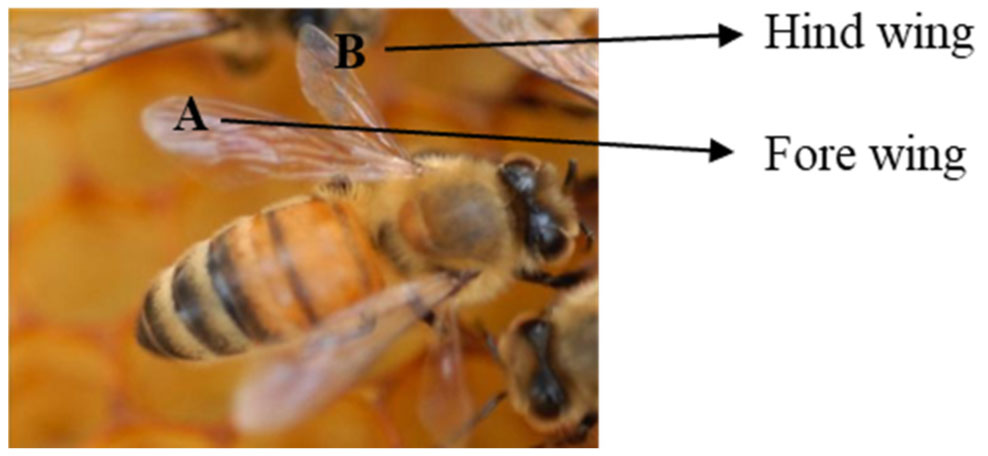
The next transformation in human civilization is occurring in the energy sector. The transition is supported mostly by concern for environmental safety (climate change). Other factors such as energy security and economics also dictate the shift. The transition will be more disruptive to oil-exporting economies such as Nigeria, which depend heavily on it for economic growth. Historical inferences from past energy transitions reveals that discovery and massive acceleration of new energy resources alters mode of production, energy consumption, economic innovation and unlocks multiplier effects on the society. Technology and availability of energy resources is the critical enabler of past transitions. Renewable energy technologies are becoming more cost-competitive relative to fossil fuels. The increasing transition will have serious socioeconomic and political implications, if the country does not diversify its economy. For example, contraction in oil export because of global transition to renewable energy will reduce Nigeria government's revenue, increase unemployment, decrease capacity to finance infrastructure and development projects, and increase poverty rate. Nigeria has enormous renewable energy potential—solar, wind, biomass, etc. The use of renewables will improve environmental quality. For example, increased use of biomass (organic wastes) for energy production will advance Nigeria solid waste management system and vice versa. All these are associated with sustainable resource management.
Citation: Chukwuebuka Okafor, Christian Madu, Charles Ajaero, Juliet Ibekwe, Happy Bebenimibo, Chinelo Nzekwe. Moving beyond fossil fuel in an oil-exporting and emerging economy: Paradigm shift[J]. AIMS Energy, 2021, 9(2): 379-413. doi: 10.3934/energy.2021020
[1] | Sunarno Sunarno, Nelly Puspandari, Fitriana Fitriana, Uly Alfi Nikmah, Hasta Handayani Idrus, Novaria Sari Dewi Panjaitan . Extended spectrum beta lactamase (ESBL)-producing Escherichia coli and Klebsiella pneumoniae in Indonesia and South East Asian countries: GLASS Data 2018. AIMS Microbiology, 2023, 9(2): 218-227. doi: 10.3934/microbiol.2023013 |
[2] | Tru Tran, Stephanie N. Dawrs, Grant J. Norton, Ravleen Virdi, Jennifer R. Honda . Brought to you courtesy of the red, white, and blue–pigments of nontuberculous mycobacteria. AIMS Microbiology, 2020, 6(4): 434-450. doi: 10.3934/microbiol.2020026 |
[3] | Noah T Thompson, David A Kitzenberg, Daniel J Kao . Persister-mediated emergence of antimicrobial resistance in agriculture due to antibiotic growth promoters. AIMS Microbiology, 2023, 9(4): 738-756. doi: 10.3934/microbiol.2023038 |
[4] | Manjusha Lekshmi, Parvathi Ammini, Jones Adjei, Leslie M. Sanford, Ugina Shrestha, Sanath Kumar, Manuel F. Varela . Modulation of antimicrobial efflux pumps of the major facilitator superfamily in Staphylococcus aureus. AIMS Microbiology, 2018, 4(1): 1-18. doi: 10.3934/microbiol.2018.1.1 |
[5] | Wanda C Reygaert . An overview of the antimicrobial resistance mechanisms of bacteria. AIMS Microbiology, 2018, 4(3): 482-501. doi: 10.3934/microbiol.2018.3.482 |
[6] | Matthew Johnston, Michael McBride, Divakar Dahiya, Richard Owusu-Apenten, Poonam Singh Nigam . Antibacterial activity of Manuka honey and its components: An overview. AIMS Microbiology, 2018, 4(4): 655-664. doi: 10.3934/microbiol.2018.4.655 |
[7] | Ana M. Castañeda-Meléndrez, José A. Magaña-Lizárraga, Marcela Martínez-Valenzuela, Aldo F. Clemente-Soto, Patricia C. García-Cervantes, Francisco Delgado-Vargas, Rodolfo Bernal-Reynaga . Genomic characterization of a multidrug-resistant uropathogenic Escherichia coli and evaluation of Echeveria plant extracts as antibacterials. AIMS Microbiology, 2024, 10(1): 41-61. doi: 10.3934/microbiol.2024003 |
[8] | Manuela Oliveira, Eva Cunha, Luís Tavares, Isa Serrano . P. aeruginosa interactions with other microbes in biofilms during co-infection. AIMS Microbiology, 2023, 9(4): 612-646. doi: 10.3934/microbiol.2023032 |
[9] | Thomas Bintsis . Lactic acid bacteria as starter cultures: An update in their metabolism and genetics. AIMS Microbiology, 2018, 4(4): 665-684. doi: 10.3934/microbiol.2018.4.665 |
[10] | Divakar Dahiya, Caoimhin Mackin, Poonam Singh Nigam . Studies on bioactivities of Manuka and regional varieties of honey for their potential use as natural antibiotic agents for infection control related to wound healing and in pharmaceutical formulations. AIMS Microbiology, 2024, 10(2): 288-310. doi: 10.3934/microbiol.2024015 |
The next transformation in human civilization is occurring in the energy sector. The transition is supported mostly by concern for environmental safety (climate change). Other factors such as energy security and economics also dictate the shift. The transition will be more disruptive to oil-exporting economies such as Nigeria, which depend heavily on it for economic growth. Historical inferences from past energy transitions reveals that discovery and massive acceleration of new energy resources alters mode of production, energy consumption, economic innovation and unlocks multiplier effects on the society. Technology and availability of energy resources is the critical enabler of past transitions. Renewable energy technologies are becoming more cost-competitive relative to fossil fuels. The increasing transition will have serious socioeconomic and political implications, if the country does not diversify its economy. For example, contraction in oil export because of global transition to renewable energy will reduce Nigeria government's revenue, increase unemployment, decrease capacity to finance infrastructure and development projects, and increase poverty rate. Nigeria has enormous renewable energy potential—solar, wind, biomass, etc. The use of renewables will improve environmental quality. For example, increased use of biomass (organic wastes) for energy production will advance Nigeria solid waste management system and vice versa. All these are associated with sustainable resource management.
In recent years the number of infections associated with antimicrobial resistance has increased and is emerging as one of the leading public health threats of the 21st century [1],[2]. The accelerated spread of antibiotic resistance is due to the inappropriate and excessive use of antibiotics in the past few decades [3]. Nanomaterials are a promising means in curbing the use of antibiotics due to their mechanism of preventing bacterial attachment to kill bacteria [4]. The bactericidal mechanisms associated with nanomaterials are generally divided into two major categories. Nanomaterials may act as carriers, delivering antibiotics to bacteria increasing drug potency and minimizing overall drug exposure. Also, nanomaterials can generate lethal damage to microbes through a physical process that destroys extracellular polymeric substances (EPS) using either enzymes or mechanical forces [4]. Insect wings have nanometer-sized structures with the potential to break EPS [5].
Insect wings are membranous, parchment-like, heavily sclerotized, and can be fringed with long hairs or covered with scales [6]. The wings enable a myriad of ecologically important behaviors including flight, thermal collection, gyroscopic stabilization, sound production, fellow species recognition, sexual overtures and contact, and protective cover [7]–[9]. Various insect wings such as cicada (e.g., Psaltoda claripennis) (Ashton, 1921), damselfly fly (e.g., Calopteryx haemorrhoidalis (Vander Linden, 1825) and dragonfly (e.g., Pantala flavescens (Fabricius, 1798)) maintain contaminant-free status and possess antimicrobial properties [10]–[12]. It has been established that the antimicrobial properties of the wings of cicada, damselfly fly, and dragon flies are mediated by the physical nanoprotrusions or nanopillars found on the wing surface [13],[14]. The nanopillars are known to damage bacteria by physically puncturing the bacterial envelope, inducing deformation [15]–[18]. These nanoarchitectures are effective against a wide range of bacteria including Pseudomonas aeruginosa, Escherichia coli, Branhamella catarrhalis, and Pseudomonas fluorescens, Bacillus subtilis, Pseudococcus maritimus, and Staphylococcus aureus [13],[15],[19],[20]. Although the antibiofouling (self-cleaning ability) and antimicrobial efficiency of cicada, damselfly fly and dragonfly has been widely reported, the effectiveness of the wings of honeybees against bacteria, however, is yet to be established.
Honeybees Apis mellifera (Linnaeus, 1758) are considered as super-generalist pollinators in agricultural systems [21],[22]. These social and hardworking insects can produce royal jelly, beeswax, propolis, venom and honey for various nutritional and medicinal purposes [23],[24]. To date, few studies have concentrated on the antimicrobial activity of honeybee products, which have been used extensively since ancient times, as documented in Egyptian, Roman, Chinese and Persian literatures [25]. The antimicrobial activity of honey is mediated by the activation of the enzyme glucose oxidase which oxidizes glucose to gluconic acid and hydrogen peroxide (H2O2), a potent antimicrobial agent [26]–[28].
In contrast, the topography of honeybee wings has received less attention. The role topography plays in antibiofouling, and antimicrobial activity of honeybee wings has never been investigated. In this study we investigated the antibiofouling and antimicrobial activities of the wings of non-reproductive female honeybees, also called “workers.” The workers were selected for this study because they are by far the most numerous castes in the hives and perform all the work needed to keep the colony fed and healthy. As the workers carry out these tasks inside and outside the hive, they could theoretically be exposed to a wide variety of pathogens providing an opportunity for pathogens to spread from the workers to the other castes, e.g., drones and queens [29]. The results of this study will help to explain why honeybee workers are such successful foragers. Also, these findings could lead to the development of self-cleaning and antimicrobial surfaces with myriad applications including hospital surfaces, filters for high-risk indwelling devices (e.g., foley catheters), agricultural and food service applications etc.
Thirty mature honeybee (Apis mellifera) workers were collected from Dr. Jeffery Andrew Meixner's beehive, in Winston Salem, North Carolina USA. The wings of honeybee are arranged in two pairs, connected by a row of hooks on the back wing [30] (Figure 1). The fore wings (~0.8 cm) are much larger than the hind wings (~0.4 cm). Both fore and hind wings of each specimen were incised by a scalpel from the body of the insect meticulously (avoiding damage to their surfaces) and stored at room temperature in sterile polystyrene Petri dishes (Fisher Scientific) until required.
Scanning electron microscopy (SEM) was used to observe the dorsal and ventral surfaces of pristine honeybee wings for the presence of microbes. SEM has been used intensively to revealed micro- and nano scales on wing surfaces [13]–[15],[30]. The wings were glued onto copper pin mounts with a double-sided carbon adhesive tape, and SEM imaging was done using a scanning electron microscope (Carl Zeiss Auriga-BU FIB FESEM) from the Joint School of Nanoscience and Nanoengineering, Greensboro, North Carolina, USA.
Escherichia coli 1946 (ATCC 25922), Staphylococcus aureus (ATCC 25923), klebsiella pneumoniae NCTC 9633 (ATCC 13883) and Micrococcus luteus (ATCC 4698) were obtained from the collection of the Department of Biological Sciences, Winston Salem State University. The isolates were checked for purity and maintained in slant of Nutrient agar (ThermoFisher Scientific, Waltham, MA, USA). Nutrient broth (NB) was used as the growth medium for the bacteria. Prior to the assays, the bacteria strains were cultured at 37 °C in 5 mL of NB media with shaking (120 rpm).
Antibacterial activities of both hind and forewings of honeybees were tested using Escherichia coli, Staphylococcus aureus, Klebsiella pneumoniae and Micrococcus luteus. These strains were cultured overnight in NB media (ThermoFisher Scientific, Waltham, MA, USA) in clear 96-well plates at a final volume of 200 µL for 24 h at 37 °C in a shaker (120 rpm). To start the twelve-row by eight-column plate was divided into 2 sections. One column was designated the “experimental” column and one was designated the “control”. With the help of a sterilized pair of forceps, each wing (intact wing) was meticulously transferred to a well. Then, 100 µL of NB media was placed into each cell of the experimental and control column down the 8 rows: negative control (media only (M)), positive control (media + cells (CM)), experimental well 1 (fore wing (FW) + media + cells), experimental well 2 (hind wings (H) + media + cells), experimental well 3 (hind wings + fore wings (FHW) + media + cells) and experimental well 3 (fore wing (FW) + media ). The assays were carried out in triplicate and bacterial growth was assessed by measuring turbidity at 600 nm for hours 0 and 24 h, using a 98-well plate format Glomaxmulti plate reader (Promega, USA). To determine the bacterial load (CFU/mL), cells from each treatment were collected after 24 hours of incubation. Samples were diluted 4 times with sterile NB and 10 µL of each dilution was spread on NA agar plates with the help of sterile L-Shaped cell spreaders. The plates were incubated at 37 °C for 24 hours. Colonies on each plate were then counted. The CFU/mL was calculated as CFU/mL = n° colonies x dilution factor). After 24 hours of incubation the forewings were aseptically removed from the 96-well plates for SEM as described above.
Statistical Analysis Statistical analyses were performed using GraphPad Prism version 7.00 (GraphPad software, La Jolla, USA). The antimicrobial activities were analyzed using Student's T-tests. Statistical differences between groups were considered significant at a p-value < 0.05.
The surface structures of the hind and fore wings of adult honeybee workers from the wild were extensively characterized by scanning electron microscope imaging technique. Forewing and hindwing surfaces were covered by an array of conical shaped pillars with sharp pointed ends on both dorsal and ventral surfaces (Figure 2). The array consisted of closely packed pillars with a base diameter 7.0 ± 0.25 µm, a height of 20.0 ± 2.22 µm, and spacing of 65.0 ± 3.41 µm apart from each other. Furthermore, the pillars were arranged in a manner similar to that of a parallelogram. Taken together these results show that honeybee workers' wings possess microscopic spikes that may defend against microbes.
According to Figure 3 microbial growth did not occur in the one of experimental wells (wings + media) suggesting self-cleaning or antibiofouling. Meanwhile, on the negative control (Cells + Media only) microbial growth was recorded. The results of the antimicrobial tests on the worker honeybee wings against bacteria showed that stronger antimicrobial activity was against the Gram-negative bacteria (E. coli and K. pneumoniae) than against the Gram-positive bacteria (S. aureus and M. luteus) (Figure 4). The fore wing (FW) was extremely effective at slowing the growth of Gram-negative bacteria when compared to Gram-positive samples. The presence of a single forewing significantly reduced the growth of E. coli and K. pnuemoniae when compared to the control samples (i.e., cells and media) (CM) (Figure 4A, 4B). In comparison, the hind wings showed no significant reduction in optical densities of E. coli and K. pnuemoniae (Figure 4A, 4B). This pattern was not observed for the Gram-positive bacterial cells. The hind wing significantly decreased the growth of S. aureus when compared to the fore wing (Figure 4C). The effects of the forewing and hind wing on the growth of M. luteus were comparable (Figure 4D). Finally, we assessed the bacterial growth in the presence of both hind and fore wings (FHW). We observed a statistically significant reduction in the growth of all the bacteria with a greater reduction in Gram-positive (E. coli and K. pneumoniae) (Figure 3).
For S. aureus, K. pneumoniae, E. coli; S. aureus, and M. luteus, significant reductions in CFU relative to controls were observed on the wings following 24-h incubation (Figure 4), corroborating the antimicrobial efficacy of a worker honeybee (Figure 3).
The bacterial cells were found to be capable of adhering effectively onto the surface of the honeybee fore wings; however, those cells that were able to attach to the surface assumed strange morphologies with extreme efficiency by the wing surface. The wing was able to punch holes in the cell membranes of Gram-negative E. coli cells (Figure 5A, B) while there is a morphology transition of gram-positive S. aureus from coccoid shape to rod shape (Figure 5C, D). This may be a survival strategy that evolved to help these bacteria survive in less-than-ideal conditions. Altogether, our results reveal that honeybee worker wings exhibit antibacterial properties against Gram-negative bacteria, E. Coli, K. pneumoniae and Gram-positive, S. aureus and M. luteus. Additionally, these wings are self-cleaning.
Some insects such as cicada, damselfly, and dragonfly are known to have antimicrobial and antibiofouling potential mediated by mediated by the physical nanoprotrusions (known as nanopillars) found on the wing surface [15],[31],[32]. To the best of our knowledge, there have been no studies that demonstrate the presence of array structures on the wings of honeybees that confer antibiofouling and antimicrobial activities. This is consequential because honeybees are arguably the most important managed species for agricultural pollination across the world.
In this study, we demonstrated that honeybee workers possess surface features that significantly increased antibiofouling and antimicrobial activities against the Gram-negative bacteria (E. coli and K. pneumoniae) and Gram-positive bacteria (S. aureus and M. luteus). Analysis of these wings using SEM showed highly ordered closely packed 20.0 µm tall spike structures, called “nanopillars”, that are spaced approximately 170 nm apart. The exact mechanisms on how nanopillars inhibit the growth of bacteria is yet to be understood and resolutions/answers have long tantalized researchers. However, recent studies involving titanium oxide suggest that the array and evenly distributed nanopillars on the wings of cicada and dragon flies exhibit antimicrobial activities by penetrating bacterial cells, causing the cellular membranes to stretch, and cells to rupture [33].
The microstructure or “nanopillars” of pristine honeybee worker wings contribute to rugged surface increasing the roughness of wings and the likelihood of anti-biofouling defined as self-cleaning ability to prevent contamination by particles such as dust, spores, and bacteria. Our investigations of the worker honeybee wings from the wild revealed that the wing surface was effective at killing or inhibiting the growth of adhered bacteria (Figure 3). Hydrophobic surfaces are famously known to inhibit the attachment of bacterial cells and there could be a relationship between hydrophobicity, surface roughness and antibiofouling that work in a concerted fashion to produce antimicrobial effects. Studies have shown that hydrophobic property associated with honeybee wings is attributed to the coupling of rough surface microstructure and hydrophobic protein constituents [34],[35]. The main constituent of the hydrophobic polypeptide belongs to the 60 S acidic ribosomal protein P1 and is reported to inhibit infectious bacteria due to an increase in intracellular reactive oxidative species (ROS), which, in turn, could affect membrane integrity and cause cell death [36],[37].
In this study, Gram-negative bacteria (K. pneumoniae, E. coli) were more susceptible to the bactericidal effect of the wings compared to Gram-positive bacteria (S. aureus, and M. luteus). These observations are consistent with reports made in previous literature that employed either naturally occurring bactericidal topographies or replicas derived from the natural counterparts. Gram-Positive bacteria are armed with rigid membranes, making them more resistant to the lethal effects of the wings while Gram-negative bacteria with more elastic membranes are more susceptible [38]. This provides further evidence in support of the assertion made in other studies using cicada wings that the bactericidal activity is mechanical in nature [13],[15] where cicada wings were more effective in killing Gram-negative bacteria (i.e., Branhamella catarrhalis, Escherichia coli, and Pseudomonas fluorescens) than Gram-positive bacteria (Bacillus subtilis, Pseudococcus maritimus, and Staphylococcus aureus) [13]. This suggests that the primary factor determining the susceptibility of cells to the wing surface was the rigidity of the cells.
Honeybee wing surfaces caused morphologic and structural variation in Gram-negative and Gram-positive bacteria. Damage to E. coli cells was characterized by holes in the membranes while S. aureus cells increased in length and transformed from a coccoid shape to rod shape. The holes in the E. coli cells were absent in S. aureus cells because of the presence a thick peptidoglycan which is known to confer strength and rigidity. An increase in length of S. aureus could be due to stress, which results in the production of ROS as observed in Lactobacillus spp, in M. tuberculosis, and M. smegmatis [39]–[41].
The nanoarchitecture and self-cleaning ability of the worker honeybee's wings serves lifestyle requirements, similar to migratory habits and foraging behavior. Honeybees have an organized system of dividing labor amongst each other in their societies [42]. In this paper, we will focus on the function of the workers' wings. The tasks delegated among the workers within a colony are based on the age of the individual and on the needs of the colony [43]. Immediately after emergence, juvenile workers clean cells and feed older larvae while mature worker bees perform indoor duties inside the hive [44],[45]. Thereafter, they become foragers, collecting water, pollen, nectar and propolis. The versatile activities of workers expose them to pathogens with significant effects such as paralysis, respiratory failure, and mortality of target (workers) and non-target (e.g., drones and queens) individuals. The A. mellifera workers need a highly adapted system as the tasks they perform during their lifespan expose them to distinct pressures from pathogenic exposure [46]. In general, the first line of defense against most pathogens is the cuticle, a preventive barrier designed to prevent or retard the entry of pathogens into the haemoceol [47],[48]. Like all insect body parts, the wings are made from cuticle, which is the second most common natural material in the world. In honeybees, the production of cuticle protein CPR14 plays important roles for cuticle maturation and maintenance, thus providing an effective protection against pathogens in adult workers [43],[49]. Therefore, the combination of the physical and chemical properties of the worker wings are efficient in creating a hostile niche for bacterial colonization and growth.
We have demonstrated evidence that worker honeybee wings exhibit a wide range of anti-biofouling and antimicrobial properties. The tested Gram-negative bacteria (Klebsiella pneumoniae, Escherichia coli) were more susceptible to the bactericidal effect of the wings compared to Gram-positive bacteria (Staphylococcus aureus, and Micrococcus luteus). These properties may be attributed primarily to a physical mechanism, but we have not ruled out any potential biochemical mechanisms. Topographical analysis of the wing surfaces showed regularly spaced conical shaped pillars with sharp points. The features of honeybee worker wings can inspire a new approach in the development of novel functional surfaces that possess an increased resistance to bacterial contamination and infection. These findings have a multitude of potential applications in industry, medical technology, clothing manufacturing, etc. Further study is needed to actualize these applications.
[1] |
Falcone PM, Lopolito A, Sica E (2017) Policy mixes towards sustainability transition in the Italian biofuel sector: Dealing with alternative crisis scenarios. Energy Res Soc Sci 33: 105-114. doi: 10.1016/j.erss.2017.09.007
![]() |
[2] |
Falcone PM (2018) Analysing stakeholders' perspectives towards a socio-technical change: The energy transition journey in Gela municipality. AIMS Energy 6: 645-657. doi: 10.3934/energy.2018.4.645
![]() |
[3] |
Kokkinos K, Karayannis V, Moustakas K (2020) Circular bio-economy via energy transition supported by Fuzzy Cognitive Map modeling towards sustainable low-carbon environment. Sci Total Environ 721: 1-16. doi: 10.1016/j.scitotenv.2020.137754
![]() |
[4] |
Okafor C, Ajaero C, Madu C, et al. (2020) Implementation of circular economy principles in management of end-of-life tyres in a developing country (Nigeria). AIMS Environ Sci 7: 406-433. doi: 10.3934/environsci.2020027
![]() |
[5] |
Thombs RP (2019) When democracy meets energy transitions: A typology of social power and energy system scale. Energy Res Soc Sci 52: 159-168. doi: 10.1016/j.erss.2019.02.020
![]() |
[6] | Owen R, Brennan G, Lyon F (2018) Enabling investment for the transition to a low carbon economy: Government policy to finance early stage green innovation. Submitted to Current Opinion in Environmental Sustainability Special Issue for the Intergovernmental Panel on Climate Change (IPCC) Sixth Assessment Cycle and Special Report on Global Warming. |
[7] | Kah M (2018) Electric vehicles and their impact on oil demand: Why forecasts differ. Center on Global Energy Policy, Columbia SIPA, 1-15. |
[8] | Stevens P (2019) The geopolitical implications of future oil demand, Research Paper. The Royal Institute of International Affairs: Chatham House, 1-42. |
[9] | Center for Sustainable Systems, University of Michigan (2016) Greenhouse Gases Factsheet. Pub. No. CSS05-21. Available from: https://css.umich.edu/factsheets/greenhouse-gases-factsheet |
[10] | IPCC (2013) Summary for policymakers. In: Stocker TF, Qin D, Plattner GK, et al. (Eds.), In: Climate Change 2013: The Physical Science Basis. Contribution of Working Group I to the Fifth Assessment Report of the Intergovernmental Panel on Climate Change. Cambridge University Press, Cambridge, United Kingdom and New York, NY, USA. |
[11] | Johnston RJ, Blakemore R, Bell R (2020) The role of oil and gas companies in the energy transition. Atlantic Council, Washington DC: USA, 1-44. |
[12] |
Maji IK (2015) Does clean energy contribute to economic growth? Evidence from Nigeria. Energy Rep 1: 145-150. doi: 10.1016/j.egyr.2015.06.001
![]() |
[13] |
Adewuyi OB, Kiptoo MK, Afolayan AF, et al. (2020) Challenges and prospects of Nigeria's sustainable energy transition. Energy Rep 6: 993-1009. doi: 10.1016/j.egyr.2020.04.022
![]() |
[14] |
Fouquet R (2016) Historical energy transitions: speed, prices and system transformation. Energy Res Soc Sci 22: 7-12. doi: 10.1016/j.erss.2016.08.014
![]() |
[15] | Oxford Institute for Energy Studies. The rise of renewables and energy transition: what adaptation strategy for oil companies and oil-exporting countries? (2018) OIES Paper: MEP 19. 1-25. Available from: https://www.oxfordenergy.org/publications/rise-renewables-energy-transition-adaptation-strategy-oil-companies-oil-exporting-countries/. |
[16] | Pistelli L (2020) Addressing Africa's energy dilemma. In: Hafner and Tagliapietra (eds), The geopolitics of the global energy transition, Lecture Notes in Energy 73: 151-174. |
[17] | Sovacool BK (2016) The history and politics of energy transitions: Comparing contested views and finding common ground. United Nations University World Institute for Development Economics Research, WIDER working paper 2016/81, Helsinki, Finland. |
[18] |
Zou C, Zhao Q, Zhang G, et al. (2016) Energy revolution: From a fossil fuel energy era to a new energy era. Nat Gas Ind B 3: 1-11. doi: 10.1016/j.ngib.2016.02.001
![]() |
[19] | Unger RW (2013) Energy transitions in history: Global cases of continuity and change. Rachel Carson Center Perspectives, 2013/2. |
[20] | Hafner M, Tagliapietra S (2020) The geopolitics of the global energy transition. In: Lecture Notes in Energy 73: 1-398. |
[21] | Bressand A (2012) The changed geopolitics of energy and climate and the challenge for Europe. A geopolitical and European perspective on the triple agenda of competition, energy security and sustainability. In: Clingendael international energy programme (CIEP), CIEP paper 04. |
[22] | REN21, Renewable Energy Policy Network for the 21st Century (2019) Renewables 2019 Global Status Report (Paris: REN21 Secretariat). ISBN 978-3-9818911-7-1. 1-336. |
[23] | IRENA (2018) Global energy transformation: A roadmap to 2050. International Renewable Energy Agency, Abu Dhabi. 1-76. ISBN 978-92-9260-059-4. |
[24] | Todd J, Thorstensen L (2013) Creating the clean energy economy: Analysis of the electric vehicle industry. International Energy Development Council, Washington DC: USA, 1-100. |
[25] | Global EV Outlook 2013, Global EV outlook: Understanding the electric vehicle landscape to 2020. Clean Energy Ministerial, Electric Vehicles Initiative and International Energy Agency. |
[26] | BP (2020) Statistical review of world energy 2020, 69th edition. Available from: https://www.bp.com/content/dam/bp/business-sites/en/global/corporate/pdfs/energy-economics/statistical-review/bp-stats-review-2020-full-report.pdf. |
[27] | IRENA, International Renewable Energy Agency (2016) Renewable energy benefits: Measuring the economics, Abu Dhabi: UAE, 1-92. |
[28] |
Endomah N, Foulds C, Jones A (2016) Energy transitions in Nigeria: The evolution of energy infrastructure provision (1800-2015). Energies 9: 484. doi: 10.3390/en9070484
![]() |
[29] | Porter G (2012) Reflections on a century of road transport developments in West Africa and their (gendered) impacts on the rural poor. EchoGeo 20: 1-16. |
[30] | Abioye O, Shubber K, Koenigsberger J (2016) Evaluating the role and impact of railway transport in the Nigerian economy, options and choices: Case of Nigerian Railway Corporation. AshEse J Econ 2: 103-113. |
[31] | Udosen C, Etok A-I, George IN (2009) Fifty years of oil exploration in Nigeria: The paradox of plenty. Glob J Soc Sci 8: 37-47. |
[32] | World Bank (2017) Nigeria federal roads development project (P090135) Report No: ICR00004170. |
[33] | EPA (2010) Landfill recovery and use in Nigeria (Pre-feasibility studies of using LFGE). Grant Number: XA83367801, US Environmental Protection Agency and Centre for People and Environment (CPE). |
[34] | US Energy Information Administration, Biomass explained: Waste-to-energy (Municipal solid waste), 2019. Available from: https://www.eia.gov/energyexplained/biomass/waste-to-energy.php. |
[35] | Clean technology investment plan for Nigeria: Updated Note, July 2014, 1-36. |
[36] | D'Adamo I, Falcone PM, Gastaldi M, et al. (2020) The economic viability of photovoltaic systems in public buildings: Evidence from Italy. Energy 207: 1-10. |
[37] | PwC, PriceWaterCoopers (2016), Nigeria: Looking beyond oil, 1-32. |
[38] | Raheem I (2016) Analysis of the effects of oil and non-oil export on economic growth in Nigeria. Hal-01401103v2. |
[39] | World Bank (2017) Nigeria Bi-annual Economic update: Fragile economy. The World Bank IBRD-IDA No. 1- April 2017. |
[40] | Oyekanmi S (2020) Updated: Nigerian economy grows by 2.27% in 2019, post highest quarterly growth since 2016 recession. Available from: https://nairametrics.com/2020/02/24/nigerian-economy-grows-by-2-27-post-highest-quarterly-growth-since-2016-recession/. |
[41] | World Bank (2020) Nigeria's economy faces worst recession in four decades, says new World Bank Report. Available from: https://www.worldbank.org/en/news/press-release/2020/06/25/nigerias-economy-faces-worst-recession-in-four-decades-says-new-world-bank-report. |
[42] | Auty RM (2001) Introduction and overview. In Auty RS (Ed.), Resource abundance and economic development (1st ed.), Oxford: Oxford University Press, 3-16. |
[43] | Rosser A (2006) The political economy of the resource curse: A literature survey. IDS Working Paper 268. |
[44] |
Roche MY, Verolme H, Agbaegbu C, et al. (2020) Achieving sustainable development goals in Nigeria's power sector: Assessment of transition pathways. Clim Policy 20: 846-865. doi: 10.1080/14693062.2019.1661818
![]() |
[45] | U.S. EIA, Energy Information Administration, (2020) Country analysis executive summary: Nigeria. 1-10. |
[46] | Okanlawon L (2015) The potential of Nigeria's residential solar rooftop systems. Available from: https://www.renewableenergyworld.com/storage/the-potential-of-nigerias-residential-solar-rooftop-systems/. |
[47] | Ministry of Mines and Steel Development, nd, Coal. Available from: https://www.minesandsteel.gov.ng/portfolio/coal/. |
[48] | Chukwu M, Folayan CO, Pam GY, et al. (2016) Characterization of some Nigerian coals for power generation. J Combust, 2016. |
[49] |
Ogunsola OI (1991) Coal production and utilization trends in Nigeria. Fuel Sci Tech Int 9: 1211-1222. doi: 10.1080/08843759108942323
![]() |
[50] | Index Mundi, nd, Nigeria coal production and consumption by year (Thousand short tons). Available from: https://www.indexmundi.com/energy/?country=ng&product=coal&graph=production+consumption. |
[51] | MGI, McKinsey Global Institute (2014) Nigeria's renewal: Delivering inclusive growth in Africa's largest economy. |
[52] | Dorian JP, Shealy MT, Simberk DR (2020) The global energy transition: Where do we go from here? IAEE Energy Forum/Second Quarter 2020: 11-18. |
[53] | World Bank, Nigeria's flaring reduction target: 2020 (2017). The World Bank IBRD-IDA. Available from: https://www.worldbank.org/en/news/feature/2017/03/10/nigerias-flaring-reduction-target. |
[54] | Index mundi (2014) Nigeria crude oil production and consumption by year (Thousand barrels per day). Available from: https://www.indexmundi.com/energy/?country=ng&product=oil&graph=production+consumption. |
[55] | Statista (2020) Oil production in Nigeria from 1998 to 2019. Available from: https://www.statista.com/statistics/265195/oil-production-in-nigeria-barrels-per-day/. |
[56] | TheGlobalEconomy.com (2020) Nigeria foreign direct investment, percent of GDP, chart. Available from: https://www.theglobaleconomy.com/Nigeria/Foreign_Direct_Investment/. |
[57] | UNCTAD, United Nations Conference on Trade and Development, (1999) World investment report 1999. United Nations, New York and Geneva. Available from: http://unct.ad.org/en/docs/wir1999_en.pdf. |
[58] |
Kareem SD, Kari F, Alam GM, et al. (2012) Foreign direct investment into oil sector and economic growth in Nigeria. Int J Appl Econ Finance 6: 127-135. doi: 10.3923/ijaef.2012.127.135
![]() |
[59] | Nordea (2020) Foreign direct investment (FDI) in Nigeria. Available from: https://www.nordeatrade.com/en/explore-new-market/nigeria/investment. |
[60] | Scholten D (ed) (2018) The geopolitics of renewables. Springer, New York. |
[61] | Spiegel (2018) Neuzulassungen: Erstmalsmehralseine Million E-Autos (New Registrations: First time more than one Million Electric Vehicles). Available from: http://www.spi'egel.de/auto/aktuell/emobilitaet-erstmals-ueber-eine-million-e-autoszugelassen-a-1197636.html. |
[62] | Couture TD, Leidreiter A (2014) How to achieve 100% renewable energy. World Future Council. Mexikoring 29, 22997 Hamburg, Germany, 1-60. |
[63] | Seplat Petroleum Development Company (2019) Nigeria's energy transformation: Special feature, Seplat Petroleum Development Company Plc Annual Report and Accounts 2019, 44-51. |
[64] | World Bank, Ecofys and Vivid Economics (2017) State and Trends of Carbon Pricing 2017 (November), by World Bank, Washington, DC. Doi: 10.1596/978-1-4648-1218-7License:CreativeCommonsAttributionCCBY3.0IGO. |
[65] | World Economic Forum (2014) Global energy architecture performance index: The new energy architecture challenge—Balancing the energy triangle. |
[66] | Ministry of Power, Federal Republic of Nigeria (2015) National Renewable Energy and Efficiency Policy (NREEEP) approved by FEC for the electricity sector. |
[67] | Falobi EO (2020) The role of renewable in Nigeria's energy policy mix. Int Assoc Energy Econ (IAEE) Energy Forum/First Quarter 2020: 41-46. |
[68] | Offgrid Nigeria (2020) CBN aims for $10m savings on import substitution for solar equipment. Available from: https://www.offgridnigeria.com/cbn-10m-savings-import-substitution-solar-equipment/. |
[69] |
Asumadu-Sarkodie S, Owusu PA (2016) A review of Ghana's solar energy potential. AIMS Energy 4: 675-696. doi: 10.3934/energy.2016.5.675
![]() |
[70] |
Akuru UB (2017) Towards 100% renewable energy in Nigeria. Renew Sustain Energy Rev 71: 943-953. doi: 10.1016/j.rser.2016.12.123
![]() |
[71] |
Esae OE, Sarah J, Mofe A (2020) A critical analysis of the role of energy generation from municipal solid waste (MSW). AIMS Environ Sci 7: 387-405. doi: 10.3934/environsci.2020026
![]() |
[72] | Bala-Gbogbo E (2012) Nigeria sovereign wealth fund to start investing in March. Available from: https://www.bloomberg.com/news/articles/2012-12-20/nigeria-sovereign-wealth-fund-to-start-investing-in-march. |
[73] | NSIA, Nigeria Sovereign Investment Authority, (2016) Available from: https://nsia.com.ng/investments. |
[74] | Eluhaiwe PN (2013) Government role in promoting renewable energy, Central Bank of Nigeria, 1-21. |
[75] | PwC (2019) X-raying the Nigerian palm oil sector, 1-8. Available from: https://www.pwc.com/ng/en/publications/x-raying-the-nigerian-palm-oil-sector.html. |
[76] | PwC (2016) Powering Nigeria for the future: The power sector in Nigeria July 2016. |
[77] | GBI (2013) Nigeria to spend $950 million on generator imports by 2020, viewed 10 July 2018. |
[78] | The Nation Online News, Nigeria is highest generator importer in Africa, 27 August, 2014. Available from: https://thenationonlineng.net//nigeria-is-highest-generator-importer-in-africa/. |
[79] | Central Bank of Nigeria (CBN) (2015) Analysis of energy market conditions in Nigeria' Occasional Paper No. 55. |
[80] | Greenstone M, Reguant M, Ryan N, et al. (2019) Energy and environment: Evidence paper. International Growth Center (IGC), London School of Economic and Political Science, Houghton Street, London WC2A 2AE, 1-44. |
[81] | Obafemi FN, Ifere EO (2013) Non-technical losses, energy efficiency and conservative methodology in the electricity sector of Nigeria: The case of Calabar, Cross River State. Int J Energy Econ Policy 3: 185-192. |
[82] | Drücke O (2018) Demand-Side Survey and Analysis of Mid-Sized Power Consumers in Nigeria. Let's Make Solar Work, A Nigerian-German Initiative: Lagos, Nigeria. |