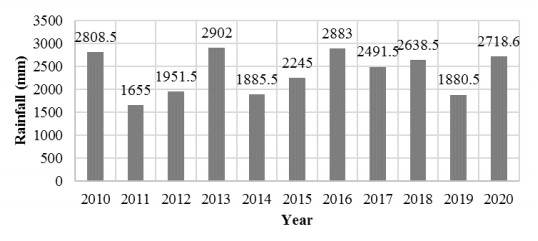
Lower bed single-row pineapple cultivation could protect pineapple from soil erosion during the rainy season and drought period; however, disease problems could arise as a result of water logging. Two experiments were conducted in Ultisol soil using a lower bed single row to investigate the ability of gypsum to provide soil calcium (Ca) to the pineapple plant, plant resistance to heart rot disease, and provide a better effect on crop growth and fruit quality. In the first trial, four levels of gypsum (0, 1.0, 1.5 and 2.0 Mg·ha−1) and dolomite (2 Mg·ha−1) were spread and incorporated into soil that had been saturated with Phytophthora nicotianae inoculums. In the second trial, gypsum treatments (0, 1.0, 1.5, 2.0 and 2.5 Mg·ha−1) were used as a basic fertiliser in the row between the single beds. P. nicotianae attacked all treatments at 6 weeks after planting (WAP), and at 10 WAP, the mortality of the dolomite treatment reached 63.8%, significantly higher than that of the gypsum treatments (3.3%–14.3%). In the second experiment, gypsum significantly increased plant weight from 3 to 9 months after planting (MAP), especially when applied at 1.5–2.5 Mg·ha−1. Fruit texture, total soluble solids (TSS), and titratable acidity (TA) were not significantly different between treatments, but they all met the standards for canned pineapple grades. The results showed that soil-applied gypsum before planting provides soil calcium, meets the plant Ca requirement during an early and fast growth stage, and is free of heart rot disease.
Citation: Supriyono Loekito, Afandi, Auliana Afandi, Naomasa Nishimura, Hiroyuki Koyama, Masateru Senge. Gypsum supplies calcium to Ultisol soil and its effect on Phytophthora nicotianae, pineapple (Ananas comosus) growth, yield and fruit quality in lower single row bed under climate change issue[J]. AIMS Agriculture and Food, 2022, 7(3): 721-736. doi: 10.3934/agrfood.2022044
[1] | Dinh Thi Hong Thanh, Yu Kaung Chang, Son Zuang Chen, Hsiao Dao Chang . Improve pineapples growth by nano-membranes accessory and under stress condition in far north of Taiwan. AIMS Agriculture and Food, 2021, 6(3): 799-817. doi: 10.3934/agrfood.2021049 |
[2] | Yenni, Mohd Hafiz Ibrahim, Rosimah Nulit, Siti Zaharah Sakimin . Influence of drought stress on growth, biochemical changes and leaf gas exchange of strawberry (Fragaria × ananassa Duch.) in Indonesia. AIMS Agriculture and Food, 2022, 7(1): 37-60. doi: 10.3934/agrfood.2022003 |
[3] | Gregorio Gullo, Antonio Dattola, Vincenzo Vonella, Rocco Zappia . Performance of the Brasiliano 92 orange cultivar with six trifoliate rootstocks. AIMS Agriculture and Food, 2021, 6(1): 203-215. doi: 10.3934/agrfood.2021013 |
[4] | Soukaina Ouansafi, Fahde Abdelilah, Mostafa Kabine, Hind Maaghloud, Fatima Bellali, Karima El Bouqdaoui . The effects of soil proprieties on the yield and the growth of tomato plants and fruits irrigated by treated wastewater. AIMS Agriculture and Food, 2019, 4(4): 921-938. doi: 10.3934/agrfood.2019.4.921 |
[5] | Saowanee Wijitkosum, Preamsuda Jiwnok . Effect of biochar on Chinese kale and carbon storage in an agricultural area on a high rise building. AIMS Agriculture and Food, 2019, 4(1): 177-193. doi: 10.3934/agrfood.2019.1.177 |
[6] | Christos Dramalis, Demetrios Katsantonis, Spyridon D. Koutroubas . Rice growth, assimilate translocation, and grain quality in response to salinity under Mediterranean conditions. AIMS Agriculture and Food, 2021, 6(1): 255-272. doi: 10.3934/agrfood.2021017 |
[7] | Gullo Gregorio, Dattola Antonio, Zappia Rocco . Comparative study of some fruit quality characteristics of two of Annona cherimola Mill. grown in southern Italy. AIMS Agriculture and Food, 2019, 4(3): 658-671. doi: 10.3934/agrfood.2019.3.658 |
[8] | Haile Desmae, Dramane Sako, Djeneba Konate . Impact of earthing up on groundnut (Arachis hypogaea L.) pod yield and its components. AIMS Agriculture and Food, 2022, 7(3): 614-622. doi: 10.3934/agrfood.2022038 |
[9] | Nono Carsono, Faza A. Maulana, Iqbal F. Elfakhriano, Ade Ismail, Noladhi Wicaksana, Santika Sari, Hiroshi Ezura . The comparative analysis of agronomic, compositional, and physiological traits of miraculin transgenic tomato in the confined field trial. AIMS Agriculture and Food, 2023, 8(1): 187-197. doi: 10.3934/agrfood.2023010 |
[10] | Stefano Puccio, Anna Perrone, Giuseppe Sortino, Giuseppe Gianguzzi, Carla Gentile, Vittorio Farina . Yield, pomological characteristics, bioactive compounds and antioxidant activity of Annona cherimola Mill. grown in mediterranean climate. AIMS Agriculture and Food, 2019, 4(3): 592-603. doi: 10.3934/agrfood.2019.3.592 |
Lower bed single-row pineapple cultivation could protect pineapple from soil erosion during the rainy season and drought period; however, disease problems could arise as a result of water logging. Two experiments were conducted in Ultisol soil using a lower bed single row to investigate the ability of gypsum to provide soil calcium (Ca) to the pineapple plant, plant resistance to heart rot disease, and provide a better effect on crop growth and fruit quality. In the first trial, four levels of gypsum (0, 1.0, 1.5 and 2.0 Mg·ha−1) and dolomite (2 Mg·ha−1) were spread and incorporated into soil that had been saturated with Phytophthora nicotianae inoculums. In the second trial, gypsum treatments (0, 1.0, 1.5, 2.0 and 2.5 Mg·ha−1) were used as a basic fertiliser in the row between the single beds. P. nicotianae attacked all treatments at 6 weeks after planting (WAP), and at 10 WAP, the mortality of the dolomite treatment reached 63.8%, significantly higher than that of the gypsum treatments (3.3%–14.3%). In the second experiment, gypsum significantly increased plant weight from 3 to 9 months after planting (MAP), especially when applied at 1.5–2.5 Mg·ha−1. Fruit texture, total soluble solids (TSS), and titratable acidity (TA) were not significantly different between treatments, but they all met the standards for canned pineapple grades. The results showed that soil-applied gypsum before planting provides soil calcium, meets the plant Ca requirement during an early and fast growth stage, and is free of heart rot disease.
The pineapple is one of the most commercially important tropical fruit in the world. Currently, the PT Great Giant Pineapple (GGP) plantation in Lampung, Sumatera, is the world's largest single pineapple plantation. The humid tropical climate has an annual rainfall of 2,500 mm, an average temperature of 21 to 33 ℃, 83% relative humidity, 4.6 hours of sunshine per day, and a standard evaporation rate of 36 mm/day. The humid tropics' year-round temperatures, heavy rainfall, and high humidity cause organic material in the soil to decompose at a rapid rate, resulting in low chemical fertility, a high clay content, and low soil pH. The soil is classified as Red-Yellow Podzolic or Ultisol soil [1]. This soil covers a large part of Indonesia's land surface, approximately 45,794,000 ha (25%), with approximately 2% of that land (9,469,000 ha) located on Sumatera Island [2].
During the last ten years, the unpredictable rainfall caused pineapple cultivation to face several challenges, particularly during the long dry season. The "wet dry season", in which the amount of rainfall fell during the dry season, occurred five times in the last ten years from 2010–2020, while the long dry season occurred four times (Figure 1). In this condition, pineapple cultivation is hampered by high rainfall, soil erosion, low soil fertility, and soil-borne disease. Pineapple is commonly planted in a raised bed double row (Figure 2A). High rainfall damaged bed shape, and disturbing roots and plants during pineapple growth are common causes of erosion. At the same time, it is vulnerable to drying and water logging conditions. In this study, pineapple cultivation in a lower bed single row (Figure 2B) was used, so the roots were not disturbed, were stronger, and were more resistant to drying than the raised bed, which faced the challenge of soil-borne disease due to poor drainage.
Aside from the recent unpredictability of weather patterns, another issue in a humid tropical climate was the availability of calcium (Ca) in the soil [2]. To manage the 32,000 ha pineapple plantation of GGP was divided into blocks, 10 to 15 ha each. When pineapple cultivation began in 1986, the soil Ca content in all plantation blocks ranged from 206 to 434 mg·kg−1, which was higher than the standard soil Ca adequate of 100 mg·kg−1 [3]. Nonetheless, soil conditions have deteriorated to the point where in 1993, 90% of the blocks had soil Ca content greater than the standard, and in 2004, only 60% of the blocks met that standard. The GGP plantation's soil pH ranges from 3.7–5.9, whereas the optimum soil pH for cultivated pineapple ranges from 4.7–5.5 [4].
Calcium is an essential nutrient and the primary cation responsible for soil pH management. It is required for the formation of a new cell wall, particularly the middle lamellae that separate the new cell during cell division, as well as the normal function of plant membranes [5]. Calcium is also essential for nutrient balance in plants and soil [6]. Ca deficiency is linked to a variety of physiological disorders in fruits and vegetables [7]. Ca availability has been found low in a humid tropical climate with high rainfall and soil leaching due to the soil's low pH and cation exchange capacity (CEC) [8]. In pineapple cultivation, however, increasing soil pH above 5 promotes diseases such as heart and root rot disease caused by Phytophthora sp. [9,10,11]. On the other hand, if the pH is extremely low, macronutrient availability will be limited.
Soil with less than 25 mg·kg−1 Ca content can cause calcium deficiency symptoms in the pineapple plant and fruit [3]. Necrosis of the edges of rapidly growing young leaves, as well as multiple small fruits, and sometimes deformed fruits, are symptoms of calcium deficiency [12]. Dolomite lime is commonly applied to fields to raise soil pH to at least 4.7 while also providing Ca and Mg. When the soil pH is relatively high, gypsum, in addition to dolomite, could supply Ca. Large amounts of gypsum will not raise the pH of the soil [13]. Gypsum, as a Ca source, could boost avocado root disease resistance and act as a mild fungicide by inhibiting the formation of Phytophthora spores [14]. P. cinnamomi zoospore production was reduced by 78% in soil treated with 1% gypsum, and the number of zoospores was reduced by 74% in soil treated with 5% gypsum [15]. At the end of the pineapple crop cycle, soil management with millet coverage and gypsum would allow adequate nutrients in the soil [16].
The purpose of the present study was to determine the effects of soil application of gypsum as a substitute for dolomite in supplying calcium in the pineapple plant and soil and to know the effects of soil application of Ca gypsum on the root system, crop growth, fruit quality, and P. nicotianae disease incidence of pineapple cultivated in Ultisol soil under lowered single bed system. The general aim was to meet Ca plants' requirements while reducing the risk of diseases.
Between 2016 and 2018, two field experiments were carried out at the research station in a pineapple field of the GGP plantation, located at latitude 04°49'13" South and longitude 105°13'13" East.
A randomized complete block design with five treatments and three replications was used in the experiment. Before September 2016, experimental plots were prepared in a Phytophthora spp. endemic area. The soil in the experimental plots was then saturated with P. nicotianae inoculums [17,18] by watering the soil with a solution prepared by soaking infected plants. The soil was tilled to incorporate the liming treatment after the treatments were applied. Table 1 shows the initial soil properties that were analyzed. Treatment G0 (untreated), G1 (1.0 and 233), G2 (1.5 and 349), G3 (2.0 and 465) of gypsum amendments, and D1 (2.0 and 440) of dolomite amendment in Mg·ha−1 and Ca in kg·ha−1 were use in the experiment. A week before planting in December 2016, gypsum was spread and incorporated into the soil. Gypsum (phosphogypsum) is a calcium sulfate hydrate obtained as a byproduct of the production of phosphoric acid, which is a solid waste from the extraction of P2O5 content from phosphate rock. Sulfuric acid attacks phosphate rock, producing phosphoric acid (H3PO4) and phosphate fertilizers. During land preparation in the plots two months before planting in October 2016, the dolomite treatment was spread and incorporated into the soil.
Properties | Unit | Experiment Ⅰ | Experiment Ⅱ | Fine compost | |
At planting (Dec. 2016) | Initial soil (May. 2017) | At planting (Sep. 2017) | |||
pH | – | 4.6 | 4.2 | 4.4 | 7.2 |
P | mg·kg−1 | 17.7 | 21.2 | 22.9 | 2.6 |
K | mg·kg−1 | 86.4 | 68.5 | 75 | 18.13 |
Ca | mg·kg−1 | 314.4 | 60.3 | 127.8 | 21.5 |
Mg | mg·kg−1 | 98.6 | 49.1 | 60.8 | 5.26 |
Cu | mg·kg−1 | Not analysed | 0.6 | 0.6 | Not analysed |
Soil Organic C | Wt% | 1.2 | 1.4 | 1.3 | 21.6 |
Total N | Wt% | Not analysed | Not analysed | Not analysed | 1.68 |
C/N ratio | – | Not analysed | Not analysed | Not analysed | 12.86 |
Note: Liming dolomite 1 Mg·ha−1 was done in June 2017 (Exp. 2). |
Each plot contained at least 200 plants in ten single row beds spaced 55 cm apart with a row spacing of 27 cm (equivalent to 67,340 plants/ha). To avoid unwanted interaction, the plots were separated by four border rows, and they were planted and maintained following standard plantation practices. Before planting, 100 kg·ha−1 of magnesium sulphate monohydrate (MgSO4·H2O) was applied to the soil as a basic fertilizer along with diammonium phosphate (DAP) and potassium chloride (KCl). In December 2016, selected medium size crowns of 'Smooth Cayenne' clone GP3 (250–350 g in weight) were planted.
Plant mortality caused by Phytophthora nicotianae was measured in each plot by counting the dead plants at 4, 6, 8 and 10 WAP, because the dead pineapple plant attacked by P. nicotianae (heart rot disease) can change daily. The percentage of plant mortality (disease incidence) was calculated using the following formula:
(1) |
Following the plant mortality assessment, the pH (H2O) of the soil was measured using a pH meter-Mettler Toledo at 0, 4 and 10 weeks after planting. The Ca and Mg concentrations in the soil were determined using atomic absorption spectrophotometry (AAS), neutralized with 1N acetic acid at pH 7.
A randomized complete block design with six treatments and four replications was used in the experiment. Soil gypsum treatments were applied in the row between the single bed as a basic fertilizer before planting along with KCl (200 kg·ha−1), DAP (250 kg·ha−1), MgSO4·H2O (300 kg·ha−1), CuSO4 (10 kg·ha−1), Borax (10 kg·ha−1) and Fine Compost (4.0 Mg·ha−1). The gypsum (G) treatment in Mg·ha−1 and kg·ha−1 Ca were G0 (Untreated and 0), G1 (0.5 and 116), G2 (1.0 and 233), G3 (1.5 and 349), G4 (2.0 and 465) and G5 (2.5 and 440). The initial and at planting soil properties were analysed (Table 1). For both experiments, the fields were irrigated with a gun sprinkle every 10 days with a volume of around 25–30 mm·ha−1.
Soil pH was initially 4.2, but 1000 kg·ha−1 of Dolomite lime was applied in June 2017, raising the pH to 4.4 at planting in September 2017 (Table 1). Soil properties (pH, P, K, Ca, Mg, Cu, total C and total N) were measured before and during planting. Ca and Mg levels in the soil and leaf were measured at 2, 3, 6, 7 and 9 MAP. At 3, 7, 9 and 11 MAP, the length of the longest leaf with a leaf angle of 45° from the soil surface (D-leaf) and plant weight were measured destructively. At 3 MAP, root length, total root number, fresh weight, and dry weight were measured. At harvest, plant, stem, fruit, and crown weights, as well as fruit and crown size distribution, were measured. Total soluble solids (TSS), titratable acidity (TA), TSS/TA ratio, and fruit texture were measured at harvest.
The soil nutrients were analyzed using the following methods: (a) pH with a pH meter-Mettler Toledo; (b) C organic with the Walkey and Black method; (c) P with the P Bray method and spectrometer reading; (d) K, Ca, and Mg were analyzed using acetic acid pH 7 extraction and AAS reading; (e) extraction and AAS reading; (f) N using Kjeldahl methods.
D-leaf was subjected to leaf analysis. To remove the upper leaves, one-third of the sample was cut. The leaves were then cut up and divided into two sections. The green portion was used for micronutrient analysis, while the white portion was used for macronutrient analysis. The samples were then dried in an oven at 70 ℃ for 24 hours. The dry leaf samples were ground and sieved with a 0.5 mm sieve. Extraction was done using HNO3 and H2O2, and destruction was done at a temperature of 175 ℃.
Each treatment plot was sampled for D-leaf. D-leaf is the longest leaf on the pineapple plant, with a leaf angle of 45 degrees from the soil surface. A ruler is used to measure the length of the D-leaf from bottom to top. The root samples were collected by circling each plant with a steel ring 54.5 cm in diameter and 25 cm in height, then carefully watering the soil to separate the soil and the rhizosphere. A ruler was used to measure the longest root length from the stem. The number of roots that sprouted from the stem was counted. The roots were separated from the basal stem, air-dried at room temperature to remove excess moisture, and weighed to determine their fresh weight. The roots were then oven-dried to a constant weight at 105 ℃.
The fruit flesh was cut into small pieces (excluding the skin, core, and top and bottom 2 cm of the fruit), and the juice was extracted. The filtrate was measured using a hand-held refractometer (MASTER-53; Atago, Japan) to determine the TSS content. Titration to pH 8.1 with 0.1 M NaOH using phenolphthalein as an indicator revealed the percentage of citric acid. A Brookfield Ametex CT3 Texture Analyzer, a compression, tension testing tool for rapid quality control analyses, was used to measure fruit texture at three-point regions of fruit slices taken from the top, middle, and bottom sections. TA5 was the fixture used (a cylindrical probe 12.7 mm in diameter and 35 mm in length). To get fruit size distribution, fruit size was determined by measured the fruit diameter: (a) < 1T diameter < 9.9 cm; (b) 1 T diameter 9.9–10.5 cm; (c) 1 3/8 T diameter 10.6–11.5 cm; (d) 2 T diameter 11.6–12.9 cm; (e) 2 ½ T diameter > 12.9 cm. Crown size distribution was made by measured the crown weight: (a) Extra small is < 150 g; (b) Small is 150–200 g; (c) Medium is 201–350 g; (d) Big is 351–450 g; (e) Extra big is > 450 g. The collected data were analyzed using an analysis of variance (ANOVA), and the means were compared with the Tukey test with a difference of 95% (P < 0.05).
The results showed that plant mortality was higher in the D (dolomite) treatment than in the G (gypsum) treatment (Table 2). For treatment D1, the shortest time from planting to significant numbers of plants showing disease symptoms was four weeks (Table 2). Plant mortality for the dolomite treatment was 54.3% at 8 WAP and reached 63.8% at 10 WAP, while plant mortality for the G treatments ranged from 3.3%–14.3% at 10 WAP; the differences were not significant.
Treatment | Plant mortality (%) | |||
4 WAP | 6 WAP | 8 WAP | 10 WAP | |
G0 (Untreated) | 0.0 a | 1.3 a | 2.7 a | 3.3 a |
G1 (Gypsum 1.0 Mg·ha−1) | 0.0 a | 4.7 a | 9.3 a | 10.3 a |
G2 (Gypsum 1.5 Mg·ha−1) | 0.0 a | 4.3 a | 7.0 a | 8.7 a |
G3 (Gypsum 2.0 Mg·ha−1) | 0.3 a | 7.3 ab | 12.0 a | 14.3 a |
D1 (Dolomite 2.0 Mg·ha−1) | 0.7 a | 24.3 b | 54.3 b | 63.8 b |
P-value | 0.12 | 0.01 | 0 | 0 |
Note: Means within a column followed by a common letter are not significantly different (P < 0.05) according to the Tuckey test of difference. |
As shown in Table 3, the pH value of the dolomite treatment (D1) was 5.9 at 10 WAP, whereas the pH values of the gypsum treatments were still low, ranging from 4.5 to 4.7. The calcium contents showed significant differences between treatments, particularly after 10 WAP. Treatments with gypsum and dolomite outperformed untreated controls by a wide margin. Untreated soil had a calcium content that was 50 mg·kg−1 lower on average than the other soil treatments used. Significant differences in magnesium concentrations were observed after 4 WAP. The higher the gypsum dose, the more calcium was available in the soil. Furthermore, after 10 WAP, the available magnesium in the soil decreased, with the soils treated with dolomite having the most reduced results (D1).
Treatment | Soil pH | Ca (mg·kg−1) | Mg (mg·kg−1) | ||||||
0 WAP | 4 WAP | 10 WAP | 0 WAP | 4 WAP | 10 WAP | 0 WAP | 4 WAP | 10 WAP | |
G0 | 4.6 | 5.1 a | 4.5 a | 314 | 430 a | 311 a | 99 | 137 ab | 75 ab |
G1 | 4.6 | 5.3 ab | 4.6 a | 314 | 493 a | 379 ab | 99 | 156 ab | 77 ac |
G2 | 4.6 | 5.3 ab | 4.7 a | 314 | 489 a | 400 b | 99 | 146 a | 98 ac |
G3 | 4.6 | 5.0 a | 4.7 a | 314 | 448 a | 389 b | 99 | 120 b | 62 b |
D1 | 4.6 | 5.5 b | 5.9 b | 314 | 467 a | 369 ab | 99 | 162 c | 159 c |
P-value | – | 0 | 0 | – | 0.61 | 0.02 | – | 0 | 0 |
Note: Means within a column followed by a common letter are not significantly different (P < 0.05) according to the Tuckey test of difference. |
The calcium content of the soil was affected by gypsum application until 6 months after planting and then declined. As shown in Figure 3, untreated plants had less Ca available at most stages after planting studied in this study. Furthermore, it is possible to see in Figure 3 that there are no significant differences in soil magnesium content between 0 and 9 MAP.
Table 4 shows the effect of gypsum soil application on plant growth, while Table 5 shows root response. The application had no statistically significant effect on D-Leaf length at 3, 7, 9 and 11 MAP, but it significantly increased plant weight from 3 to 9 MAP. At 11 MAP, there was no significant difference in plant weight between treatments. The calcium content in the leaf peaked after 7 MAP, as shown in Figure 4, and the untreated soils provided the lowest calcium content to the leaf throughout all experiments. However, Figure 4 demonstrated how the magnesium content in the leaf reached a high value in all treatments at 7 MAP, as did the calcium; however, in this case, the untreated soil delivered the highest content at 9 MAP. According to this figure, the gypsum could have a negative impact on the magnesium in the leaf for as long as the plant grows.
Treatment | D-Leaf length (cm) | Plant weight (g) | ||||||
3 MAP | 7 MAP | 9 MAP | 11 MAP | 3 MAP | 7 MAP | 9 MAP | 11 MAP | |
G0 (Untreated) | 51.2 a | 75.9 a | 87.2 a | 95.1 a | 201.3 a | 842.5 a | 1336.3 a | 2546.3 a |
G1 (0.5 Mg·ha−1) | 51.0 a | 75.8 a | 88.1 a | 97.4 a | 215.0 ab | 1032.5 a | 1606.3 ab | 2592.5 a |
G2 (1.0 Mg·ha−1) | 53.1 a | 77.4 a | 89.4 a | 96.5 a | 222.5 ab | 901.3 ab | 1450.0 ab | 2772.5 a |
G3 (1.5 Mg·ha−1) | 51.9 a | 76.4 a | 89.0 a | 97.2 a | 207.5 ab | 1021.3 b | 1736.3 b | 2598.8 a |
G4 (2.0 Mg·ha−1) | 53.6 a | 78.1 a | 90.2 a | 104.7 a | 210.0 ab | 1002.5 ab | 1698.8 ab | 2722.5 a |
G5 (2.5 Mg·ha−1) | 54.1 a | 76.6 a | 88.7 a | 96.2 a | 225.0 b | 1037.5 b | 1725.0 ab | 2520.0 a |
P-value | 0.56 | 0.94 | 0.91 | 0.36 | 0.02 | 0.01 | 0.02 | 0.66 |
Note: Means within a column followed by a common letter are not significantly different (P < 0.05) according to Tuckey test of difference. |
Treatment | Root length (cm) | Total root number | Root fresh weight (cm) | Root dry weight (cm) |
G0 (Untreated) | 43.1 a | 38.9 a | 5.3 a | 2.5 a |
G1 (0.5 Mg·ha−1) | 41.8 a | 29.9 a | 5.8 a | 2.7 a |
G2 (1.0 Mg·ha−1) | 45.1 a | 35.8 a | 5.3 a | 2.6 a |
G3 (1.5 Mg·ha−1) | 41.9 a | 32.5 a | 5.3 a | 2.4 a |
G4 (2.0 Mg·ha−1) | 46.3 a | 34.7 a | 4.5 a | 2.1 a |
G5 (2.5 Mg·ha−1) | 43.9 a | 36.3 a | 6.5 a | 3.3 a |
P-value | 0.82 | 0.16 | 0.25 | 0.1 |
Note: Means within a column followed by a common letter are not significantly different (P < 0.05) according to the Tuckey test of difference. |
Gypsum application had no effect on root length, total root number, root fresh and dry weight (Table 5). Nonetheless, in terms of average values across all treatments, the soil treated with 2.5 Mg·ha−1 had the highest root weight values (G5). This suggested that gypsum could have a greater impact on these variables than the root length and number.
Fruit, crown, stem, and plant weight at harvest were higher in all gypsum treatments than in the untreated one, but the differences were not statistically significant (Table 6). In this case, while 0.5 Mg·ha−1 rate resulted in the highest average value for plant, stem, and crown weight at harvest, 1.0 Mg·ha−1 rate resulted in the highest fruit weight at harvest.
Treatment | Plant weight at harvest (g) | Stem weight at harvest (g) | Fruit weight (g) | Crown weight (g) |
G0 (Untreated) | 2451.3 a | 520.0 a | 1455.0 a | 322.5 a |
G1 (0.5 Mg·ha−1) | 2908.3 a | 607.5 a | 1497.5 a | 402.5 a |
G2 (1.0 Mg·ha−1) | 2730.0 a | 590.0 a | 1731.3 a | 365.0 a |
G3 (1.5 Mg·ha−1) | 2645.0 a | 580.0 a | 1467.5 a | 330.0 a |
G4 (2.0 Mg·ha−1) | 2647.5 a | 532.5 a | 1677.5 a | 335.0 a |
G5 (2.5 Mg·ha−1) | 2527.3 a | 537.5 a | 1632.5 a | 345.0 a |
P-value | 0.41 | 0.59 | 0.13 | 0.51 |
Note: Means within a column followed by a common letter are not significantly different (P < 0.05) according to the Tuckey test of difference. |
Table 7 shows the TSS, TA, TSS/TA ratio, and fruit texture (firmness) of various fruits. TSS, TA and fruit texture did not differ significantly between treatments, but all gypsum treatments had higher firmness values than the untreated, both in-shell colours 1 and 3. Tables 8 and 9 showed the distribution of fruit size and crown size at harvest. All Ca gypsum treatments had the potential to increase the number of large fruits (size 2 T and 2 12 T). Based on the average values, 0.5 Mg·ha−1 of gypsum can provide the fruit with the greatest diameter at harvest when compared to higher doses of this fertilizer and the untreated treatment. Meanwhile, 2.5 Mg·ha−1 of gypsum can produce a higher crown weight than the doses used and the untreated soil treatment.
Treatment | Fruit TSS (oBrix) | Fruit TA (%) | TSS/TA ratio | Fruit texture (firmness) | |||||
Shell colour 1 (10% yellow) | Shell colour 3 (20%–30% yellow) | ||||||||
Top (g) | Middle (g) | Bottom (g) | Top (g) | Middle (g) | Bottom (g) | ||||
G0 (Untreated) | 13.5 a | 0.55 a | 24.5 | 282.8 a | 282.2 a | 278.3 a | 230.6 a | 261.7 a | 230.6 a |
G1 (0.5 Mg·ha−1) | 12.6 a | 0.49 a | 25.6 | 296.7 a | 298.3 a | 282.2 a | 267.2 a | 267.2 a | 267.2 a |
G2 (1.0 Mg·ha−1) | 12.1 ab | 0.46 a | 26.2 | 283.3 a | 289.4 a | 281.7 a | 257.8 a | 277.2 a | 257.8 a |
G3 (1.5 Mg·ha−1) | 12.7 a | 0.53 a | 23.9 | 326.1 a | 312.8 a | 282.2 a | 262.8 a | 279.4 a | 262.8 a |
G4 (2.0 Mg·ha−1) | 12.0 ab | 0.50 a | 23.9 | 281.1 a | 289.4 a | 299.4 a | 254.4 a | 267.8 a | 254.4 a |
G5 (2.5 Mg·ha−1) | 13.3 a | 0.52 a | 25.6 | 285.6 a | 292.2 a | 303.3 a | 293.9 a | 275.6 a | 293.9 a |
P-value | 0.045 | 0.14 | – | 0.916 | 0.72 | 0.844 | 0.431 | 0.977 | 0.121 |
Note: Means within a column followed by a common letter are not significantly different (P < 0.05) according to the Tuckey test of difference. |
Treatment | Fruit size distribution (%) | |||||
< 1T | 1 T | 1 3/8 T | 2 T | 2 1/2 T | (2 T + 2 1/2 T) | |
G0 (Untreated) | 3.7 a | 12.0 a | 15.4 a | 26.0 a | 43.0 a | 68.9 |
G1 (0.5 Mg·ha−1) | 3.0 a | 8.2 a | 10.7 a | 31.2 a | 46.8 a | 78 |
G2 (1.0 Mg·ha−1) | 2.8 a | 8.0 a | 15.6 a | 33.1 a | 40.5 a | 73.6 |
G3 (1.5 Mg·ha−1) | 2.2 a | 8.2 a | 15.1 a | 28.5 a | 46.1 a | 74.5 |
G4 (2.0 Mg·ha−1) | 4.5 a | 8.4 a | 13.1 a | 30.8 a | 43.1 a | 73.9 |
G5 (2.5 Mg·ha−1) | 1.7 a | 11.4 a | 14.8 a | 30.7 a | 41.4 a | 72.1 |
P-value | 0.601 | 0.771 | 0.707 | 0.863 | 0.588 | – |
Note: Means within a column followed by a common letter are not significantly different (P < 0.05) according to the Tuckey test of difference. |
Treatment | Crown size distribution (%) | |||||
Extra small | Small | Medium | Big | Extra big | (Big + Extra big) | |
G0 (Untreated) | 0.3 a | 3.2 a | 60.1 a | 33.2 a | 3.2 a | 36.4 |
G1 (0.5 Mg·ha−1) | 0.0 a | 0.0 a | 40.1 a | 54.2 a | 5.7 a | 59.9 |
G2 (1.0 Mg·ha−1) | 0.0 a | 3.0 a | 39.3 a | 54.0 a | 3.7 a | 57.7 |
G3 (1.5 Mg·ha−1) | 0.0 a | 1.7 a | 43.9 a | 52.6 a | 1.8 a | 54.4 |
G4 (2.0 Mg·ha−1) | 0.0 a | 3.3 a | 40.8 a | 54.4 a | 1.5 a | 56.9 |
G5 (2.5 Mg·ha−1) | 0.0 a | 3.7 a | 35.0 a | 56.0 a | 5.7 a | 61.7 |
P-value | 0.458 | 0.895 | 0.433 | 0.346 | 0.691 | – |
Note: Means within a column followed by a common letter are not significantly different (P < 0.05) according to the Tuckey test of difference. |
The current experiment's goals were to assess the effect of gypsum soil application on hearth rot disease caused by P. nicotianae as well as the effect on soil and leaf nutrition, plant response, and pineapple fruit quality. P. nicotianae attacked pineapple plants in all treatments six weeks after planting when the soil pH exceeded 5.0 due to high rainfall from December 2016 to March 2017, namely 270,369,352 and 418 mm per month, respectively. As the pH of the soil increased, so did the mortality rate. When the soil pH was 5.9 ten weeks after planting, the mortality with treatment D1 reached 63.8% when the soil pH was 5.9. This is significantly different from the G treatment (3.3%–14.3%), which has a soil pH level of 4.5–4.7.
Increasing the amount of gypsum did not consistently raise the pH of the soil (Table 1). Plant mortality was low for treatments G0 through G3 ten weeks after planting and significantly different from treatment D1. This demonstrates that maintaining a low soil pH (treatment G0) and adding Ca with gypsum (treatments G1–G3) are more beneficial than using dolomite (treatment D1). Soil amendment with gypsum inhibited sporangium formation in P. parasitica in a way that lime, hydrated lime, or dolomite lime could not [19]. As a result of fungal attacks, calcium ions may stimulate a phytoalexin, a compound known to be involved in plant defence mechanisms [20].
The results also revealed that ten weeks after planting, the soil Ca contents in treatments G2, and G3 were significantly higher than in untreated soil (Table 1). Gypsum has a moderate water solubility (2.5 g·L−1) that is approximately 200 times greater than lime [21]. This makes the Ca in gypsum more mobile than the Ca in dolomite lime, allowing it to move more easily through the soil profile. A magnesium application is required when the initial Mg content of the soil is less than 50 mg·kg−1 [22]. In the current experiment, the initial Mg content was 99 mg·kg−1, and kieserite fertiliser was applied at planting time along with DAP and KCl to keep the Mg content in the soil at or above 50 mg·kg−1. Despite this, the Mg content in the soil increased to 162 mg·kg−1 after eight months and decreased to 159 mg·kg−1 with D1 higher than all G treatments ten weeks after the pineapples were kept on the GGP fertilizer regime. Phytophthora can be suppressed by magnesium, according to Nome et al. [23], by influencing how the pathogens invade and colonize plant tissue. When Mg levels are high enough during plant growth, the structural integrity of the middle lamella improves, as does the production of the energy required for defences functions and the inactivation of pathogen metabolites [24]. Magnesium ion causes P. parasitica sporangia to become nonfunctional or prevents zoospore release [19]. Treatment D1 had the highest Mg content in the soil, but it also had the highest mortality. It appears that higher soil pH levels have a greater impact on disease progression than Mg's ability to suppress disease.
Calcium stimulates photosynthesis and increases the size of sellable plant parts by increasing the absorption of some nutrients such as ammonium, potassium, and phosphorus [25]. Soil application of 1.0–2.5 Mg·ha−1 gypsum could keep soil Ca content above the standard. The higher the gypsum dose applied to the soil, the greater the Ca uptake by the leaves (Figure 5). Meanwhile, if Ca was only supplied at liming through dolomite application, it must be reapplied to meet the standard requirement of not less than 100 mg·kg−1 (Figure 3).
Calcium is used to synthesise new cell walls, specifically the middle lamella to separate newly divided cells [25]. The D-Leaf had a 'succulent-brittle' leaf base, which is commonly used as an index of growth to evaluate the plant's nutrient status [26]. This experiment demonstrated that applying calcium gypsum as a basic fertiliser to the soil has no significant effect on D-leaf length. When applied at a high-level dose of 1.5–2.5 Mg·ha−1, calcium gypsum increased plant weight significantly from 3 to 9 MAP (Table 4). Actually, after planting, the stem weight gradually increases, with no noticeable morphological changes in the plant until the reproductive development phase begins [3]. Calcium can be supplied at high concentrations of more than 10% of the dry weight in mature leaves without causing toxicity or severely impeding plant growth [27].
The treatments involving the use of gypsum in the soil may have provided more available calcium ions into the plant tissue structure [28]. The availability of calcium ions may result in a negative interaction and a decrease in the magnesium content of the leaf after planting (Figure 6). A similar finding was found in [29], where calcium fertilisation reduced magnesium assimilation in pineapple plant cell walls. Furthermore, [30] explained that over-fertilized soils with Ca can result in lower magnesium availability and uptake by the plant, which could also explain the results seen in this experiment. However, more experiments in this area should be conducted in the future. On the other hand, concerning the interaction between K and Mg, it has been established that the ratio K/Mg should not be higher than 4.0 and not present a shortage of Mg in the soil; while, for the Ca/Mg the recommendation is not more elevated than 1.25 [28,29]; in this research, the soil planting conditions exposed in Table 1 shows that the ratio K/Mg is still around 1.2, inferring a no limiting impact on Mg caused by the K level. Nevertheless, the ratio Ca/Mg is 2.2, which is higher than the suggested value. Therefore, the Ca could limit the Mg uptake by the plant even when the soil is fertilized because the Mg is relatively non-mobile in the soil. Besides, this situation could be associated with the antagonism concentration observed in the leaf.
Furthermore, applying gypsum to the soil surface as a Ca electrolyte source reduced the concentration and mass loss of dissolved reactive phosphorus and total phosphorus in runoff [28]. The gypsum salt increases the soil's ionic strength, which increases the phosphorus retention capacity of the soil particle surface, lowers the phosphorus concentration in the soil solution, and reduces leaching.
At three months after planting, gypsum application did not affect root length, root number, root fresh weight, or root dry weight (Table 5). The roots of the pineapple plant can grow continuously all year. There is evidence, however, that root growth slows after flower induction and that the maximum root mass is reached at anthesis [3]. The availability of Ca in the rhizosphere promotes root cell elongation [31]. The proliferation, however, is dependent on water and minerals in the rhizosphere. Root growth is slowed when the rhizosphere is too dry or deficient in nutrients. When the rhizosphere's condition improves, root growth increases [25]. Excessive or insufficient moisture, high salinity, and root disease are all conditions that limit Ca uptake and can result in Ca deficiency symptoms in plants [32]. The results of these experiments should be investigated further, as there has been little research on the effects of gypsum on pineapple root status and physiology behaviour.
Fruit size, crown size and fruit quality are heavily influenced by variety, environment and cultivation. Although not statistically significant, calcium gypsum affected fruit weight, crown weight, plant weight, and stem weight at harvest and increased compared to untreated regardless of treatment dose (Table 6). It has been demonstrated that high calcium content and assimilation in the plant are associated with superior potassium ion assimilation [33]. Potassium is a mineral that has been linked to increased fruit, crown, and plant structure weight at harvest in pineapple [12]. This explains why the gypsum treatments in this experiment produced higher plant, stem, fruit, and crown weight values than the untreated soil.
Sugar content determines fruit quality in most fruits [34]. Fruit quality (Physico-chemical) analyses were performed in this study with the following TSS, TA, TSS/TA (Brix acid) ratio and fruit firmness. TSS, TA and TSS/TA ratio were not significantly different between treatments, according to the results (Table 7). The total soluble solids levels in this experiment ranged from 12.0 to 13.5 Brix. These meet the requirement for fresh fruit markets in Hawaii and Australia, where the fruit must have a minimum TSS content of 12 Brix [35]. All, however, meet the United States Standards for Canned Pineapple Grades [36]. TSS activity increased in all fruits as they ripened.
When the soil contains more soluble calcium, calcium uptake into the pineapple fruit increases, as does the firmness of the flesh. In treated soil, pressing the pineapple flesh into changing shape or anthesis requires more energy (gram force) than in untreated soil, though the difference is not significant in the top, middle and bottom parts of the fruit (Table 7). Two stages of fruit ripening were observed to determine fruit firmness: SC1 (shell colour 1, 10% yellow) and SC3 (shell colour 3, 20%–30% yellow). All gypsum treatments increased the firmness of the fruits in SC1 and SC3. When more soluble calcium is available in the soil, calcium uptake into the pineapple fruit increases, as does the firmness of the flesh. It takes more energy (gram force) to press the pineapple flesh into changing shape or anthesis in treated soil than in untreated soil, though the difference is not significant in the top, middle, and bottom parts of the fruit (Table 7). To determine fruit firmness, two stages of fruit ripening were observed: SC1 (shell colour 1, 10% yellow) and SC3 (shell colour 3, 20%–30% yellow). All gypsum treatments increased the firmness of the fruits in SC1 and SC3. Previous research suggested that a high calcium level could prevent cell wall pectate deterioration. It is critical for maintaining cell membrane integrity and cell wall stabilisation [27]. Finally, the higher percentage of fruit and crown size distribution observed in gypsum treatments, particularly at doses of 0.5 and 2.5 Mg·ha−1 (Tables 8 and 9), can be attributed to the previously described calcium effect, which makes more potassium ions available to plant sink organs such as the fruit, increasing tissue structure size and weight [12,32].
Plant mortality in the dolomite treatment was 63.8% when the soil pH was 5.9, which was higher than in the gypsum treatments (3.3%–14.3%) when the soil pH was 4.5–4.7 ten weeks after planting. Soil application of 1.0–2.5 Mg·ha−1 gypsum could keep soil Ca content above the standard value of 100 mg·kg−1 for more than 7 MAP, increased Ca uptake to the leaves, but not Mg. When applied at a high dose of 1.5–2.5 Mg·ha−1, calcium gypsum increased plant weight significantly from 3 to 9 MAP. Gypsum applied to the soil before planting, followed by a fertiliser regime, met the plant's Ca and Mg requirements during an early and rapid growth stage. It is recommended to use as a substitute for dolomite when the soil pH must be maintained at a safe level to prevent heart rot disease.
The authors would like to express their gratitude to the United Graduate School of Agricultural Science (UGSAS), Gifu University, Japan for the support of this research through the Ronpaku Program. Sincere thanks are extended to the Management of PT. Great Giant Pineapple, for permitting, funding and supporting this study. Thanks are also extended to Dewi Puspita Widiarini and Ratdiana, my colleagues in R & D Dept. of GGP.
The authors declare there are no conflicts of interest.
[1] | Von Uexkull HR, Bossart RP (1989) Management of acid upland soils in Asia, In: Craswell ET, Pushparajah E (Eds.), Management of acid soils in the humid tropics of Asia, NSW: RodenPrint, 2–19. |
[2] | Prasetyo BH, Suriadikarta DA (2006) Karakteristik, potensi, dan teknologi pengelolaan tanah ultisol untuk pengembangan pertanian lahan kering di Indonesia. J Litbang Pertanian 25: 39–46. |
[3] | Malezieux E, Bartholomew DP (2003) Plant nutrition, In: Bartholomew DP, Paul RE, Rohrbach KG (Eds.), The pineapple: Botany, production and uses, Wallingford: CABI Publishing, 143–165. https://doi.org/10.1079/9780851995038.0143. |
[4] | Uchida R, Hue NV (2000) Soil acidity and liming, In: Silva JA, Uchida R (Eds.), Plant nutrient management in Hawaii's soils, approaches for tropical and subtropical agriculture, Hawaii: University of Hawaii, 101–106. |
[5] | Taiz L, Zeiger E, Moller IM, et al. (2015) Plant physiology and development, 6 Eds., Sunderland, Massachusetts: Sinaur Associates, 16–23. |
[6] | Tailep WMAK, El-Saady AM, El-Dahshouri MF, et al. (2019) Influence of foliar spray of different calcium sources on nutritional status, seed yield and quality for some peanut genotypes. Biosci Res 16: 309–319. |
[7] |
Olle M, Bender I (2009) Causes and control of calcium deficiency disorders in vegetables: A review. J Hortic Sci Bitech 84: 577–584. https://doi.org/10.1080/14620316.2009.11512568 doi: 10.1080/14620316.2009.11512568
![]() |
[8] | Von Uexkull HR (1986) Efficient fertilizer use in acid upland soils of the humid tropics, Rome: FAO, 1–59. |
[9] | Rohrbach KG, Johnson MW (2003) Pests, diseases and weeds, In: Bartholomew DP, Paul RE, Rohrbach KG (Eds.), The pineapple: Botany, production and uses, Wallingford: CABI Publishing, 203–252. |
[10] | Pegg KG (1993) Diseases, In: Broadly RH, Wassman RC, Sinclair E (Eds.), Pineapple pest and disorder, Queensland: Department of Primary Industries, 11–32. |
[11] | Mite F, Espinosa J, Medina L (2010) Liming effect on pineapple yield and soil properties in volcanic soils. Better Crops 94: 7–9. |
[12] | Vasquez-Jimenez J, Bartholomew DP (2018) Plant nutrition, In: Sanewski GM, Bartholomew DP, Paull RE (Eds.), The pineapple: Botany, production and uses, 2 Eds., Wallingford: CABI Publishing, 175–202. |
[13] |
Silva JA, Hamasaki R, Ogoshi R, et al. (2006) Lime, gypsum, and basaltic dust effects on the calcium nutrition and fruit quality of pineapple. Acta Hortic 702: 123–131. https://doi.org/10.17660/ActaHortic.2006.702.15 doi: 10.17660/ActaHortic.2006.702.15
![]() |
[14] | Pegg K, Giblin F (2008) Principle of Phytophthora root rot management in establish orchards Queensland. Talking Avocados 19: 36–38. |
[15] |
Messenger BJ, Menge JA, Pond E (2000) Effect of gypsum on zoospores and sporangia of Phytophthora cinnamomi in field soil. Plant Dis 84: 617–621. https://doi.org/10.1094/PDIS.2000.84.6.617 doi: 10.1094/PDIS.2000.84.6.617
![]() |
[16] | Correia AF, Neves LG, Serafim ME, et al. (2017) Chemical attributes of soil with use of a cover crop and agricultural gypsum in pineapple. Rev Cienc Agrar 60: 25–32. |
[17] |
Afandi A, Subandiyah S, Wibowo A, et al. (2021) Population genetic analysis of Phytophthora nicotianae associated with heart rot in pineapple revealed gen flow between population. Biodiversitas 22: 3342–3348. https://doi.org/10.13057/biodiv/d220830 doi: 10.13057/biodiv/d220830
![]() |
[18] |
Loekito S, Afandi, Afandi A, et al. (2022) Study on soil properties and species conformity of Phytophthora species in a pineapple field. Int J Agric Biol 27: 361–370. https://doi.org/10.17957/IJAB/15.1936 doi: 10.17957/IJAB/15.1936
![]() |
[19] | Tsao PH, Draft GC, Sztejnberg A, et al., Potting Mixes for Control Phytophthora Root Rot, 1986. Available from: https://slosson.ucdavis.edu/newsletters/Tsao_198629144.pdf. |
[20] |
Zook MN, Rush JS, Kuc JC (1987) A role for Ca2+ in the elicitation of rishitin and lubimin accumulation in potato tuber tissue. Plant Physiol 84: 520–525. https://doi.org/10.1104/pp.84.2.520 doi: 10.1104/pp.84.2.520
![]() |
[21] | Liming C, Warren AD, Gypsum as an Agricultural Amendment, General Use Guidelines, 2011. Available from: https://www.academia.edu/35029694/Bulletin_945. |
[22] | Kelly DS (1993) Nutritional disorder, In: Roger HB, Rudolf CW, Eric S (Eds.), Pineapple pest and disorders, Queensland: Department of Primary Industries, 33–42. |
[23] |
Nome C, Magalhaes PC, Oliveira E, et al. (2009) Differences in intracellular localization of corn stunt spiroplasmas in magnesium treated maize. Biocell 33: 133–136. https://doi.org/10.32604/biocell.2009.33.133 doi: 10.32604/biocell.2009.33.133
![]() |
[24] |
Huber DM, Jones J (2013) The role of magnesium in plant disease. Plant Soil 368: 73–85. https://doi.org/10.1007/s11104-012-1476-0 doi: 10.1007/s11104-012-1476-0
![]() |
[25] | Taiz L, Zeiger E (2002) Plant physiology, 3 Eds. Sunderland, Massachusetts: Sinaur Associates. |
[26] | Souza LFS, Reinhardt DH (2007) Pineapple, In: Johnson AE (Ed.), Tropical fruits of Brazil, Horgen, Switzerland: International Potash Institute, 179–201. |
[27] | Marschner H (2012) Mineral nutrition and yield response, In: Marschner's mineral nutrition of higher plants, 2 Eds, London, San Diego: Academic Press, 184–200. https://doi.org/10.1016/B978-0-08-057187-4.50012-6 |
[28] |
Favaretto N, Norton LD, Joern BC, et al. (2006) Gypsum amendment and exchangeable calcium and magnesium affecting phosphorus and nitrogen in runoff. Soil Sci Soc Am J 70: 1788–1796. https://doi.org/10.2136/sssaj2005.0228 doi: 10.2136/sssaj2005.0228
![]() |
[29] |
Cano-Reinoso DM, Soesanto L, Kharisun, et al. (2021) Effect of pre-harvest fruit covers and calcium fertilization on pineapple thermotolerance and flesh translucency. Emir J Food Agr 33: 834–845. https://doi.org/10.9755/ejfa.2021.v33.i10.2766 doi: 10.9755/ejfa.2021.v33.i10.2766
![]() |
[30] | JB Jones Jr (2012) Soil fertility and principals, In: Plant nutrition and soil fertility manual, 2 Eds., Boca Raton: CRC press, 5–11. https://doi.org/10.1201/b11577-5 |
[31] | De Freitas ST, Mitcham EJ (2012) Factors involved in fruit calcium deficiency disorders. Hortic Rev 40: 107–146. |
[32] |
Saure M (2014) Why calcium deficiency is not the cause of blossom-end rot in tomato and pepper fruit—a reappraisal. Sci Hortic 174: 151–154. https://doi.org/10.1016/j.scienta.2014.05.020 doi: 10.1016/j.scienta.2014.05.020
![]() |
[33] |
Gerendás J, Führs H (2013) The significance of magnesium for crop quality. Plant Soil 368: 101–128. https://doi.org/10.1007/s11104-012-1555-2 doi: 10.1007/s11104-012-1555-2
![]() |
[34] |
Villanueva MJ, Tenorio MD, Esteban MA, et al. (2004) Compositional changes during ripening of two cultivars of maskmelon fruits. Food Chem 87: 179–183. https:/doi.org/10.1016/j.foodchem.2003.11.009 doi: 10.1016/j.foodchem.2003.11.009
![]() |
[35] | Lobo MG, Yahia E (2017) Biology and postharvest physiology of pineapple, In: Lobo MG, Paull RE (Eds.), Handbook of pineapple technology: Production, postharvest science, processing and nutrition. Chichester, Hoboken: John Wiley & Sons, 39–61. https://doi.org/10.1002/9781118967355.ch3 |
[36] | United States Department of Agriculture, United States Standards for Grades of Canned Pineapple, 1990. Available from: https://ams.usda.gov/sites/default/files/media/canned_pineapple_standard.pdf. |
Properties | Unit | Experiment Ⅰ | Experiment Ⅱ | Fine compost | |
At planting (Dec. 2016) | Initial soil (May. 2017) | At planting (Sep. 2017) | |||
pH | – | 4.6 | 4.2 | 4.4 | 7.2 |
P | mg·kg−1 | 17.7 | 21.2 | 22.9 | 2.6 |
K | mg·kg−1 | 86.4 | 68.5 | 75 | 18.13 |
Ca | mg·kg−1 | 314.4 | 60.3 | 127.8 | 21.5 |
Mg | mg·kg−1 | 98.6 | 49.1 | 60.8 | 5.26 |
Cu | mg·kg−1 | Not analysed | 0.6 | 0.6 | Not analysed |
Soil Organic C | Wt% | 1.2 | 1.4 | 1.3 | 21.6 |
Total N | Wt% | Not analysed | Not analysed | Not analysed | 1.68 |
C/N ratio | – | Not analysed | Not analysed | Not analysed | 12.86 |
Note: Liming dolomite 1 Mg·ha−1 was done in June 2017 (Exp. 2). |
Treatment | Plant mortality (%) | |||
4 WAP | 6 WAP | 8 WAP | 10 WAP | |
G0 (Untreated) | 0.0 a | 1.3 a | 2.7 a | 3.3 a |
G1 (Gypsum 1.0 Mg·ha−1) | 0.0 a | 4.7 a | 9.3 a | 10.3 a |
G2 (Gypsum 1.5 Mg·ha−1) | 0.0 a | 4.3 a | 7.0 a | 8.7 a |
G3 (Gypsum 2.0 Mg·ha−1) | 0.3 a | 7.3 ab | 12.0 a | 14.3 a |
D1 (Dolomite 2.0 Mg·ha−1) | 0.7 a | 24.3 b | 54.3 b | 63.8 b |
P-value | 0.12 | 0.01 | 0 | 0 |
Note: Means within a column followed by a common letter are not significantly different (P < 0.05) according to the Tuckey test of difference. |
Treatment | Soil pH | Ca (mg·kg−1) | Mg (mg·kg−1) | ||||||
0 WAP | 4 WAP | 10 WAP | 0 WAP | 4 WAP | 10 WAP | 0 WAP | 4 WAP | 10 WAP | |
G0 | 4.6 | 5.1 a | 4.5 a | 314 | 430 a | 311 a | 99 | 137 ab | 75 ab |
G1 | 4.6 | 5.3 ab | 4.6 a | 314 | 493 a | 379 ab | 99 | 156 ab | 77 ac |
G2 | 4.6 | 5.3 ab | 4.7 a | 314 | 489 a | 400 b | 99 | 146 a | 98 ac |
G3 | 4.6 | 5.0 a | 4.7 a | 314 | 448 a | 389 b | 99 | 120 b | 62 b |
D1 | 4.6 | 5.5 b | 5.9 b | 314 | 467 a | 369 ab | 99 | 162 c | 159 c |
P-value | – | 0 | 0 | – | 0.61 | 0.02 | – | 0 | 0 |
Note: Means within a column followed by a common letter are not significantly different (P < 0.05) according to the Tuckey test of difference. |
Treatment | D-Leaf length (cm) | Plant weight (g) | ||||||
3 MAP | 7 MAP | 9 MAP | 11 MAP | 3 MAP | 7 MAP | 9 MAP | 11 MAP | |
G0 (Untreated) | 51.2 a | 75.9 a | 87.2 a | 95.1 a | 201.3 a | 842.5 a | 1336.3 a | 2546.3 a |
G1 (0.5 Mg·ha−1) | 51.0 a | 75.8 a | 88.1 a | 97.4 a | 215.0 ab | 1032.5 a | 1606.3 ab | 2592.5 a |
G2 (1.0 Mg·ha−1) | 53.1 a | 77.4 a | 89.4 a | 96.5 a | 222.5 ab | 901.3 ab | 1450.0 ab | 2772.5 a |
G3 (1.5 Mg·ha−1) | 51.9 a | 76.4 a | 89.0 a | 97.2 a | 207.5 ab | 1021.3 b | 1736.3 b | 2598.8 a |
G4 (2.0 Mg·ha−1) | 53.6 a | 78.1 a | 90.2 a | 104.7 a | 210.0 ab | 1002.5 ab | 1698.8 ab | 2722.5 a |
G5 (2.5 Mg·ha−1) | 54.1 a | 76.6 a | 88.7 a | 96.2 a | 225.0 b | 1037.5 b | 1725.0 ab | 2520.0 a |
P-value | 0.56 | 0.94 | 0.91 | 0.36 | 0.02 | 0.01 | 0.02 | 0.66 |
Note: Means within a column followed by a common letter are not significantly different (P < 0.05) according to Tuckey test of difference. |
Treatment | Root length (cm) | Total root number | Root fresh weight (cm) | Root dry weight (cm) |
G0 (Untreated) | 43.1 a | 38.9 a | 5.3 a | 2.5 a |
G1 (0.5 Mg·ha−1) | 41.8 a | 29.9 a | 5.8 a | 2.7 a |
G2 (1.0 Mg·ha−1) | 45.1 a | 35.8 a | 5.3 a | 2.6 a |
G3 (1.5 Mg·ha−1) | 41.9 a | 32.5 a | 5.3 a | 2.4 a |
G4 (2.0 Mg·ha−1) | 46.3 a | 34.7 a | 4.5 a | 2.1 a |
G5 (2.5 Mg·ha−1) | 43.9 a | 36.3 a | 6.5 a | 3.3 a |
P-value | 0.82 | 0.16 | 0.25 | 0.1 |
Note: Means within a column followed by a common letter are not significantly different (P < 0.05) according to the Tuckey test of difference. |
Treatment | Plant weight at harvest (g) | Stem weight at harvest (g) | Fruit weight (g) | Crown weight (g) |
G0 (Untreated) | 2451.3 a | 520.0 a | 1455.0 a | 322.5 a |
G1 (0.5 Mg·ha−1) | 2908.3 a | 607.5 a | 1497.5 a | 402.5 a |
G2 (1.0 Mg·ha−1) | 2730.0 a | 590.0 a | 1731.3 a | 365.0 a |
G3 (1.5 Mg·ha−1) | 2645.0 a | 580.0 a | 1467.5 a | 330.0 a |
G4 (2.0 Mg·ha−1) | 2647.5 a | 532.5 a | 1677.5 a | 335.0 a |
G5 (2.5 Mg·ha−1) | 2527.3 a | 537.5 a | 1632.5 a | 345.0 a |
P-value | 0.41 | 0.59 | 0.13 | 0.51 |
Note: Means within a column followed by a common letter are not significantly different (P < 0.05) according to the Tuckey test of difference. |
Treatment | Fruit TSS (oBrix) | Fruit TA (%) | TSS/TA ratio | Fruit texture (firmness) | |||||
Shell colour 1 (10% yellow) | Shell colour 3 (20%–30% yellow) | ||||||||
Top (g) | Middle (g) | Bottom (g) | Top (g) | Middle (g) | Bottom (g) | ||||
G0 (Untreated) | 13.5 a | 0.55 a | 24.5 | 282.8 a | 282.2 a | 278.3 a | 230.6 a | 261.7 a | 230.6 a |
G1 (0.5 Mg·ha−1) | 12.6 a | 0.49 a | 25.6 | 296.7 a | 298.3 a | 282.2 a | 267.2 a | 267.2 a | 267.2 a |
G2 (1.0 Mg·ha−1) | 12.1 ab | 0.46 a | 26.2 | 283.3 a | 289.4 a | 281.7 a | 257.8 a | 277.2 a | 257.8 a |
G3 (1.5 Mg·ha−1) | 12.7 a | 0.53 a | 23.9 | 326.1 a | 312.8 a | 282.2 a | 262.8 a | 279.4 a | 262.8 a |
G4 (2.0 Mg·ha−1) | 12.0 ab | 0.50 a | 23.9 | 281.1 a | 289.4 a | 299.4 a | 254.4 a | 267.8 a | 254.4 a |
G5 (2.5 Mg·ha−1) | 13.3 a | 0.52 a | 25.6 | 285.6 a | 292.2 a | 303.3 a | 293.9 a | 275.6 a | 293.9 a |
P-value | 0.045 | 0.14 | – | 0.916 | 0.72 | 0.844 | 0.431 | 0.977 | 0.121 |
Note: Means within a column followed by a common letter are not significantly different (P < 0.05) according to the Tuckey test of difference. |
Treatment | Fruit size distribution (%) | |||||
< 1T | 1 T | 1 3/8 T | 2 T | 2 1/2 T | (2 T + 2 1/2 T) | |
G0 (Untreated) | 3.7 a | 12.0 a | 15.4 a | 26.0 a | 43.0 a | 68.9 |
G1 (0.5 Mg·ha−1) | 3.0 a | 8.2 a | 10.7 a | 31.2 a | 46.8 a | 78 |
G2 (1.0 Mg·ha−1) | 2.8 a | 8.0 a | 15.6 a | 33.1 a | 40.5 a | 73.6 |
G3 (1.5 Mg·ha−1) | 2.2 a | 8.2 a | 15.1 a | 28.5 a | 46.1 a | 74.5 |
G4 (2.0 Mg·ha−1) | 4.5 a | 8.4 a | 13.1 a | 30.8 a | 43.1 a | 73.9 |
G5 (2.5 Mg·ha−1) | 1.7 a | 11.4 a | 14.8 a | 30.7 a | 41.4 a | 72.1 |
P-value | 0.601 | 0.771 | 0.707 | 0.863 | 0.588 | – |
Note: Means within a column followed by a common letter are not significantly different (P < 0.05) according to the Tuckey test of difference. |
Treatment | Crown size distribution (%) | |||||
Extra small | Small | Medium | Big | Extra big | (Big + Extra big) | |
G0 (Untreated) | 0.3 a | 3.2 a | 60.1 a | 33.2 a | 3.2 a | 36.4 |
G1 (0.5 Mg·ha−1) | 0.0 a | 0.0 a | 40.1 a | 54.2 a | 5.7 a | 59.9 |
G2 (1.0 Mg·ha−1) | 0.0 a | 3.0 a | 39.3 a | 54.0 a | 3.7 a | 57.7 |
G3 (1.5 Mg·ha−1) | 0.0 a | 1.7 a | 43.9 a | 52.6 a | 1.8 a | 54.4 |
G4 (2.0 Mg·ha−1) | 0.0 a | 3.3 a | 40.8 a | 54.4 a | 1.5 a | 56.9 |
G5 (2.5 Mg·ha−1) | 0.0 a | 3.7 a | 35.0 a | 56.0 a | 5.7 a | 61.7 |
P-value | 0.458 | 0.895 | 0.433 | 0.346 | 0.691 | – |
Note: Means within a column followed by a common letter are not significantly different (P < 0.05) according to the Tuckey test of difference. |
Properties | Unit | Experiment Ⅰ | Experiment Ⅱ | Fine compost | |
At planting (Dec. 2016) | Initial soil (May. 2017) | At planting (Sep. 2017) | |||
pH | – | 4.6 | 4.2 | 4.4 | 7.2 |
P | mg·kg−1 | 17.7 | 21.2 | 22.9 | 2.6 |
K | mg·kg−1 | 86.4 | 68.5 | 75 | 18.13 |
Ca | mg·kg−1 | 314.4 | 60.3 | 127.8 | 21.5 |
Mg | mg·kg−1 | 98.6 | 49.1 | 60.8 | 5.26 |
Cu | mg·kg−1 | Not analysed | 0.6 | 0.6 | Not analysed |
Soil Organic C | Wt% | 1.2 | 1.4 | 1.3 | 21.6 |
Total N | Wt% | Not analysed | Not analysed | Not analysed | 1.68 |
C/N ratio | – | Not analysed | Not analysed | Not analysed | 12.86 |
Note: Liming dolomite 1 Mg·ha−1 was done in June 2017 (Exp. 2). |
Treatment | Plant mortality (%) | |||
4 WAP | 6 WAP | 8 WAP | 10 WAP | |
G0 (Untreated) | 0.0 a | 1.3 a | 2.7 a | 3.3 a |
G1 (Gypsum 1.0 Mg·ha−1) | 0.0 a | 4.7 a | 9.3 a | 10.3 a |
G2 (Gypsum 1.5 Mg·ha−1) | 0.0 a | 4.3 a | 7.0 a | 8.7 a |
G3 (Gypsum 2.0 Mg·ha−1) | 0.3 a | 7.3 ab | 12.0 a | 14.3 a |
D1 (Dolomite 2.0 Mg·ha−1) | 0.7 a | 24.3 b | 54.3 b | 63.8 b |
P-value | 0.12 | 0.01 | 0 | 0 |
Note: Means within a column followed by a common letter are not significantly different (P < 0.05) according to the Tuckey test of difference. |
Treatment | Soil pH | Ca (mg·kg−1) | Mg (mg·kg−1) | ||||||
0 WAP | 4 WAP | 10 WAP | 0 WAP | 4 WAP | 10 WAP | 0 WAP | 4 WAP | 10 WAP | |
G0 | 4.6 | 5.1 a | 4.5 a | 314 | 430 a | 311 a | 99 | 137 ab | 75 ab |
G1 | 4.6 | 5.3 ab | 4.6 a | 314 | 493 a | 379 ab | 99 | 156 ab | 77 ac |
G2 | 4.6 | 5.3 ab | 4.7 a | 314 | 489 a | 400 b | 99 | 146 a | 98 ac |
G3 | 4.6 | 5.0 a | 4.7 a | 314 | 448 a | 389 b | 99 | 120 b | 62 b |
D1 | 4.6 | 5.5 b | 5.9 b | 314 | 467 a | 369 ab | 99 | 162 c | 159 c |
P-value | – | 0 | 0 | – | 0.61 | 0.02 | – | 0 | 0 |
Note: Means within a column followed by a common letter are not significantly different (P < 0.05) according to the Tuckey test of difference. |
Treatment | D-Leaf length (cm) | Plant weight (g) | ||||||
3 MAP | 7 MAP | 9 MAP | 11 MAP | 3 MAP | 7 MAP | 9 MAP | 11 MAP | |
G0 (Untreated) | 51.2 a | 75.9 a | 87.2 a | 95.1 a | 201.3 a | 842.5 a | 1336.3 a | 2546.3 a |
G1 (0.5 Mg·ha−1) | 51.0 a | 75.8 a | 88.1 a | 97.4 a | 215.0 ab | 1032.5 a | 1606.3 ab | 2592.5 a |
G2 (1.0 Mg·ha−1) | 53.1 a | 77.4 a | 89.4 a | 96.5 a | 222.5 ab | 901.3 ab | 1450.0 ab | 2772.5 a |
G3 (1.5 Mg·ha−1) | 51.9 a | 76.4 a | 89.0 a | 97.2 a | 207.5 ab | 1021.3 b | 1736.3 b | 2598.8 a |
G4 (2.0 Mg·ha−1) | 53.6 a | 78.1 a | 90.2 a | 104.7 a | 210.0 ab | 1002.5 ab | 1698.8 ab | 2722.5 a |
G5 (2.5 Mg·ha−1) | 54.1 a | 76.6 a | 88.7 a | 96.2 a | 225.0 b | 1037.5 b | 1725.0 ab | 2520.0 a |
P-value | 0.56 | 0.94 | 0.91 | 0.36 | 0.02 | 0.01 | 0.02 | 0.66 |
Note: Means within a column followed by a common letter are not significantly different (P < 0.05) according to Tuckey test of difference. |
Treatment | Root length (cm) | Total root number | Root fresh weight (cm) | Root dry weight (cm) |
G0 (Untreated) | 43.1 a | 38.9 a | 5.3 a | 2.5 a |
G1 (0.5 Mg·ha−1) | 41.8 a | 29.9 a | 5.8 a | 2.7 a |
G2 (1.0 Mg·ha−1) | 45.1 a | 35.8 a | 5.3 a | 2.6 a |
G3 (1.5 Mg·ha−1) | 41.9 a | 32.5 a | 5.3 a | 2.4 a |
G4 (2.0 Mg·ha−1) | 46.3 a | 34.7 a | 4.5 a | 2.1 a |
G5 (2.5 Mg·ha−1) | 43.9 a | 36.3 a | 6.5 a | 3.3 a |
P-value | 0.82 | 0.16 | 0.25 | 0.1 |
Note: Means within a column followed by a common letter are not significantly different (P < 0.05) according to the Tuckey test of difference. |
Treatment | Plant weight at harvest (g) | Stem weight at harvest (g) | Fruit weight (g) | Crown weight (g) |
G0 (Untreated) | 2451.3 a | 520.0 a | 1455.0 a | 322.5 a |
G1 (0.5 Mg·ha−1) | 2908.3 a | 607.5 a | 1497.5 a | 402.5 a |
G2 (1.0 Mg·ha−1) | 2730.0 a | 590.0 a | 1731.3 a | 365.0 a |
G3 (1.5 Mg·ha−1) | 2645.0 a | 580.0 a | 1467.5 a | 330.0 a |
G4 (2.0 Mg·ha−1) | 2647.5 a | 532.5 a | 1677.5 a | 335.0 a |
G5 (2.5 Mg·ha−1) | 2527.3 a | 537.5 a | 1632.5 a | 345.0 a |
P-value | 0.41 | 0.59 | 0.13 | 0.51 |
Note: Means within a column followed by a common letter are not significantly different (P < 0.05) according to the Tuckey test of difference. |
Treatment | Fruit TSS (oBrix) | Fruit TA (%) | TSS/TA ratio | Fruit texture (firmness) | |||||
Shell colour 1 (10% yellow) | Shell colour 3 (20%–30% yellow) | ||||||||
Top (g) | Middle (g) | Bottom (g) | Top (g) | Middle (g) | Bottom (g) | ||||
G0 (Untreated) | 13.5 a | 0.55 a | 24.5 | 282.8 a | 282.2 a | 278.3 a | 230.6 a | 261.7 a | 230.6 a |
G1 (0.5 Mg·ha−1) | 12.6 a | 0.49 a | 25.6 | 296.7 a | 298.3 a | 282.2 a | 267.2 a | 267.2 a | 267.2 a |
G2 (1.0 Mg·ha−1) | 12.1 ab | 0.46 a | 26.2 | 283.3 a | 289.4 a | 281.7 a | 257.8 a | 277.2 a | 257.8 a |
G3 (1.5 Mg·ha−1) | 12.7 a | 0.53 a | 23.9 | 326.1 a | 312.8 a | 282.2 a | 262.8 a | 279.4 a | 262.8 a |
G4 (2.0 Mg·ha−1) | 12.0 ab | 0.50 a | 23.9 | 281.1 a | 289.4 a | 299.4 a | 254.4 a | 267.8 a | 254.4 a |
G5 (2.5 Mg·ha−1) | 13.3 a | 0.52 a | 25.6 | 285.6 a | 292.2 a | 303.3 a | 293.9 a | 275.6 a | 293.9 a |
P-value | 0.045 | 0.14 | – | 0.916 | 0.72 | 0.844 | 0.431 | 0.977 | 0.121 |
Note: Means within a column followed by a common letter are not significantly different (P < 0.05) according to the Tuckey test of difference. |
Treatment | Fruit size distribution (%) | |||||
< 1T | 1 T | 1 3/8 T | 2 T | 2 1/2 T | (2 T + 2 1/2 T) | |
G0 (Untreated) | 3.7 a | 12.0 a | 15.4 a | 26.0 a | 43.0 a | 68.9 |
G1 (0.5 Mg·ha−1) | 3.0 a | 8.2 a | 10.7 a | 31.2 a | 46.8 a | 78 |
G2 (1.0 Mg·ha−1) | 2.8 a | 8.0 a | 15.6 a | 33.1 a | 40.5 a | 73.6 |
G3 (1.5 Mg·ha−1) | 2.2 a | 8.2 a | 15.1 a | 28.5 a | 46.1 a | 74.5 |
G4 (2.0 Mg·ha−1) | 4.5 a | 8.4 a | 13.1 a | 30.8 a | 43.1 a | 73.9 |
G5 (2.5 Mg·ha−1) | 1.7 a | 11.4 a | 14.8 a | 30.7 a | 41.4 a | 72.1 |
P-value | 0.601 | 0.771 | 0.707 | 0.863 | 0.588 | – |
Note: Means within a column followed by a common letter are not significantly different (P < 0.05) according to the Tuckey test of difference. |
Treatment | Crown size distribution (%) | |||||
Extra small | Small | Medium | Big | Extra big | (Big + Extra big) | |
G0 (Untreated) | 0.3 a | 3.2 a | 60.1 a | 33.2 a | 3.2 a | 36.4 |
G1 (0.5 Mg·ha−1) | 0.0 a | 0.0 a | 40.1 a | 54.2 a | 5.7 a | 59.9 |
G2 (1.0 Mg·ha−1) | 0.0 a | 3.0 a | 39.3 a | 54.0 a | 3.7 a | 57.7 |
G3 (1.5 Mg·ha−1) | 0.0 a | 1.7 a | 43.9 a | 52.6 a | 1.8 a | 54.4 |
G4 (2.0 Mg·ha−1) | 0.0 a | 3.3 a | 40.8 a | 54.4 a | 1.5 a | 56.9 |
G5 (2.5 Mg·ha−1) | 0.0 a | 3.7 a | 35.0 a | 56.0 a | 5.7 a | 61.7 |
P-value | 0.458 | 0.895 | 0.433 | 0.346 | 0.691 | – |
Note: Means within a column followed by a common letter are not significantly different (P < 0.05) according to the Tuckey test of difference. |