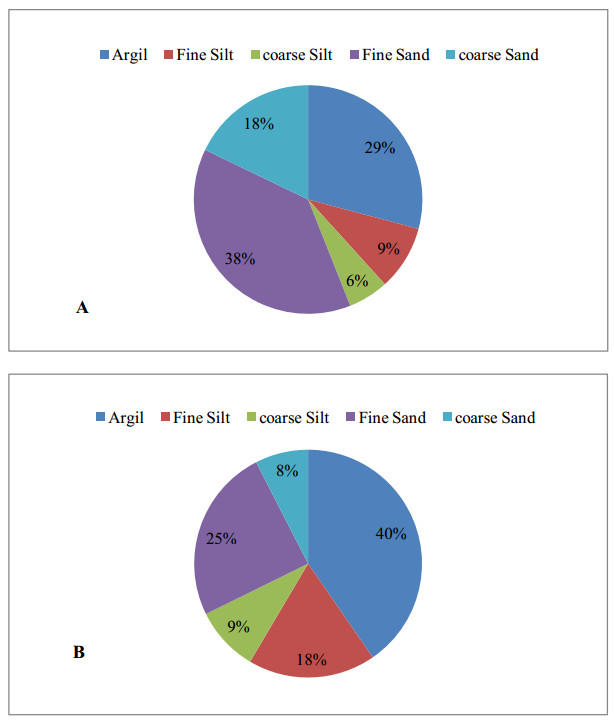
Citation: Soukaina Ouansafi, Fahde Abdelilah, Mostafa Kabine, Hind Maaghloud, Fatima Bellali, Karima El Bouqdaoui. The effects of soil proprieties on the yield and the growth of tomato plants and fruits irrigated by treated wastewater[J]. AIMS Agriculture and Food, 2019, 4(4): 921-938. doi: 10.3934/agrfood.2019.4.921
[1] | Nono Carsono, Fadlilah Aida Rahmani, Rangga Jiwa Wibawa, Santika Sari, Anas, Ryo Ohsawa, Ayako Shimono, Hiroshi Ezura . Invasiveness, allelopathic potential and unintended effects of miraculin transgenic tomato to soil microbes. AIMS Agriculture and Food, 2022, 7(4): 872-882. doi: 10.3934/agrfood.2022053 |
[2] | Nono Carsono, Faza A. Maulana, Iqbal F. Elfakhriano, Ade Ismail, Noladhi Wicaksana, Santika Sari, Hiroshi Ezura . The comparative analysis of agronomic, compositional, and physiological traits of miraculin transgenic tomato in the confined field trial. AIMS Agriculture and Food, 2023, 8(1): 187-197. doi: 10.3934/agrfood.2023010 |
[3] | Saowanee Wijitkosum, Preamsuda Jiwnok . Effect of biochar on Chinese kale and carbon storage in an agricultural area on a high rise building. AIMS Agriculture and Food, 2019, 4(1): 177-193. doi: 10.3934/agrfood.2019.1.177 |
[4] | Md. Shaha Nur Kabir, Kamal Rasool, Wang-Hee Lee, Seong-In Cho, Sun-Ok Chung . Influence of delayed cooling on the quality of tomatoes (Solanum lycopersicum L.) stored in a controlled chamber. AIMS Agriculture and Food, 2020, 5(2): 272-285. doi: 10.3934/agrfood.2020.2.272 |
[5] | Ogunniyi Adebayo, Omonona Bolarin, Abioye Oyewale, Olagunju Kehinde . Impact of irrigation technology use on crop yield, crop income and household food security in Nigeria: A treatment effect approach. AIMS Agriculture and Food, 2018, 3(2): 154-171. doi: 10.3934/agrfood.2018.2.154 |
[6] | W. Mupangwa, I. Nyagumbo, E. Mutsamba . Effect of different mulching materials on maize growth and yield in conservation agriculture systems of sub-humid Zimbabwe. AIMS Agriculture and Food, 2016, 1(2): 239-253. doi: 10.3934/agrfood.2016.2.239 |
[7] | Diana N. Sholehah, Sucipto Hariyanto, Hery Purnobasuki . Effect of salinity on growth, physiology, and production of groundcherry (Physalis angulata L.). AIMS Agriculture and Food, 2022, 7(4): 750-761. doi: 10.3934/agrfood.2022046 |
[8] | A. Nurbekov, A. Akramkhanov, A. Kassam, D. Sydyk, Z. Ziyadaullaev, J.P.A. Lamers . Conservation Agriculture for combating land degradation in Central Asia: a synthesis. AIMS Agriculture and Food, 2016, 1(2): 144-156. doi: 10.3934/agrfood.2016.2.144 |
[9] | Mohammad Zahirul Islam, Young-Tack Lee, Mahmuda Akter Mele, In-Lee Choi, Ho-Min Kang . Effect of fruit size on fruit quality, shelf life and microbial activity in cherry tomatoes. AIMS Agriculture and Food, 2019, 4(2): 340-348. doi: 10.3934/agrfood.2019.2.340 |
[10] | Supriyono Loekito, Afandi, Auliana Afandi, Naomasa Nishimura, Hiroyuki Koyama, Masateru Senge . Gypsum supplies calcium to Ultisol soil and its effect on Phytophthora nicotianae, pineapple (Ananas comosus) growth, yield and fruit quality in lower single row bed under climate change issue. AIMS Agriculture and Food, 2022, 7(3): 721-736. doi: 10.3934/agrfood.2022044 |
In Morocco, the increasing population growth and the establishment of anthropogenic activities in urban and rural areas have caused huge quantities of wastewater discharges to be generated. These have been released into watercourses without prior treatment and used for the irrigation of agricultural fields, estimated at an area of 7,200 hectares [1] thus causing major imbalances in the different components of agro ecosystems.
In this context, various studies have been carried out in several aquatic environments such as Oum ErRbia, Oued Sebbou and Oued Bouregreg, which have revealed disturbing pollution levels [2,3,4]. In addition, water from Oued Hassar, used mainly in irrigating crops in the Mediouna zone, was the subject of a physico-chemical and metallic study demonstrating the effect of direct discharges of wastewater from the Mediouna locality. This study revealed important chemical contaminations, a sign of the degradation of the quality of this irrigation water [5]. In fact, Lydec installed a waste water treatment plant (WWTP) in the suburban region of Greater Casablanca (Mediouna Province) 2013 in order to reduce the harmful impact of these discharges in the water of Oued Hassar [6].
As a result, alternative supply is conceivable in order to combat any shortage of this reserve, and economically the use of treated waste water for the means of irrigation would make it possible to provide significant quantities of water at a lower cost. In addition, in terms of environment this water would present a significant source of nutrition for the growth of the plants because of its richness in trace elements [7]. The tomato is among the most demanding crops in terms of water and also one of the most predominant of Moroccan agriculture. This vegetable occupies a key place with an annual world production of more than 130 million tons (2015–2016). At the national level, 1.2 million tons per hectare of this vegetable crop are produced, of which 950,000 tons are primary tomatoes, 240,000 tons are seasonal tomatoes and 40,000 tons are industrial tomatoes [8].
Therefore, in collaboration with Lydec and within the framework of rational management of the water and edaphic resources, our objective was to evaluate the effect of these abiotic parameters on the agricultural yield in a qualitative and quantitative way in order to answer the needs of Mediouna's population. At the same time, our endeavor was to ensure sustainable development by adopting a strategy which is economically profitable and above all environmentally protective.
The main objective of the present study is to investigate the effects of two different soils: FS and MS in Casablanca region, Morocco, on the yield and the growth of tomato plants and fruits irrigated by a surface drip system with treated wastewater.
In this study, we chose two different types of soils to use for comparison: the first one studied belongs to the Mediouna WWTP(MS), known for its agricultural activities, while the other study was conducted at the Ain Chock Faculty of Science (FS) of the Hassan 2 University in Casablanca. Both soils were analyzed in order to evaluate their degree of fertility as well as their granulometric compositions. In each plot, eight samples were taken at a depth of 30 cm and then dried [9,10], crushed with mortar, then sieved to 2 mm and finally subjected to chemical analyzes.
The irrigation water used in our tests is treated water from the WWTP in the province of Mediouna taken at the end of the treatment process. This station receives waste water of various types: domestic, industrial and rainwater, which are collected in a separate sewage sanitary network; i.e., the industrial wastewater is discharged into a collector located downstream from the industrial area of Mediouna and joins the domestic wastewater system afterwards. The quantity of water treated daily is equivalent to 3800 m3, according to an intensive treatment system of the RBM (Membrane Biological Reactor) type, which is a biological treatment coupled with external membrane filtration. The TWW which has gone through this treatment process is discharged into the Oued Hassar stream, used by the population of Mediouna Province for agricultural purposes [6].
The qualitative study of the treated wastewater concerned the physicochemical analyzes and the metal contents according to the standards required for the water intended for irrigation, drinking water, and the environment [11].
The treated wastewater irrigation was analyzed in triplicate, which refer to the common methods [12]. The analysis included the physicochemical parameters of pH, electrical conductivity (ECw; µs cm −1), total sus- solids (TSS; mg L−1), biological oxygen demand over 5 days (BOD5; mg L−1), chemical oxygen demand (COD; mg L−1), nitrate-nitrogen (NO3-N; mg L−1), nitrite-nitrogen (NO2–N; mg L−1), total phosphorus (Total P; mg L−1), orthophosphorus (PO4-P; mg L−1), nitrogen Kjeldahl total (NTK; mg L−1), sulphate (SO4-; mg L−1).
The soil electrical conductivity (mmhos cm−1), pH (H2O) and pH (KCl) were measured on aqueous soil extracts (ASE) using an electrical conductivity meter and pH meter. The organic Matter (OM, %) was determined by dichromate oxidation method [13]. The ammonium-Nitrogen (NH4-N, mg kg−1) and nitrate nitrogen (NO3-N, mg kg−1) were determined according to Bremner and Keeney [14].The chloride ion (Cl−, mg kg−1) and sodium ion (Na+, mg kg−1) in ASE were determined by titration[15]. The phosphorus pentoxide (P2O5, mg kg−1) was estimated by Olsen's method [16]. The potassium oxide (K2O, mg kg−1), magnesium oxide (MgO, mg kg−1) and calcium oxide (CaO, mg kg−1) were analyzed by fluorescence through X rays [17]. The total carbonate (Total CaCO3, %) was determined according to the method described by Black et al. [18], while active carbonate (Active CaCO3, %) was determined by the method of Loeppert and Suarez [19].
Pb, Zn, Cu, Co, Cr, Cd, Fe, Hg, As, Al, Cr 6, Sn, Ar, Ba, Mn and Ni contents in the soil samples and the water. Metal concentrations were determined by atomic absorption spectrophotometry (Perkin-Elmer, AAAnalyst800). The limits standard of detection the different heavy metals are presented in Tables 2 and 3.
The species that was the subject of this study is the tomato (Solanum lycopersicum) belonging to the Solanaceae family, very often consumed by Moroccan households in the form of raw or cooked fleshy fruit. This species was chosen firstly due to its culinary and nutritional importance given its high phosphorus content, vitamins A and C [20] and secondly due to its high water requirements estimated at 6000 m3 per hectare with the drip irrigation system.
Tomato seeds were placed in alveolate trays containing peat (18/03/2015) and irrigated daily with drinking water. As soon as seedlings at the 6-leaf stage were obtained, they were transplanted into both soils (18/04/2015) and irrigated this time with TWW.
The morphological parameters evaluated by the study are: leaf length and width, leaf area, stem length and diameter, number of flowers, number of fruits, fruit size and fresh weight of the fruit.
These morphological parameters evaluated are considered as important indices of yield because the dimensions of the leaves and stems give us information on the quality of the photosynthesis carried out and the other parameters belonging to the reproductive system (flowers and fruits) inform us of quantitative way on productivity (biomass).
The measurements were expressed in term of average and standard deviation using the Microsoft Excel. Student's t-test and Snedecor's F-test were used to examine statistically significant differences in the average heavy metal concentrations and the physicochemical analysis among groups of soil MS and FS. The p < 0.05 was considered as statistically significant.
The granulometric study carried out on both MS and FS using the triangular texture diagram revealed the presence of a difference in the textures of the two substrates as shown in Figure 1.
The FS has a silty-clay-sandy texture, giving it a well-ventilated and lumpy structure suitable for agricultural activities. In addition, this structure lets the excess water flow through and retains a sufficient amount to ensure the proper development of plants and aeration of the root system [21].
The MS texture is loam-clay. We found high levels of clay (40%) and silt (27%) (Figure 1) giving it a more compact structure, less suitable than the FS soil. Indeed, soil compaction as with MS can impact several physicochemical properties of soil such as conductivity or bulk density. It would cause a significant reduction of the poral space thus preventing the good flow of water downward through the higher horizons and thus less available to the roots [22].The state of the clayey soil (MS) characteristic of the area of Mediouna increases its resistance to penetration of the root system. The finer the texture, the more it will form a barrier that is difficult for the roots to cross [21].
In addition, this fine texture causes a compaction that reduces the availability of the raw sap essential to the achievement of photosynthesis thus slowing the growth of the plant [23].This is confirmed by the work done on pepper (Capsicum annuum. L.); a species belonging to the same family as that of the tomato (solanaceae) cultivated on two soils of different textures [24]. This study proved that the silty clay soil of the Beni Mellal region, whose structure is compact, reduced the germination rate of the seeds belonging to this species and even limited the growth of the aerial part, unlike the sandy Maamoura soil which presented better edaphic conditions where this plant developed well. The same results have been observed in the beet (Beta Vulgaris L.), a vegetable crop plant whose hypocotyl develops in the soil, cultivated in two parcels of different grain size compositions and which obtained a better yield in the soil that presented the most suitable structure for its development [25].
Table 1 shows the physicochemical analyzes of both soils, MS and FS, compared to national standards for agricultural soils. Organic matter is distributed in much the same way in both soils. The results of the conductivity (EC) measurement indicate that it is much higher in MS than in FS with values of 1.099 mmhos cm−1 and 0.691 mmhos cm−1 respectively. By referring to the Durand scale of soil classification according to their salinity [26]. These values show that the FS is slightly salty (0.5 ≤ CE < 1mmhos cm−1) which would have an impact only on cultures very sensitive to salts whereas the MS is qualified as saline soil (1 ≤ EC < 2) which would reduce the yield of most crops. The higher average of Sodium (842.5 mg kg−1) of the MS exceed the permissible limits of agricultural soil (480 mg kg−1) by more than 90% according to Moroccan standards. The presence of Sodium in MS causes the decrease of soil permeability [27] and also increases the swelling and dispersing capacity of the clay causing a reduction in macroporosity [28]. The same trends were observed for chlorides that are more abundant in MS (217 mg kg−1) compared to (174.3 mg kg−1) in FS. Since the chlorine present in the substrate competes with the absorption of other essential minerals such as phosphate or nitrate. So, if the level of these macroelements is low in the soil and the chlorine is much more abundant, the plant will not be able to differentiate them and will absorb what is most readily available causing enormous deficiencies for it. The same observation has been observed in tomato individuals exposed to high concentrations of Sodium Chloride (NaCl), which induced a reduction in growth as well as changes in metabolic processes that may go so far as to inhibit seed germination [29]. In the light of the results obtained, the two soils are classified as being alkaline with pH values of 7.94 and 7.89 respectively corresponding to the MS and FS. This is because salty soils have an abundance of hydroxides despite hydrogen ions.
Parameters | Unit | FS | MS | Signification | Limit values ** |
OrganicMatter | % | 2.17 ± 0.02 | 2.95 ± 0.02 | * | 1.5–3 |
EC 1/5 | Mmhoscm−1 | 0.691 ± 0.01 | 1.099 ± 0.01 | * | < 0.5 |
pH (H2O) | - | 7.89 ± 0.01 | 7.94 ± 0.01 | * | 6.5–7.5 |
pH (KCl) | - | 7.49 ± 0.01 | 7.48 ± 0.01 | ns | 5.3 < pH |
Total CaCO3 | % | 11.4 ± 0.1 | 13.3 ± 0.2 | * | < 15 |
Active CaCO3 | % | 4.2 ± 0.01 | 5.5 ± 0.02 | * | < 10 |
CaO | mg kg−1 | 7210 ± 46.37 | 9520 ± 8.02 | * | 2500–5000 |
NH4-N | mg kg−1 | 1 ± 0.02 | 27 ± 0.08 | * | - |
NO3-N | mg kg−1 | 9 ± 0.01 | 4 ± 0.01 | * | 20–30 |
Cl− | mg kg−1 | 174.3 ± 0.82 | 217 ± 0.46 | * | 60–150 |
Na+ | mg kg−1 | 188.7 ± 6.15 | 842.5 ± 10.69 | * | 200–480 |
P2O5 | mg kg−1 | 68.7 ± 6.08 | 41.22 ± 3.68 | * | 30–60 |
K2O | mg kg−1 | 629.6 ± 29.49 | 353 | * | 140–360 |
MgO | mg kg−1 | 246.5 ± 29.64 | 796.8 ± 39.78 | * | 250–500 |
Note: Avearge ± SD, ns: not significant, *: Statistically significant at p ≤ 0.05. **: limit values according to Moroccan standards of agricultural soils. The means are the average of height samples. |
These alkaline soils are equally classified as calcareous soils because of their natural richness in calcium. This is what was found in the MS with very high content levels (9520 mg kg−1), almost double the maximum value admitted in soils for agriculture. The FS also has a high amount of this chemical element (7210 mg kg−1). Certainly, calcium is a nutrient that has several advantages. Firstly, it improves the soil structure and maintains its stability [30]. Secondly, it contributes to the rigidity of plant cell walls and activates certain chemical reactions in the foliar system, thus reducing ammonium nitrate and promoting root growth [31]. However, an excessive concentration of calcium in the soil as is the case in our present study, especially in the MS, could lead to inhibition of growth due to a high content of CaO in the substrate. This would limit the use of the products from photosynthesis and induce their accumulation in the root system [32].
Despite the fact that the MS has a higher ammonium ion load (27 mg kg−1), so that it can be assimilated by plants, it has to be converted into nitrate through nitrifying bacteria. Furthermore, according to the results of analyzes obtained, 9 mg kg−1 of nitrate is found in the FS, which represents twice the quantity of this chemical element in the MS, already ready to be used by the plants and converted into amino acids in the foliar system. This is unlike ammonium, which must be transformed into nitrogenous organic compounds in the roots. This process is accompanied by significant energy expenditure notwithstanding other vital processes of the plant such as fruiting or growth [33]. Another advantage in favor of nitrate is that the latter promotes the absorption of certain cations such as potassium, calcium, and magnesium while ammonium competes with these chemical elements.
Both FS and MS contain average levels of phosphoric acid (P2O5) according to soil standards for agriculture. These phosphorus concentrations favor the physiological activities of the plant acting on several levels: it guarantees a harmonious growth of the plant with rigidity of the cellular tissues as well as a good yield in terms of the quality of the fruit [34].
The abundance of K2O is also an advantage in favor of FS. It is favorable to plants since it regulates the cellular exchanges, stimulates the realization of the photosynthesis, in particular the production of the carbohydrates in the foliar system, and participates in the elaboration of the proteins in reserve organs such as the fruit [35]. An average content of MgO (246.5 mg kg−1) was found in the FS soil, representing three times less than in MS (796.8 mg kg−1).
Table 2 shows the concentrations of heavy metals in both soils compared to the required limit values. We chose the latest Finnish legislation [36] as it represents a good approximation of the average values of different European national systems, and in addition has been applied internationally for agricultural soils [37].
Heavy metals (mg kg−1) | FS | MS | Signification | Limit values ** |
Cu | 6.67 ± 0.12 | 1.7 ±0.10 | * | 100 |
Mn | 20.12 ± 0.03 | 11.7 ± 0.11 | * | 10–20 |
Fe | 6.6 ± 0.02 | 4.7 ± 0.02 | * | 40–100 |
Zn | 19.7 ± 0.02 | 6 ± 0.04 | * | 200 |
Cr | 18.75 ± 5.06 | 21.5 ± 5.71 | ns | 100 |
Pb | 16.01 ± 3.2 | 19 ± 1.54 | ns | 60 |
Cd | 0.086 ± 0.01 | 0.05 ± 0.01 | * | 1 |
Ni | 10.65 ± 3.50 | 13.75 ± 2.62 | ns | 50 |
Hg | 0.048 ±0.01 | 0.065 ± 0.004 | ns | 0.5 |
As | 0.082 ± 0.01 | 0.07 ± 0.005 | ns | 5 |
Co | 7.8 ± 2.21 | 12 ± 1.67 | ns | 20 |
Note: Avearge ± SD, ns: not significant, *: Statistically significant at p ≤ 0.05. **: [36]. The means are the average of height samples. |
As for heavy metals such as copper, manganese and zinc, we note that their contents at MS and FS are included in the required intervals: Cu < 100, 10 ≤ Mn ≤ 20, Zn < 200 respectively (Table 2).
In addition, the low iron content in both soils can be explained by their calcareous nature. It is likely that his richness in calcium also leads to a disrupted uptake of this trace element by the plants.
A deficit or excess of these mineral elements is likely to be responsible for low agricultural yields. Plants ensure their growth and development by absorbing most of the trace elements accumulated in the soil [38] because certain metal ions such as copper, zinc, and manganese are part of the constituents of certain enzymes or act as cofactors [39].
For both soil types, heavy metal concentrations of Cr, Pb, Ni, Hg and Co remain below the limit values for soils to be used for agricultural activities [36], with an abundance more visible in MS than in that of SF except for Cadmium (0.086 mg kg−1) and Arsenic (0.082 mg kg−1) which are more present in the SF soil.
The physicochemical results of the treated wastewater show that the hydrogen potential of this water is slightly alkaline (pH = 7.32) (Table 3). This pH corresponds perfectly to this agricultural activity since its value is within the range required by Moroccan standards [11].The TSS levels approaching 30 mg L−1 present no risk of clogging according to FAO standards [27]. These values are also tolerated by the [11] which imposes a maximum threshold of 200 mg L−1.
Physicochemical parameters | Unit | TWW | Limit values |
pH | - | 7.32 ±0.2 | 6.5–8.4* |
TSS | mg L−1 | 30 ± 4.58 | 200* |
EC | µs cm−1 | 3910 ± 76.03 | 12000* |
COD | mg L−1 | 85 ± 12.76 | 90* |
BOD5 | mg L−1 | 21 ± 9.49 | 30* |
NTK | mg L−1 | 22 ± 2.81 | - |
Total P | mg L−1 | 8.7 ± 1.03 | - |
PO4-P | mg L−1 | 5 ± 0.46 | 2** |
SO4− | mg L−1 | 275 ± 0.9 | 250* |
NO3− | mg L−1 | 22.15 ± 0.56 | 30* |
NO2− | mg L−1 | 0.01 ± 0.002 | 5*, 3** |
F- | mg L−1 | 0.2 ± 0.16 | 1* |
Al | mg L−1 | 0.045 ± 0.011 | 5* |
Total Cr | mg L−1 | 0.016 ± 0.003 | 1* |
Ar | mg L−1 | 0.027 ± 0.006 | - |
Ba | mg L−1 | 0.034 ± 0.006 | - |
Co | mg L−1 | 0.013 ± 0.001 | 0.5* |
Cr 6 | mg L−1 | 0.01 ± 0.001 | - |
Sn | mg L−1 | 0.0011 ± 0.0004 | - |
Mn | mg L−1 | 0.046 ± 0.002 | 0.2* |
Fe | mg L−1 | 0.056 ± 0.009 | 5* |
Hg | mg L−1 | 0.001 ± 0.0002 | 0.001* |
Cu | mg L−1 | 0.016 ± 0.002 | 2* |
Cd | mg L−1 | 0.0014 ± 0.0003 | 0.01* |
Zn | mg L−1 | 0.23 ± 0.001 | 2* |
Pb | mg L−1 | 0.025 ± 0.003 | 5* |
*: Limit values [11], **: Limit values [42]. Means are the average of three samples. |
The electrical conductivity (EC) value of this irrigation water remains tolerable by the tomato plant whose yield decreases by 10% from an electrical conductivity of 5000 μs cm−1, the equivalent of 2.5 g L−1 [40].
The chemical oxygen demand (COD) remains acceptable at the threshold set by the [11] which proves the presence of chemical pollution which may be due to the use of pesticides since the WWTP is in an agricultural zone (province of Mediouna). The same observation is valid concerning the BOD5, which meets the standards required for water intended for irrigation demonstrating the presence of rejected organic matter of domestic origin, such as fecal matter [41].
Sulphate concentrations were slightly lower than the reference values (275–250 mg L−1). The load of this water in terms of nitrates is acceptable (below the required limit values) and will contribute positively to the good development of tomato plants by providing them with essential quantities of these minerals vital for their growth.
The findings show that all recorded heavy metal values remain below the standards required by [11] except for mercury which has a value at the limit of reference levels (Table 3). It is deduced that this water is adequate for agricultural use provided that there is a reduction in the load of mercury, which can increase in the horizons of the soil over time through successive and continuous irrigations. In addition, it can accumulate in the underground vegetative system through root absorption, even spreading to the aerial system (leaf, stalk or even fruit). This is the phenomenon of bioaccumulation, as confirmed by studies [43,44].
We followed the growth of the aerial vegetative body of tomato plants grown in both soils: MS and FS throughout the experimental season (Figures 2, 3). The results obtained show that the length and width of the leaves increase with time showing greater growth in FS. At the end of the farming season, the final length reached in tomato leaves is 12 cm (FS) compared to only 8 cm (MS). The width obtained in July in both plants is 5.5 cm and 3.9 cm respectively for FS and MS (Figures 2A, B). Statistical analysis revealed the presence of a significant growth difference between FS and MS for both length (F = 0.77 and p = 0.414) and width (F = 0.86 and p = 0.390). This difference was much more visible from the month of June. The leaf area obtained in the plants grown at FS is equivalent to twice the leaf development observed in MS with averages of 33.4343 ± 5.15 cm2 and 16.87 ± 1.53cm2 respectively (Figure 3A).This morphological parameter is clearly influenced by the nature of the soil (p < 0.0001).
We also observed an increase in stem size over time (Figures 4A, B). The height of the plant grown at FS and MS at the end of the experiment averaged was respectively 73.87 ± 2.41 cm and 55.68 ± 3.73 cm (Figure 3B). Statistical analysis showed the presence of a significant difference between the two soils (F = 0.28, p = 0.618).
This difference in growth is due to several factors: among which we can mention the difference of texture, because the FS is characterized by a silty-clay-sandy texture which gives a good aeration of the soil allowing the roots to penetrate it easily. In contrast, the MS is characterized by the dominance of clay followed by silt making this geological support little aerated while retaining a large quantity of water and therefore less permeable and suitable than the FS. The impact of the mechanical constraints generates a reduction of growth and root development [45,46], which leads to low water and mineral absorption by the root system. This means a decrease in the efficiency of resource use and thus the growth of the vegetative system (stem and leaf) will be directly affected [47,48,49]. Another consequence of this compaction of the soil is of the hormonal type, it corresponds to the sending of a negative signal, such as abscisic acid (ABA), by the root towards the aerial system [50].
In response to this signal, several phenomena are observed, namely: a reduction in the osmotic potential of the root, which leads to an increase in turgor pressure and a decrease in the number of cells [51]. Other effects include increased stomata resistance [45,52], depletion of leaf nutrient content, and decreased leaf elongation[21].
The important load of Sodium is also another factor likely to be the cause of this difference of growth observed between the two soils. Sodium in the MS exceeds 7 times that of the FS (842.5 mg kg−1 vs. 188.7 mg kg−1) and chloride is always more abundant in the MS (217.46 mg kg−1). This would reduce the permeability of the soil and inhibit the good absorption of the raw sap since the osmotic pressure of the soil will be much greater than that of the absorbent hairs of the piliferous zone of the roots. In addition, the notable decrease in the size of various organs such as the leaf system and the height of the stem is a kind of morphological adaptation and also resistance to salt stress that this plant undergoes [28].
A wealth of assimilable elements and trace elements is still in favor of FS which makes its nutrients available to plants in sufficient quantities. The average potassium content in plants grown on MS reduces the efficiency of photosynthesis since this chemical element intervenes in the smooth functioning of stomata by regulating their closing and opening and thus promotes the realization of photosynthetic gas exchange allowing a production of organic materials. In addition, it ensures a flow of water in the plant particularly by regulating the pH level of the cells and the osmotic pressure. Potash is involved in the development of several molecules essential for photosynthesis such as ATP and enzymes.
In addition to the differences observed in the vegetative body of these plants, a significant difference was noted also for the reproductive parts with the plants grown in FS showing a higher yield.
The number of flowers obtained in the FS is 22.20 ± 3.075, while 16.80 ± 2.042 is found in the MS (Figure 5A). Concerning the number of fruits, the values are of the order of 17.40 ± 1.95 and 9.47 ± 2.167 for the FS and MS respectively. These two morphological parameters are strongly dependent on the nature of the soil (p < 0.0001). The size of the fruits obtained shows a significant difference 30.31 ± 2.96 cm2 for FS versus 23.22 ± 2.09 cm2 for MS (Figure 5B). The observation is idem for fresh weight which is higher in plants grown in FS (135.87 ± 10.69 g) and only (96.43 ± 7.22 g) in that of MS (Figure 5C). This proves that these tomato characteristics are mainly conditioned by edaphic factors (p < 0.0001).
The decrease in productivity experienced by tomato plants grown in MS can be explained by the nature of the soil substrate as its compact structure limited the water supply causing water stress that is poorly supported by the tomato plant, which then reduces the number of flower buds formed. This is in line with the work of [53] who showed that a decrease in the soil water content delayed the beginning of flowering by decreasing its speed and that the development of the reproductive system was more affected than the vegetative system in response to a water deficit. In fact, the flowering and fruit setting periods are among the most sensitive stages of water stress. The lack of availability of this resource because of the soil texture and its high salt loading which induces salt stress make the yield much lower in the MS with relatively small fresh sizes and weights. These results corroborate those of [54] who claim that an accumulation of salts in the plant decreased fruit yield by affecting the formation and viability of the reproductive organs. This decrease in productivity is manifested by a reduction in the fresh weight of tomato given the difficulty for water to accumulate in the fruit in the presence of high salinity, since the flow of water is restricted by a low water potential. This decrease in the fresh weight observed is due to its weak growth during the cell expansion phase following the increase of the osmotic potential in the root system as well as the slowing down of the fruit xylem development. Simply by reducing the amount of organic matter produced, the plant saves energy in order to bring water in opposition to the osmosis law through active transport. This is completely different from tomatoes planted in the FS, which is airy, has a suitable structure, and is rich enough in nutrients essential for the growth of these plants.
The richness of the FS in nitrates, and in certain trace elements and assimilable elements, such as phosphorus, potash, copper and zinc, promotes an early flowering as well as an effective fruiting which results in a substantial number of large fruits with a high fresh weight. This is in line with work carried out on tomatoes amended with compost [55], which showed that a sufficient supply of nitrogen present in organic fertilizers would profusely enhance the yield of the crop, because nitrate is the mineral source of nitrogen best assimilated by plants. The absorbed potassium promotes both a qualitative and quantitative enhancement of the fruit. During the fruiting stage, the accumulation of phosphorus brought to the fruit by the soil is much higher than that brought to the vegetative system, namely the leaves and branches.
The results obtained demonstrated that the physicochemical characteristics of the soil significantly influence the development and growth of cultivated plants as well as the quality of the tomato fruit. In fact, the yields obtained in the FS are more satisfactory than those of the MS. Meanwhile, the results are encouraging as the small imperfections of the MS are remediable. Its fine texture and compact structure can be improved by an additional supply of humus and compost which will not only eliminate its mechanical stresses, but will even enrich it with mineral and organic substances making it more fertile. This will countermeasure the low concentrations of trace elements that inhibited photosynthesis and resulted in inadequate morphological results compared to the FS, such as smaller fruit sizes and shorter stem lengths. In addition, the supply of organic matter proves to be essential because by improving the structure of the soil, the elimination of accumulated salts would be facilitated. A high load of salts such as sodium may also be attenuated by the use of low salt water to limit the growth of excess salinity. Another alternative may be implemented, such as the renewal of the earth to a depth of about ten centimeters, which would promote the growth of some plants intolerant to high salinities that have accumulated in the upper horizons of the soil.
The study of water parameters revealed that the use of this treated wastewater from the Mediouna (WWTP) can be envisaged as part of a circular economy that aims to: recycle waste in order to compensate for the lack of this resource water rich in minerals essential for the development of elaborated sap providing that:
—The mercury and sulphate levels in this irrigation water are reduced.
—Studies on the microbiological quality of fruits are further extended.
Finally, more work is being carried out on other species belonging to the same genus Solanaceae, in order to evaluate the physicochemical quality of the fruits resulting from the use of treated waste water, to guarantee their safety, and to avoid any bacteriological contamination or bioaccumulation of heavy metals that may endanger public health.
The authors declare no conflict of interest in this paper.
[1] | El Kettani S, Azzouzi M (2006) Prevalence of helminths among a rural population using wastewater for agricultural production in Settat (Morocco). Environ, Risks & Health 5: 99-106. |
[2] | Benabdellaoui Y, Chlaida M, Zabari M (2001) Impact of hydraulic developments on thewater and sediment quality in the Oued Oum Er Rbia estuary (Atlantic coast, Morocco). Bull Sci Inst, Rabat, Life Sci Sect 23: 71-76. |
[3] | Benmessaoud F (2007) Physico-chemical, metallic and bacteriological quality of the waters of the Bouregregreg estuary and impact on the biology and demography of Venerupis Decussata (LINNE, 1758) and Cardium Edule (LINNE, 1767), State Doctorate Thesis, Faculty of Sciences Rabat. |
[4] | Derwich E, Beziane Z, Benaabidate L, et al. (2008) Assessment of the quality of surface water from Oued Fès and Sebou used in market gardening in Morocco. Larhyss J 7: 59-77. |
[5] | Fouad S, Hajjami K, Cohen N, et al. (2014) Physico-chemical quality and metallic contamination of the waters of Oued Hassar: impacts of wastewater from the town of Mediouna (suburban Casablanca, Morocco). Afr Sc: Int Rev Sci Technol 10: 91-102. |
[6] | Nhaili A, Sakhraoui N, Hebabaze, S, et al. (2016) Biological membrane treatment and agricultural reuse of waste water from Mediouna (Casablanca, Morocco). J Mater Environ Sci 7: 40-49. |
[7] | Gatta, G, Libutti, A, Gagliardi, A, et al. (2015) Treated agro-industrial wastewater irrigation of tomato crop: Effects on qualitative/quantitative characteristics of production and microbiological properties of the soil. Agric Water Manage 149: 3-4. |
[8] | MAPMDREF (Ministry of Agriculture, Maritime Fisheries, Rural Development and Waters and Forests) (2016) Available from: http://www.agriculture.gov.ma/sites/default/files/agriculture_en_chiffres_2016_.pdf. |
[9] | Disciglio G, Gatta G, Libutti A, et al. (2015) Effects of irrigation with treated agro-industrial wastewater on soil chemical characteristics and fungal populations during processing tomato crop cycle. J Soil Sci Plant Nutr 15: 765-780. |
[10] | Alghobar MA, Suresha S (2017) Evaluation of metal accumulation in soil and tomatoes irrigated with sewage water from Mysore city, Karnataka, India. J Saudi Soc of Agric Sci 16: 49-59. |
[11] | S.E.E.E (State Secretariat at the Ministry of Energy, Mines, Water and Environment) (2007) Dirctorate of Research and Water Planning, Water quality standards for water irrigation, Rabat. |
[12] | APHA (2005) Standard methods for the examination of water and wastewater, In: Eaton, AD, Clesceri LS, Rice EW, et al., American Public Health Association (APHA), American Water Works Association (AWWA) and Water Environment Federation (WEF), Washington, D.C., USA. |
[13] | Walkley A, Black IA (1934) An examination of the Degtjareff method for deter-mining organic carbon in soils: Effect of variations in digestion conditions and of inorganic soil constituents. Soil Sci 63: 251-263. |
[14] | Bremner JM, Keeney DR (1996) Determination and isotope-ratio analysis for different forms of nitrogen in soils: 3. exchangeable ammonium, nitrate, and nitrite by extraction-distillation methods. Proc Soil Sci Soc Am 30: 577-582. |
[15] | Richards LA (1954) Diagnosis and improvement of saline and alkali soils. Agricultural handbook No. 60.United Stated Department of Agriculture (USDA), Washington, DC, 166. |
[16] | Olsen SR, Sommers LE (1982) Phosphorus. In: Page AL, Miller RH, Keeney DR, Methods of Soil Analysis, part 2, Chemical and Microbiological Properties. American Society of Agronomy, Madison, WI, 403-430. |
[17] |
Öblad M, Standzenieks P, Selin E, et al. (1982) Application of an energy dispersive X-Ray fluorescence spectrometer to air pollution studies. Phys. Scripta 26: 257-261. doi: 10.1088/0031-8949/26/4/001
![]() |
[18] | Black CA, Evans DD, Dinauer RC (1965) Methods of Soil Analysis, American Society of Agronomy, Madison, WI, 653-708. |
[19] | Loeppert RH, Suarez DL (1996) Carbonate and gypsum. In: Sparks DL, Methods of Soil Analysis, Part 3. Chemical Methods, Madison, Wisconsin, USA, 437-474. |
[20] |
Toor RK, Savage GP, Heeb A (2006) Influence of different types of fertilisers on the major antioxidant components of tomatoes. J Food Compos Anal 19: 20-27. doi: 10.1016/j.jfca.2005.03.003
![]() |
[21] | Mirleau-Thebaud V (2012) Effects of soil mechanical stresses on limiting sunflower yields. PhD thesis, University of Toulouse, National Polytechnic Institute of Toulouse, France. |
[22] | Richard G, Cousin I, Sillon, JF, et al. (2001) Effect of compaction on the porosity of a silty soil: Influence on unsaturated hydraulic properties. Eur J Soil Sci 52: 49-58. |
[23] |
Marschner P, Kandeler E, Marschner B (2003) Structure and function of the soil microbial community in a long-term fertilizer experiment. Soil Biol Biochem 35: 453-461. doi: 10.1016/S0038-0717(02)00297-3
![]() |
[24] | Hbaiz EM, Ouihman, EM, Lebkiri M, et al. (2014) Effect sewage of wastewater from the treatment plant on the growth in pepper (Capsicum annuum. L. ) Cultivated on two different grounds. J Mater Environ Sci 5: 1376-1381. |
[25] | Ez-Zarhouny D, Hbaiz E, Lebkiri M, et al. (2015) Evaluation of the impact of wastewater from a wastewater treatment plant on the development of red beet (beta vulgaris) grown on two different soils. Larhyss J 23:105-115. |
[26] | Durand JH (1983) Irrigable soils. Soil study, University Press of France, Agency for Cultural and Technical Cooperation, France, 338. |
[27] | Bedouh Y (2014) Assessment of the toxicity of wastewater treated by the Guelma wastewater treatment plant and its impact on onions (Allium cepa). PhD thesis, University of Annaba, Algeria, 128. |
[28] | Derkaoui KM (2011) Morphological, physiological and anatomical responses of tomato roots (Solanum lycopersicum L.) to saline stress. PhD Thesis, Oran University 1 Ahmed Ben Bella, Algeria, 109 p. |
[29] | Arbaoui M, Yahia N, Belkhodja M (2015) Germination of the tomato (Lycopersicon esculentum Mill.) in response to salt stress combined with hormones. Int J Agron Agricl Res 7: 14-24. |
[30] | Cristelo N, Glendinning S, Fernandes L, et al. (2012) Effect of calcium content on soil stabilisation with alkaline activation. Constr Build Mater 29: 167-174. |
[31] | Hepler PK (2005) Calcium: A central regulator of plant growth and development. The Plant Cell 17: 2142-2155. |
[32] | Giel P, Bojarczuk K (2011) Effects of high concentrations of calcium salts in the substrate and its pH on the growth of selected rhododendron cultivars. Acta Soc Bot Pol 80: 105-114. |
[33] | Evans RD (2001) Physiological mechanisms influencing plant nitrogen isotope composition. Trends Plant Sci 6: 121-126. |
[34] | Shen J, Yuan L, Zhang J, et al. (2011) Phosphorus dynamics: From soil to plant. Plant Physiol 156: 997-1005. |
[35] | Wang M, Zheng Q, Shen Q, et al. (2013) The critical role of potassium in plant stress response. Int J Mol Sci 14: 7370-7390. |
[36] | MEF (Ministry of the Environment, Finland) (2007) Government decree on the assessment of soil contamination and remediation needs, Finland. |
[37] | Van der Voet E, Salminen R, Eckelman M, et al. (2013) Environmental challenges of anthropogenic metals flows and cycles, United Nations Environment Programme, 235. |
[38] | Temgoua E, Tsafack HN, Pfeifer HR, et al. (2015) Contents of major elements and trace elements in soil and some market gardening crops in the city of Dschang, Cameroon. Afr Crop Sci J 23: 35-44. |
[39] |
Yang X, Feng Y, He Z, et al. (2005) Molecular mechanisms of heavy metal hyperaccumulation and phytoremediation. J Trace Elem Med Biol 18: 339-353. doi: 10.1016/j.jtemb.2005.02.007
![]() |
[40] | PNTTA (National Program for the Transfer of Technology in Agriculture) (2003) Technical Data Cards V, Tomato, Eggplant, Pepper and Gumbo. |
[41] | Belghyti D, El Guamri Y, Ztit G, et al. (2009) Physicochemical characterization of slaughterhouse wastewater in order to implement adequate treatment: case of Kenitra in Morocco. Afr Sci: Int Rev Sci Technol 5: 199-216. |
[42] | FAO (Food and Agriculture Organisation) (1992) Wastewater treatment and use in agriculture. In: Pescod MB, Irrigation and Drainage, Paper 47, Rome. |
[43] |
Khan A, Khan S, Khan MA, et al. (2015) The uptake and bioaccumulation of heavy metals by food plants, their effects on plants nutrients, and associated health risk: A review. Environ Sci Pollut Res 22: 13772-13799. doi: 10.1007/s11356-015-4881-0
![]() |
[44] | Ruchuwararak P, Intamat S, Tengjaroenkul B, et al. (2018) Bioaccumulation of heavy metals in local edible plants near a municipal landfill and the related human health risk assessment. Human and Ecological Risk Assessment: An International Journal, 1-13. |
[45] | Lipiec J, Hatano R (2003) Quantification of compaction effects on soil physical properties and crop growth. Geoderma 116: 0-136. |
[46] |
Sadras VO, O'Leary GJ, Roget DK (2005) Crop responses to compacted soil: capture and efficiency in the use of water and radiation. Field Crops Res 91: 131-148. doi: 10.1016/j.fcr.2004.06.011
![]() |
[47] | Passioura JB (2002) Soil conditions and plant growth. Plant, Cell & Environ 25: 311-318. |
[48] | Clark RN, Swayze GA, Livo KE, et al. (2003) Imaging spectroscopy: Earth and planetary remote sensing with the USGS Tetracorder and expert systems. J Geophys Res: Planets 108: 1-44. |
[49] | Hodge A, Berta G, Doussan C, et al. (2009) Plant root growth, architecture and function. Plant Soil 321: 153-187. |
[50] | Tardieu F (1994) Growth and functioning of roots and of root systems subjected to soil compaction. Towards a system with multiple signalling? Soil Tillage Res 30: 217-243. |
[51] | Bengough AG, Croser C, Pritchard J (1997) A biophysical analysis of root growth under mechanical stress, In: Plant Roots-From Cells to Systems, Springer, Dordrecht, 107-116. |
[52] |
Håkansson I, Lipiec J (2000) A review of the usefulness of relative bulk density values in studies of soil structure and compaction. Soil Tillage Res 53: 71-85. doi: 10.1016/S0167-1987(99)00095-1
![]() |
[53] | Koch M, Kruse J, Eichler-Löbermann B, et al. (2018) Phosphorus stocks and speciation in soil profiles of a long-term fertilizer experiment: Evidence from sequential fractionation, P K-edge XANES, and 31P NMR spectroscopy. Geoderma 316: 115-126. |
[54] | Maaouia-Houimli, SI, Denden M, Dridi-Mouhandes B, et al. (2011) Characteristics of fruit growth and production in three varieties of pepper (Capsicum annuum L.) under salt stress. Tropicultura 29: 75-81. |
[55] | Kitabala MA, Tshala UJ, Kalenda MA, et al. (2016) Effects of different compost doses on the production and profitability of tomato (Lycopersicon esculentum Mill) in the city of Kolwezi, Lualaba Province (DR Congo). J Appl Biosci 102: 9669-9679. |
1. | Aliya MAXOTOVA, Elmira NURBAYEVA, тemirzhan AİTBAYEV, Balseker NURGALİYEVA, Yield and yield components of five tomato varieties (Solanum lycopersicum) as influenced by chemical NPK fertilizer applications under chestnut soil conditions, 2021, 10, 2147-4249, 327, 10.18393/ejss.962545 | |
2. | Ermias Alayu, Seyoum Leta, Evaluation of irrigation suitability potential of brewery effluent post treated in a pilot horizontal subsurface flow constructed wetland system: implications for sustainable urban agriculture, 2021, 7, 24058440, e07129, 10.1016/j.heliyon.2021.e07129 | |
3. | Eugenio Cozzolino, Antonio Salluzzo, Luisa del Piano, Alessio Vincenzo Tallarita, Vincenzo Cenvinzo, Antonio Cuciniello, Antonietta Cerbone, Pasquale Lombardi, Gianluca Caruso, Effects of the Application of a Plant-Based Compost on Yield and Quality of Industrial Tomato (Solanum lycopersicum L.) Grown in Different Soils, 2023, 13, 2076-3417, 8401, 10.3390/app13148401 |
Parameters | Unit | FS | MS | Signification | Limit values ** |
OrganicMatter | % | 2.17 ± 0.02 | 2.95 ± 0.02 | * | 1.5–3 |
EC 1/5 | Mmhoscm−1 | 0.691 ± 0.01 | 1.099 ± 0.01 | * | < 0.5 |
pH (H2O) | - | 7.89 ± 0.01 | 7.94 ± 0.01 | * | 6.5–7.5 |
pH (KCl) | - | 7.49 ± 0.01 | 7.48 ± 0.01 | ns | 5.3 < pH |
Total CaCO3 | % | 11.4 ± 0.1 | 13.3 ± 0.2 | * | < 15 |
Active CaCO3 | % | 4.2 ± 0.01 | 5.5 ± 0.02 | * | < 10 |
CaO | mg kg−1 | 7210 ± 46.37 | 9520 ± 8.02 | * | 2500–5000 |
NH4-N | mg kg−1 | 1 ± 0.02 | 27 ± 0.08 | * | - |
NO3-N | mg kg−1 | 9 ± 0.01 | 4 ± 0.01 | * | 20–30 |
Cl− | mg kg−1 | 174.3 ± 0.82 | 217 ± 0.46 | * | 60–150 |
Na+ | mg kg−1 | 188.7 ± 6.15 | 842.5 ± 10.69 | * | 200–480 |
P2O5 | mg kg−1 | 68.7 ± 6.08 | 41.22 ± 3.68 | * | 30–60 |
K2O | mg kg−1 | 629.6 ± 29.49 | 353 | * | 140–360 |
MgO | mg kg−1 | 246.5 ± 29.64 | 796.8 ± 39.78 | * | 250–500 |
Note: Avearge ± SD, ns: not significant, *: Statistically significant at p ≤ 0.05. **: limit values according to Moroccan standards of agricultural soils. The means are the average of height samples. |
Heavy metals (mg kg−1) | FS | MS | Signification | Limit values ** |
Cu | 6.67 ± 0.12 | 1.7 ±0.10 | * | 100 |
Mn | 20.12 ± 0.03 | 11.7 ± 0.11 | * | 10–20 |
Fe | 6.6 ± 0.02 | 4.7 ± 0.02 | * | 40–100 |
Zn | 19.7 ± 0.02 | 6 ± 0.04 | * | 200 |
Cr | 18.75 ± 5.06 | 21.5 ± 5.71 | ns | 100 |
Pb | 16.01 ± 3.2 | 19 ± 1.54 | ns | 60 |
Cd | 0.086 ± 0.01 | 0.05 ± 0.01 | * | 1 |
Ni | 10.65 ± 3.50 | 13.75 ± 2.62 | ns | 50 |
Hg | 0.048 ±0.01 | 0.065 ± 0.004 | ns | 0.5 |
As | 0.082 ± 0.01 | 0.07 ± 0.005 | ns | 5 |
Co | 7.8 ± 2.21 | 12 ± 1.67 | ns | 20 |
Note: Avearge ± SD, ns: not significant, *: Statistically significant at p ≤ 0.05. **: [36]. The means are the average of height samples. |
Physicochemical parameters | Unit | TWW | Limit values |
pH | - | 7.32 ±0.2 | 6.5–8.4* |
TSS | mg L−1 | 30 ± 4.58 | 200* |
EC | µs cm−1 | 3910 ± 76.03 | 12000* |
COD | mg L−1 | 85 ± 12.76 | 90* |
BOD5 | mg L−1 | 21 ± 9.49 | 30* |
NTK | mg L−1 | 22 ± 2.81 | - |
Total P | mg L−1 | 8.7 ± 1.03 | - |
PO4-P | mg L−1 | 5 ± 0.46 | 2** |
SO4− | mg L−1 | 275 ± 0.9 | 250* |
NO3− | mg L−1 | 22.15 ± 0.56 | 30* |
NO2− | mg L−1 | 0.01 ± 0.002 | 5*, 3** |
F- | mg L−1 | 0.2 ± 0.16 | 1* |
Al | mg L−1 | 0.045 ± 0.011 | 5* |
Total Cr | mg L−1 | 0.016 ± 0.003 | 1* |
Ar | mg L−1 | 0.027 ± 0.006 | - |
Ba | mg L−1 | 0.034 ± 0.006 | - |
Co | mg L−1 | 0.013 ± 0.001 | 0.5* |
Cr 6 | mg L−1 | 0.01 ± 0.001 | - |
Sn | mg L−1 | 0.0011 ± 0.0004 | - |
Mn | mg L−1 | 0.046 ± 0.002 | 0.2* |
Fe | mg L−1 | 0.056 ± 0.009 | 5* |
Hg | mg L−1 | 0.001 ± 0.0002 | 0.001* |
Cu | mg L−1 | 0.016 ± 0.002 | 2* |
Cd | mg L−1 | 0.0014 ± 0.0003 | 0.01* |
Zn | mg L−1 | 0.23 ± 0.001 | 2* |
Pb | mg L−1 | 0.025 ± 0.003 | 5* |
*: Limit values [11], **: Limit values [42]. Means are the average of three samples. |
Parameters | Unit | FS | MS | Signification | Limit values ** |
OrganicMatter | % | 2.17 ± 0.02 | 2.95 ± 0.02 | * | 1.5–3 |
EC 1/5 | Mmhoscm−1 | 0.691 ± 0.01 | 1.099 ± 0.01 | * | < 0.5 |
pH (H2O) | - | 7.89 ± 0.01 | 7.94 ± 0.01 | * | 6.5–7.5 |
pH (KCl) | - | 7.49 ± 0.01 | 7.48 ± 0.01 | ns | 5.3 < pH |
Total CaCO3 | % | 11.4 ± 0.1 | 13.3 ± 0.2 | * | < 15 |
Active CaCO3 | % | 4.2 ± 0.01 | 5.5 ± 0.02 | * | < 10 |
CaO | mg kg−1 | 7210 ± 46.37 | 9520 ± 8.02 | * | 2500–5000 |
NH4-N | mg kg−1 | 1 ± 0.02 | 27 ± 0.08 | * | - |
NO3-N | mg kg−1 | 9 ± 0.01 | 4 ± 0.01 | * | 20–30 |
Cl− | mg kg−1 | 174.3 ± 0.82 | 217 ± 0.46 | * | 60–150 |
Na+ | mg kg−1 | 188.7 ± 6.15 | 842.5 ± 10.69 | * | 200–480 |
P2O5 | mg kg−1 | 68.7 ± 6.08 | 41.22 ± 3.68 | * | 30–60 |
K2O | mg kg−1 | 629.6 ± 29.49 | 353 | * | 140–360 |
MgO | mg kg−1 | 246.5 ± 29.64 | 796.8 ± 39.78 | * | 250–500 |
Note: Avearge ± SD, ns: not significant, *: Statistically significant at p ≤ 0.05. **: limit values according to Moroccan standards of agricultural soils. The means are the average of height samples. |
Heavy metals (mg kg−1) | FS | MS | Signification | Limit values ** |
Cu | 6.67 ± 0.12 | 1.7 ±0.10 | * | 100 |
Mn | 20.12 ± 0.03 | 11.7 ± 0.11 | * | 10–20 |
Fe | 6.6 ± 0.02 | 4.7 ± 0.02 | * | 40–100 |
Zn | 19.7 ± 0.02 | 6 ± 0.04 | * | 200 |
Cr | 18.75 ± 5.06 | 21.5 ± 5.71 | ns | 100 |
Pb | 16.01 ± 3.2 | 19 ± 1.54 | ns | 60 |
Cd | 0.086 ± 0.01 | 0.05 ± 0.01 | * | 1 |
Ni | 10.65 ± 3.50 | 13.75 ± 2.62 | ns | 50 |
Hg | 0.048 ±0.01 | 0.065 ± 0.004 | ns | 0.5 |
As | 0.082 ± 0.01 | 0.07 ± 0.005 | ns | 5 |
Co | 7.8 ± 2.21 | 12 ± 1.67 | ns | 20 |
Note: Avearge ± SD, ns: not significant, *: Statistically significant at p ≤ 0.05. **: [36]. The means are the average of height samples. |
Physicochemical parameters | Unit | TWW | Limit values |
pH | - | 7.32 ±0.2 | 6.5–8.4* |
TSS | mg L−1 | 30 ± 4.58 | 200* |
EC | µs cm−1 | 3910 ± 76.03 | 12000* |
COD | mg L−1 | 85 ± 12.76 | 90* |
BOD5 | mg L−1 | 21 ± 9.49 | 30* |
NTK | mg L−1 | 22 ± 2.81 | - |
Total P | mg L−1 | 8.7 ± 1.03 | - |
PO4-P | mg L−1 | 5 ± 0.46 | 2** |
SO4− | mg L−1 | 275 ± 0.9 | 250* |
NO3− | mg L−1 | 22.15 ± 0.56 | 30* |
NO2− | mg L−1 | 0.01 ± 0.002 | 5*, 3** |
F- | mg L−1 | 0.2 ± 0.16 | 1* |
Al | mg L−1 | 0.045 ± 0.011 | 5* |
Total Cr | mg L−1 | 0.016 ± 0.003 | 1* |
Ar | mg L−1 | 0.027 ± 0.006 | - |
Ba | mg L−1 | 0.034 ± 0.006 | - |
Co | mg L−1 | 0.013 ± 0.001 | 0.5* |
Cr 6 | mg L−1 | 0.01 ± 0.001 | - |
Sn | mg L−1 | 0.0011 ± 0.0004 | - |
Mn | mg L−1 | 0.046 ± 0.002 | 0.2* |
Fe | mg L−1 | 0.056 ± 0.009 | 5* |
Hg | mg L−1 | 0.001 ± 0.0002 | 0.001* |
Cu | mg L−1 | 0.016 ± 0.002 | 2* |
Cd | mg L−1 | 0.0014 ± 0.0003 | 0.01* |
Zn | mg L−1 | 0.23 ± 0.001 | 2* |
Pb | mg L−1 | 0.025 ± 0.003 | 5* |
*: Limit values [11], **: Limit values [42]. Means are the average of three samples. |