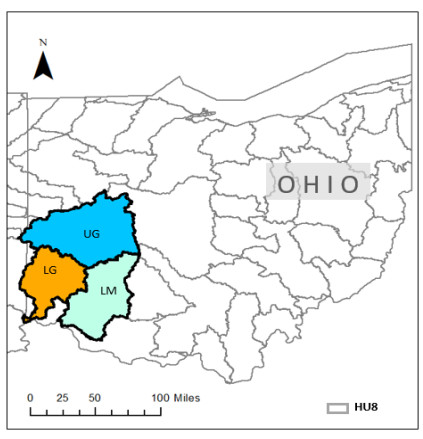
Citation: Eric Ariel L. Salas, Sakthi Kumaran Subburayalu. Implications of climate change on nutrient pollution: a look into the nitrogen and phosphorus loadings in the Great Miami and Little Miami watersheds in Ohio[J]. AIMS Environmental Science, 2019, 6(3): 186-221. doi: 10.3934/environsci.2019.3.186
[1] | Dong Chen, Marcus Thatcher, Xiaoming Wang, Guy Barnett, Anthony Kachenko, Robert Prince . Summer cooling potential of urban vegetation—a modeling study for Melbourne, Australia. AIMS Environmental Science, 2015, 2(3): 648-667. doi: 10.3934/environsci.2015.3.648 |
[2] | Megan K. Creutzburg, Emilie B. Henderson, David R. Conklin . Climate change and land management impact rangeland condition and sage-grouse habitat in southeastern Oregon. AIMS Environmental Science, 2015, 2(2): 203-236. doi: 10.3934/environsci.2015.2.203 |
[3] | Maryem EL FAHEM, Abdellah BENZAOUAK, Habiba ZOUITEN, Amal SERGHINI, Mohamed FEKHAOUI . Hydrogeochemical assessment of mine water discharges from mining activity. Case of the Haut Beht mine (central Morocco). AIMS Environmental Science, 2021, 8(1): 60-85. doi: 10.3934/environsci.2021005 |
[4] | Yenni, Mohd Hafiz Ibrahim, Rosimah Nulit, Siti Zaharah Sakimin . The interactive effects of fertilizer and water stress on plant growth, leaf gas exchange and nutrient uptake on strawberry (Fragaria x ananassa, Duch). AIMS Environmental Science, 2021, 8(6): 597-618. doi: 10.3934/environsci.2021038 |
[5] | Philipp Braun, Timm Faulwasser, Lars Grüne, Christopher M. Kellett, Willi Semmler, Steven R. Weller . On the social cost of carbon and discounting in the DICE model. AIMS Environmental Science, 2024, 11(3): 471-495. doi: 10.3934/environsci.2024024 |
[6] | Dumisani Chirambo . Increasing the value of climate finance in an uncertain environment: Diaspora financial resources as a source of climate finance for Sub-Saharan Africa. AIMS Environmental Science, 2017, 4(6): 730-742. doi: 10.3934/environsci.2017.6.730 |
[7] | Djordje Vilimanovic, Gangadhar Andaluri, Robert Hannah, Rominder Suri, A. Ronald MacGillivray . Occurrence and aquatic toxicity of contaminants of emerging concern (CECs) in tributaries of an urbanized section of the Delaware River Watershed. AIMS Environmental Science, 2020, 7(4): 302-319. doi: 10.3934/environsci.2020019 |
[8] | Mounir OUABA, Mohamed Elmehdi SAIDI . Contribution of morphological study to the understanding of watersheds in arid environment: A case study (Morocco). AIMS Environmental Science, 2023, 10(1): 16-32. doi: 10.3934/environsci.2023002 |
[9] | Jacqueline Jerney, Magdalena Mayr, Michael Schagerl . Biofilm scrubbing for restoration—algae community composition and succession in artificial streams. AIMS Environmental Science, 2016, 3(3): 560-581. doi: 10.3934/environsci.2016.3.560 |
[10] | Benjamin M. Sleeter, Jinxun Liu, Colin Daniel, Leonardo Frid, Zhiliang Zhu . An integrated approach to modeling changes in land use, land cover, and disturbance and their impact on ecosystem carbon dynamics: a case study in the Sierra Nevada Mountains of California. AIMS Environmental Science, 2015, 2(3): 577-606. doi: 10.3934/environsci.2015.3.577 |
The Great Miami (GM) and Little Miami (LM) basins (Figure 1) in southwestern Ohio are among the major river systems that were chosen by the National Water-Quality Assessment (NAWQA) Program to signify the varied geography, water resources, landuse and water use in the U.S. Over the years since the watersheds were included in the NAWQA program in 1991, various anthropogenic activities have amplified in the area. Ohio, being the seventh most populous state in the U.S. [1], has experienced substantial land-use changes through much of the watersheds in recent decades [2]. According to Ohio Environmental Protection Agency (OEPA), these changes in land use is reflected in both point (e.g., municipal wastewater treatment plants) and nonpoint (e.g., runoff from agricultural land use and urban areas) source impacts to the watersheds. Changes in agricultural land use, such as channel modification for flood control and ditching and tiling of farmland [3], have resulted in denudation of riparian vegetation, low instream dissolved oxygen, altered watercourses, high proportion of fine sediment, and have exacerbated nutrient enrichment from cropland runoff [4]. Evidence has shown that watershed urbanization has been increasing for both GM and LM watersheds over the years (Tables 1 and 2) and has not only caused increased volumes of wastewater and pollution [5] but reduced the infiltration capacity [6], and accelerated rates of erosion especially when control measures are inadequate. From 2006 to 2011, the urban area has increased 0.23% and 0.29% for the GM and LM watersheds, respectively (Figure 2). It is worse when the base map is from the land cover in 2001--an increase of 0.49% and 0.94% for the GM and LM watersheds, respectively. This increased impervious surface area typically could result in more surface runoffs. Since water is the carrier of agricultural pollutants, an increase of surface runoffs could mean more variety of nonpoint pollutants including nitrogen (N) and phosphorus (P) nutrients and pesticides go into streams. Although the percentage of total P contributed by agricultural land use is dominant in small streams and lakes [7], urban runoff contribution in GM and LM basins is still high at about 25% of the total detected P concentrations [8]. A study in Maryland involving urban watersheds has linked the intensified concentrations of N and P measured in surface runoff with the increased expanse of impervious surfaces [9]. In fact, watersheds that are highly urbanized exhibited higher proportions of total N and P loadings [10].
Land cover | 2001 | 2006 | 2011 |
Water body | 0.98% | 0.99% | 1.01% |
Urban area | 17.32% | 17.58% | 17.81% |
Forested | 11.55% | 11.59% | 11.54% |
Agricultural (crops, pasture, and hay) | 68.57% | 68.25% | 68.06% |
Other | 1.58% | 1.58% | 1.59% |
Land cover | 2001 | 2006 | 2011 |
Water body | 1.0% | 0.97% | 0.98% |
Urban area | 17.8% | 18.45% | 18.74% |
Forested | 23.7% | 23.64% | 23.49% |
Agricultural (crops, pasture, and hay) | 56.2% | 55.60% | 55.41% |
Other | 1.4% | 1.35% | 1.37% |
Surface runoffs primarily deliver nutrients from terrestrial surroundings to adjacent streams and rivers that could lead to deterioration of water quality and cause harm to aquatic ecosystems [11]. Agriculture runoff is the nation's primary cause of impaired water quality on surveyed surface water systems [12]. In addition, polluted runoff from agricultural system is ranked second in terms of the largest sources of damages towards wetlands. In the GM watershed alone, nutrient loading from agricultural land use runoffs is considered among the highest nutrient flux in the Ohio watersheds [13].
A number of negative human health and environmental impacts due to excessive concentrations of N and P nutrients have already been previously studied. Some of the impacts include: declination of stream quality and impairment of biological communities and aquatic life such eutrophication, fish anomalies, and fish kills [14]; degradation of drinking water quality [15,16]; increased severity of harmful algal blooms (HAB, associated with cyanobacteria or blue-green algae) [17] and macrophyte production [18,19,20]; and decreased dissolved oxygen (DO) concentrations, and increasing fluctuations in diel DO and pH [21]. Algal blooms in the GM river were detected in summers of 1995 [22] and 2011 [23]. These negative impacts are not only felt in river networks inside the GM and LM watersheds but including the larger connecting river systems where these watersheds eventually drain. More information about the N and P nutrients in the GM and LM river watersheds is discussed in a subsequent section of this paper.
The most current version of Ohio's Non-Point Source Management Plan [24] and Water Quality Monitoring and Assessment Report [25] echoed the sources of the recent algal blooms in the Ohio River. Apart from the excessive nutrients coming from Ohio River basins, algal growth has been exacerbated by changing weather patterns, specifically, warmer temperatures and more intense storm events. The OEPA [25] recommended to reduce sources of nutrient pollutants while holistically restoring stream health and improving the waterway's ability to integrate and utilize nutrients. By doing so, streams could build resilience to climate change impacts.
One of the biggest concerns of OEPA listed in the Integrated Report Comments section [25] is the lack of information that address the intricate issues of climate change in their draft report, although the impact of climate change on watersheds is already studied locally and globally [26,27,28,29,30]. A quick literature review would reveal that studies on the effects of climate change on hydrologic processes have not been fully explored in the GM and LM regions [30]. Climate change, as represented by temperature increase and changes in timing and geographical patterns of precipitation, is expected to directly influence the hydrologic cycle, affecting plant-available moisture, production of water discharge, snowpack and snowmelt, streamflow, flood hazard, and water quality [31,32,33,34,35]. Precipitation and temperature changes not only modify the timing of runoff, soil moisture [36,37], and nutrient cycles [38,39], but their magnitude as well. Butterbach-Bahl and Dannenmann [40] showed that N mineralization, nitrification, and denitrification, are directly related to soil temperature and soil moisture – factors that are controlled by climate change.
For the Lake Erie Protection and Restoration Plan [41], climate change has already been included as one of the strategic priorities towards the protection of the watershed. As temperature is expected to continue to rise in the next decades, climate change could play a role in the growth of harmful algal blooms as more intensive rain events could mobilize nutrient runoff. It could alter many environmental conditions, such as habitat changes that marine HABs could invade and occur in freshwater. The six impacts of climate change on the occurrence of HABs include warmer water temperatures, increases in atmospheric carbon dioxide concentration, changes in rainfall patterns and salinity, intensification of coastal upwelling, and sea level rise [17]. In addition, climate change could pose an additional threat to the watersheds as urbanization increases, reducing water availability and degrading water quality [42]. It is, therefore, vital to be able to monitor and study the possible consequences of present and future climate on the hydrologic and water quality in the watersheds for management actions to be effectively implemented.
There is a continuing effort to find optimum and adopt new strategies to address the above stressors related to changes in climate that are affecting the GM and LM watersheds. In line with these efforts, we present in this review paper the current investigation and modeling of the N and P nutrients in the GM and LM watersheds – two important watersheds that, undoubtedly, continue to increase in urbanization size, and increase in wastewater and combined sewer overflows [43]. With the present strains in the watersheds, climate change could pose an additional threat to the water quality. Therefore, we look into the effects of climate changes and how future climate scenarios could impact the efforts in reducing the nutrient pollutants in the watersheds. Reference is made to many pertinent new publications that have become available in recent years as well as to the classic works. Further, this review paper is broken down into three main parts:
(1) an introduction that provides the background of the Great Miami and Little Miami basins: section 2 on study area, section 3 on agricultural land use and tillage system, section 4 on nitrogen and phosphorus nutrient loadings
(2) a discussion on the impacts of climate change: section 5 on specific climate change impacts on biodiversity and watersheds
(3) an overview of past and current methods applied to model N and P nutrient pollutants: section 6 on tools for modeling climate change impacts on nutrient loadings.
The GM and LM watersheds drain about 7087 m2 in southwestern Ohio and southeastern Indiana. Since 1997, both have been part of the 51 major river basins in the nation that are called "study units" for long-term assessment of water-quality conditions under the USGS NAWQA Program. Major rivers are the Great Miami River and Little Miami River in Ohio and the Whitewater River in Indiana. All major streams drain directly south-southwest into the Ohio River. The estimated population of the watersheds was approximately 2.8 million in 1995, mostly concentrated in the largest cities of Cincinnati, Dayton, and Hamilton within the study area [44]. High altitudes of 1550 ft above sea level are near the headwaters of the Mad River, while low altitudes of 450 ft are near the Ohio River at Cincinnati. The study area is temperate continental with mean annual temperature ranges from 49°F to 55°F and average annual precipitation in the study area ranges from 35 inches to 43 inches and increases towards the south; about one-third of the precipitation becomes surface runoff [44]. In terms of average snowfall, it is 20 to 30 inches annually.
The headwaters of the LM watershed (drainage area of 1757 m2) are located in the southeastern portion of Clark County, Ohio. Major rivers and streams of the Little Miami River include the Caesar Creek (242 m2), Todd Fork (261 m2), and East Fork Little Miami River (499 m2) [45] (Figure 3). The headwaters of these three major tributaries are located along the far eastern section of the study area; the convergence of the Little Miami River and the Ohio River is east of Cincinnati. Five dams affect the streamflow. One dam is located at the East Fork Whitewater River, and the other four are on the East Fork Little Miami River and main stem of the Little Miami River [46].
There are about 2360 m of rivers and streams in the GM river system. The headwater is located in Indian Lake in Logan County, Ohio. Draining three large cities—Dayton, Hamilton, and Springfield, Ohio—GM has a total drainage area of 5330 m2. Major tributaries in the UGM include the Stillwater River (676 m2) and the Mad River (657 m2) [45] (Figure 4). Mad River's massive amount of groundwater discharge has met OEPA's criteria for a cold-water habitat [47]. Major tributaries in the LGM include Twin Creek (316 m2) and Four Mile Creek (315 m2). The Great Miami River flows 170 m southwest to its confluence with the Ohio River west of Cincinnati. Streamflow in the Great Miami River Basin is affected by 13 dams [48].
Despite the increase of urbanization in the GM and LM watersheds (Tables 1 and 2), agricultural land use still covers 68.06% for GM and 55.41% for LM in the latest land use map. Corn and soybeans dominate the cropland; grown on approximately 1.77 million acres that surround the heavily urbanized Dayton-Cincinnati corridor in 2017 (Figure 5). Spring and early summer are the seasons for corn and soybeans are planting and fall is harvesting season. For winter wheat crops, they are planted in the fall and harvested in the summer. The usage of fertilizers and chemical pesticides is often associated with agricultural practices and the reduction of surface water quality [47]. Rankin et al. [47] found that surface water quality degradation in the watersheds was caused by poor agricultural practices that resulted in habitat modification such as reduction of riparian buffers and filter strips allowing nutrients from commercial fertilizers to reach streams. Commercial fertilizers N, P2O5 (phosphate), and K2O (potash) are used widely in the GM and LM watersheds of predominantly cropland [42]. These commercial fertilizers from row-crop farming in addition to manure from livestock production are the major sources of nutrients in surface and ground waters [25].
In order to reduce runoff and nutrient exports and control soil loss, the National Research Council [49] suggested the use of conservation tillage (CT) – a planting scheme that guarantees cover crop residue of 30 percent or more. CT systems decrease erosion rates by at least 50 to 60 percent [50]. Broad classes of conservation tillage include: no-till or strip-till (NT), ridge-till (RT), and mulch-till (MT). Complete details of each class is provided by Walters and Jasa [51], which also discussed tillage practices such as reduced-till (RDT) and conventional-till (CVT).
Substantial effects of CT consist of increased soil organic matter content in the upper part of the soil profile to improve soil structural stability, and reduced soil erosion by covering soil with crop residue [52,53]. Tillage system impacts soil characteristics such as pH, N, and P levels and the distribution of nutrients and pesticides [54,55].
Conservation tillage plays a substantial share in minimizing emissions of greenhouse gas (GHG) from agriculture and helps in the endeavors to mitigate against climate change [56]. A study by Mangalassery et al. [57] demonstrated that NT practice has 26–31% lower net global warming potential compared with CVT systems. The reason was that NT reduced soil porosity by 33%, reducing the potential CO2 efflux in the end. Mangalassery et al. [57] concluded that under CT, more respiration of aerobic organisms has caused increased soil porosity, thus improving movement of water and air through the soils with important implications for CO2 emissions.
Many farmers practice CT to limit soil loss and decrease the amount of runoff. In fact, about 40% of all U.S. cropland utilizes CT. In the Midwest, for example, NT soybeans remain to be more popular than NT corn. In 2004, Ohio planted 2.9 million acres of NT soybeans, while only 0.8 million acres of corn were planted using NT practice (Table 3) [58]. The low NT percentage for corn is due to the soil temperature and available soil water that are affected by tillage system. Cox et al. [59] determined how the extent CVT, NT, and RT systems could influence the soil temperature and conditions of soil water, thus manipulating the exchange rates of CO2 and growth of corn. The results showed that with the NT system corn kernel phytomass at physiological maturity has lowered compared to CVT. Further, NT and RT systems decreased the soil water uptake, CO2 exchange rates and growth of corn [59].
Crop | Acres | NT | MT (30% residue) | RDT (15–30% residue) | CVT (0–15% residue) |
Soybean | 4,630,915 | 63.7% | 9.0% | 8.3% | 19.0% |
Corn | 3,527,939 | 23.5% | 9.9% | 13.1% | 53.4% |
Cover crops (CC) (e.g., grasses, legumes, brassicas) are also used for conservation tillage systems as nutrient management tools [60]. Apart from covering the soil to guard against soil erosion, the benefits of cover crops have been well documented in the literature: from building healthier soils, to suppressing weed growth, providing habitat for pollinators, keeping nutrients from leaving the field, reducing the loss of phosphorus with runoff, and slowing down runoff allowing improved moisture infiltration [61,62,63,64]. In long-term no-till systems with cover crops uninterrupted, P is efficiently salvaged by the soil, which could lead to a lesser need for P fertilizer [65]. The number of farmers and crop consultants that acknowledge the benefits of planting cover crops is growing, indicating that it is becoming an important farming strategy. Dabney et al. [66] in a review of winter CC and Singh et al. [66] in a study of rice-wheat rotation highlighted that leguminous crops provide N to succeeding crops. There are also other cover crops, particularly grasses and brassicas, that are known to be better at rummaging remaining N.
In 2014, the Sustainable Agriculture Research and Education (SARE) program and the Conservation Technology Information Center (CTIC) did a national survey about CC use involving 2903 farmers [67]. The data that were collected from almost all states (except Utah, Nevada, Arizona and New Jersey) indicated that 75% of the farmers surveyed grow cover crops. Most of the responses from farmers using CC were from the states of Minnesota, Iowa, Missouri, Illinois, Indiana and Ohio. The area with CC has increased tremendously by an average of about 30% per year, according to the survey [67].
While CC have benefits, they also come with some disadvantages namely: extra costs on purchasing cover crop seeds; more time and labor needed for planting and managing; possible increase of pest populations that could be damaging to succeeding crops; challenging to incorporate with tillage system; and selection of the proper variety of cover crops as they function in diverse purposes [64,66,68]. However, these impediments could be addressed through proper training and education. In the SARE-CTIC [67] report, many farmers get their information from agricultural retailers. These information range from helping farmers in their nutrient management plans to adjust for CC types, assess and recognize soil changes resulting from planting cover crops, and to encourage them to use cover crops. University extension educators also impacts farmers' decision on CC purchases. Farmers who use CC are greatly attracted to academic sources [69]. Moving forward, one of the crucial results of the survey was the path on research priorities that farmers preferred. Nearly 1160 farmers surveyed identified "developing cover crops that fit my cash crop timing" and "developing cover crops that fit the climate in my area" as the two most important research subjects [69]. The rest of the top five research priorities that the farmers rated high in terms of importance, according to the SARE-CTIC 2015 report, were: "developing cover crops that help scavenge nitrogen", "developing cover crops that enhance cash crop disease resistance", and "developing cover crops that fit common soil types."
In small amounts, nitrogen and phosphorus are vital nutrients to vigorous aquatic ecosystem operations. However, N and P could exert negative effects in the environment when they are excessive, causing water degradation and aquatic-ecosystem impairment in the watersheds. Commercial fertilizers and animal manure are the principal sources of nutrient pollution from agriculture. Nutrients that are applied in surplus quantities and beyond the amount needed by crops could run off from fields during storms, resulting in high concentrations of nutrients and pesticides entering into surface waters [8] or be carried to subsurface tile drainage systems and eventually infiltrated to the ground water [70]. N and P are primary nutrients for algal and macrophyte production [22]. When algal growth is excessive, it could reduce the dissolved oxygen content of surface waters through plant respiration. Worse, during hot weather, this situation is augmented as water reduces its capacity to hold dissolved oxygen. One of the effects of low dissolved oxygen content in waters is fish kills, because fish and other aquatic insects need oxygen to survive [25]. A graphic depiction of the harmful effects of increasing amount of nutrients in a coastal ecosystem is shown in Figure 6.
Significant amounts of mobilized agricultural N and P from fertilizers in watersheds are transported to the Ohio River and other coastal marine systems such as the Gulf of Mexico. A recent study by Gorham et al. [71] showed the Ohio River with the highest phycocyanin levels. Phycocyanin is a photosynthetic pigment produced by (and more specific to) cyanobacteria. A study on the nutrient fluxes into the Gulf of Mexico showed the GM watershed to be a major contributor of N and P loadings in the entire Mississippi-Atchafalaya River Watershed [72]. A National Center for Water Quality Research (NCWQR) study [73] found the GM River to have the highest soluble reactive phosphorus concentrations and the highest time weighted average total P concentration amongst 10 streams studied in Ohio. Also, the increased growth of HABs in the Gulf is believed to have been caused by transport of N and P nutrients during spring runoff [74]. The USGS SPARROW model on nutrient flux [75] calculated the N and P contributions of various Ohio watersheds to the Gulf of Mexico. Ohio's contribution to the total fluxes of total N and total P to the Gulf is 5.4% and 4.1%, respectively. Table 4 provides a summary of the watersheds within or partially within Ohio that contribute the highest nutrient flux. UG and LM all have high N fluxes, while the LG has high N and P fluxes. Battaglin and Goolsby [76] estimated the N and P fertilizer-application rates from county-level data in the GM and LM watersheds (Figure 7). The rates of fertilizer application for N were highest in these five counties: Mercer, Randolph, Fayette, Union, and Darke. While for P, the top five counties were Randolph, Union, Mercer, Fayette, and Wayne.
High nutrient flux | Watershed (HUC 8#) | N Flux (kg/km2) | P Flux (kg/km2) |
N&P | Upper Wabash (05120101)* | 2159 | 254 |
N | Upper Great Miami (05080001)* | 1790 | 50 |
N | Upper Scioto (05060001)* | 1786 | 88 |
N&P | Lower Great Miami (05080002)* | 1735 | 127 |
N | Little Miami (05090202) | 1653 | 99 |
N | Paint (05060003) | 1405 | 65 |
N&P | Mill Creek (05090203) | 1345 | 174 |
P | Ohio Brush-Whiteoak (05090201) | 1218 | 126 |
The quality of water in the watersheds' river systems has been improving in the last two decades [78]. When the Clean Water Act (CWA), also known as Federal Water Pollution Control Act Amendments, was passed in 1972, substantial improvements have been made to clean up the nation's surface waters from contaminants, especially those that discharged directly into streams by point sources. With the shutdown of point-source dischargers, substantial reductions in contaminant loadings were achieved. Lake Erie was one of the leading recipients of the CWA in Ohio, when it reduced the amount of runoff pollution into the lake, specifically P, thereby improving sewage treatments around the lake [79]. The GM and LM watersheds have also benefited by the CWA. In fact, there have been significant progress in the efforts to improve water quality in the watersheds through enhancing the treatment of wastewaters. Furthermore, by implementing the CWA, other scopes of surface water quality services have expanded such as community-based and voluntary pollution control projects. The collection of scientific data such as the location of pollution sources increased. With the CWA, it becomes easy to monitor agricultural management practices, ensuring that the desired outcomes are met.
The improved biological performance of the GM and LM river systems in the last decade or so could be a cause for optimism. However, publicly-available OEPA reports (1995, 1997, 2001, 2011, 2012, 2013) indicated that surplus of N and P nutrients in the GM watershed still occur. In fact, elevated concentrations of nutrients detected in streams were occasionally at levels that surpassed the recommended statewide nutrient target concentration standards. Rowe et al. [8] found that the N and P measurements have decreased by 50% and 40% respectively since 1974 in the NAWQA study area, yet, still continue to be among the highest concentrations detected in the nation. The phosphorus concentration remains higher than the EPA guideline of 0.1 mg/l, at 0.3 mg/l. In a recent biological and water quality study, nutrient surplus is listed as one of the most persistent sources of impairment in the UGM watershed system [4]. There are really no state-wide standards for in-stream nutrient concentrations in Ohio. However, the Administrative Code 3745-1-04 Part E sets the level of P needed to prevent algal blooms and weeds. The Nutrient Reduction Strategy report [17] lists the recommended statewide nutrient target concentrations (Table 5).
Stream category | Drainage area (m2) | EWH TP (mg/L) | WWH TP (mg/L) | EWH Nitrate + Nitrite (mg/L) | WWH Nitrate + Nitrite (mg/L) |
Headwater | < 20 | 0.05 | 0.08 | 0.50 | 1.0 |
Wadable | 20 to < 200 | 0.05 | 0.10 | 0.50 | 1.0 |
Small river | 200 to < 1000 | 0.10 | 0.17 | 1.0 | 1.5 |
Large river | > 1000 | 0.15 | 0.30 | 1.5 | 2.0 |
The current edition of the nutrient balance report [10] computed the total loadings of N and P on a water-year basis for 2013 and 2014 – the only years with the most recent and complete data sets that included daily water quality monitoring by NCWQR at Heidelberg University in Ohio and the U.S. Geological Survey (USGS). The GM watershed was one of the seven major watersheds included for the biannual analysis of loading sources because it is a major contributor of nutrient load to the Ohio River systems. The total N loadings (by water year) in metric tonnes per annum (mta), were 18,638 mta and 20,805 mta for 2013 and 2014, respectively. That was equivalent to 12% positive difference. For P, the increase was 42% from 1266 mta in 2013 to 1798 mta in 2014. OEPA [10] noted that the nonpoint source is the largest share of the total P (56%) and total N (80%) loads in the GM watershed. The NCWQR monitors water quality station at a USGS gaging station in Miamisburg, Ohio that serves as a pour point for nutrient mass balance calculations. A pour point is a location on a river or stream where the total load is known.
A 2015 Miami Conservancy District (MCD) annual nutrient loading analysis report also showed slightly above record averages for N and P [80]. We provided supplementary figures (Figures A1 and A2) that showed the yearly trends of nutrient loadings and yields from 2006 to 2016. Also, in an OEPA report that nutrient concentrations in the water column of the GM river and its tributaries frequently indicated enrichment. The analysis indicated the direct relationships between total N and total P concentrations, and river discharge for the GM watershed at the pour point in Miamisburg, Ohio. The report illustrated the trends of how total N and total P concentrations rose at the events of runoff. As the events ended, N and P concentrations decreased back to normal levels. Spring and early summer runoff events in 2015 recorded the highest concentrations of N. For phosphorous, an increasing trend was observed at extended periods of lower flows during the summer and early fall. This increase was likely due to the discharges from wastewater treatment plants [80]. In the 2018 OEPA [25] report that analyzed data collected from 2015 to 2017, it highlighted that nutrient enrichment still remains a major contributing factor in impairment of aquatic life and the recent widespread HABs that have been detected in Lake Erie, the Ohio River and other water bodies in Ohio.
Simulations using climate models provide a quantitative basis for estimating the many phases of future climate change and the impacts of changing climate to nutrient loadings in the watersheds. These climate models or popularly known as General Climate Models (GCMs) evaluate properties of the atmosphere and ocean (e.g., temperature and precipitation) to project a range of different emissions scenarios for the future. These scenarios depend mainly on cumulative emissions of GHG and aerosols from potential resource use patterns, technological innovations, and demographics [81]. The outcomes from climate models based on these emissions scenarios provide a scope of plausible Representative Concentration Pathways (RCP), contingent on the assumptions that are measured by each scenario. The Intergovernmental Panel on Climate Change (IPCC) has the sole obligation for assessing and summarizing information pertinent to climate change and reporting this information to the policy makers and the public. IPCC was jointly established by the World Meteorological Organization (WMO) and the United Nations Environment Program (UNEP) in 1988. The panel has published major assessment reports, the most recent one is in 2014. The fifth IPCC assessment report [82] underlined that the global temperature is projected to rise over the 21st century under all assessed emission scenarios. Changes of projected temperature range from 4.7 °F–8.6 °F (2.6 ℃–4.8 ℃) under the extreme scenario (RCP8.5) to 0.5 °F–1.3 °F (0.3 ℃–1.7 ℃) under the conservative scenario (RCP2.6), for 2081–2100 relative to 1986–2005.
Global effects of climate change could be seen in the drop in snow cover and melting of glaciers that have led to a northward shift in plant and animal distributions, and alterations in algal and fish communities and food webs [83]. As climate change persists, it is expected that heat waves could occur frequently and last much longer in many regions [84,85,86], causing threats to human water security and surface water diversity [87,88]. Changes in atmospheric conditions such as precipitation amount, type, timing and even temperature could intensify the water cycle [89], alter runoff and flood frequency, change the timing of the peaks of streamflow [90,91,92,93,94] and have consequent effects on water quality and stream biomes [95]. These atmospheric changes could occur in tandem with water management and land use changes – further worsening climate-induced river flooding [96]. Flooding could lead to further concerns when stream banks erode and stream channels widen [30,97].
In the plant community, a wealth of literature focused on the observed movement of distributions of plant species as a response to climate change [98]. The latest IPCC report has supported this observation through a statement that many terrestrial species would shift their geographic ranges and change migration patterns due to the current climate change [82]. The impact of this shift to the plant distributions would rest on the ability and mobility of the species to migrate and remain on balance with climate [99] as geographic range contracts or expands [100,101]. However, the shift of distribution pattern is not an immediate alarm in the agricultural systems. The long-term alarm is how crop growers would be able to classify and procure plant species that are adjusted to the changing climates [102,103,104]. Another major alarm in conventional agricultural settings is how the current management of accustomed domesticated plant species could still work when local atmospheric conditions vary too fast [105]. So a question remains of whether populations of wild crop relatives would be invaded by other species that are swift to migrate to track the changing climate. A current study by Duku et al. [106] in a tropical, sub-humid watershed in sub-Saharan Africa found that between 50% and 95% of cultivated areas that currently maintain consecutive cropping would have to return to single cropping by 2090 as climate change impacts agricultural systems, particularly rainfed sequential cropping, rainfed cropland expansion, and irrigation expansion. In the end, if climate change causes significant shifts related to agricultural species, this could result in considerable changes in the quantity of transported N and P nutrients to the river systems. That change could include increase detection of nutrient loadings in surface waters that previously recorded minimal amount of contaminant, as climate continues its role to transport and transform nutrients [107,108,109]. Figure 8 provides an overview of the potential hydrological changes to rivers, streams, and coastal areas as a result of the climate changing, and how they interact with current and future human activities [77]. The dashed lines denote adverse response to the system, specifically nutrient-enhanced productivity.
In Ohio, signs of higher temperature have already been reported, and further increases are projected [110]. The average temperature between 2000 and 2007 in Cleveland was 0.45 ℃ above the 30-year average prior to this period. In Cincinnati, it recorded 25 days each year with a temperature of at least 32.2 ℃ – that was six days more than the historical average before 2000. Easterling [110] and Easterling et al. [111] found that during the 20th century there was a cooling of about 0.6 ℃ in the Ohio watersheds, with warming in the northern Midwest of about 2 ℃ for the same period. Additionally, during the second half of the 20th century, the length of the snow season in the Ohio Valley has been decreased by 16 days. Also, northern Ohio has recorded an increasing trend of precipitation by 10% since 1900, in contrast to the decreasing trend by 10% in the south [112]. Kundzewicz et al. [113] and Milly et al. [114] looked at 24 GCM projections for 2041 to 2060 relative to 1901 to 1970 and showed, based on two-thirds of model agreement, that the Midwest region of the USA would have 10–25% increase in runoff events. A similar result was found by Wu [115] with 20% increase using 14 GCMs. Jha et al. [116], through a simulation, found a 21% increase in future precipitation that could cause a 50% net upsurge in surface water output in the Midwest, and this increasing trend could continue in the future [117]. Milly and Dunne [118], in a study of evaporation and surface cooling that included the Ohio River suggested that internal climate forcing mechanisms were the root of the high amounts of precipitation observed in the region. These observed patterns of increasing temperature and varying amounts of precipitation could have intense hydrological effects in the Midwest region [26].
Figures 9 and 10 show the susceptible hot spots where climate change impacts the GM and LM watersheds in the decades to come. The maps represent the precipitation (Figure 9) and temperature (Figure 10) differences between present (averaged for the years 1970–2000) and each of the three selected GCMs based on downscaled data provided by WorldClim [119].
The GCMs used were Community Climate System Model version 4 (CCSM4; [120]), Model for Interdisciplinary Research on Climate version 5 (MIROC5; [121]), and Hadley Centre Global Environment Model version 2-Earth System (HadGEM2; [122]). These GCMs were examined according to existing published evaluations and performances [108,123,124] across the continental U.S. and regions that intersect the study area. Future climate conditions were downloaded for Representative Concentration Pathways (RCP 8.5) available for all selected GCMs for the time period year 2070—average for 2061 to 2080. RCP 8.5 is the most severe scenario that demands the highest projected increase in multiple greenhouse gas concentrations in the atmosphere [125] and associated increases in global surface temperatures [126].
In the GM and LM watersheds, changes in flood frequency and intensity are projected as heavy precipitation events are likely to increase [80]. All three GCMs projected an increase precipitation amounts from 20 mm to 100 mm, especially in the northern part of the watersheds. This is consistent, for instance, with the upward trend of annual precipitation observed in a 30-year average for the Great Miami watersheds – from 947 mm in 1988 to 1046 mm in 2015 [80]. HadGEM2 projected a drier southern tip (Little Miami) in the late-21st century and mostly wetter north (Upper Great Miami), exaggerating water resources problems in some sections of the study area and alleviating them in others. These changes in intense precipitation caused by global temperature increases have already been evident in literature for the last two decades [127,128,129,130].
All three models projected a warmer temperature all throughout the watersheds, showing temperature increases between 2 ℃ to a high of 6 ℃. More prominent changes are likely to befall in the mid-section as CCSM4 projected it to reach 4 ℃, MIROC5 to 5 ℃, and HadGEM2 to 6 ℃. As temperatures climb, a shift from snow to rain in winter precipitation could alter the timing of streamflow peaks in many parts of the watersheds. Also, a wetter climate that is categorized by stronger storm intensity and longer storm duration, could prompt more flushes of nutrients into freshwater environments [131] as surface runoff increases [80]. In the mean annual runoff analysis at Hamilton gaging station, a significant increase was recorded from 299 mm in 1960 to 390 mm in 2015, that is an increase of 91 mm over 55 years.
The impacts of climate change on the watershed ecosystems and hydrologic processes are complex due to a range of components involved in the system. Watershed or hydrological models integrate these components that represent climate, soil, land use, lakes, rivers and coastal waters to evaluate the impacts of catchment change and the interaction among processes. To date, projecting changes in the hydrological processes and water resources is generally addressed by a method combining hydrological models with GCMs. How this method works is that it utilizes the projections of climate models, downscales the results and use them as reasonable input variables to drive hydrologic models to simulate of system operations. Many of the recent studies related to watershed and climate change showed results of model projections out to year 2100.
Having a good understanding of the important biogeochemical and hydrologic processes under climate change is the key to managing N and P nutrients. A review of literature has indicated that there are a number of watershed hydrological and water quality models that are formulated to simulate nutrient cycles and determine total maximum daily loads caused by anthropogenic activities and current hydrologic conditions. To name a few, they are: Soil and Water Assessment Tool (SWAT: [132,133]); Agricultural Nonpoint Source model (AGNPS: [134]); Dynamic Watershed Simulation Model (DWSM: [135]); and Hydrologic Simulation Program Fortran (HSPF: [136]). These models have the capability to evaluate temporal nutrient fluxes by relying on the spatial variability of input variables [137,138]. Without the inclusion of spatial variability (e.g., land use and climate), confidence in model predictions are observed to significantly reduce [139,140].
The spatial data availability and advances in fast computing and simulations are being credited for the rapid increase of popularity of process-based, distributed, and temporal models [141]. Yet, immense data availability could still become a major concern when the degree of intricacy and flexibility of some models are taken into consideration. These models (e.g., SWAT) require extensive input data and calibration [142]. In addition, there are varying degrees of systematic biases in some of the models when compared to actual climate settings [143]. When these biases are identified, they could define the strengths and weaknesses of the model simulations. It is, therefore, necessary that climate model outputs be validated specifically in the context of their applicability for regional hydrological and ecosystem impact analysis [144].
Research efforts have also been conducted on the application of artificial neural networks (ANNs) as predictors of watershed nutrient loadings. ANN is a highly nonlinear approximator that is used as an alternative to the commonly used physical-based models in estimating N and P loadings [145,146,147]. So far, ANN has been effective in studies that estimated surface water and groundwater level variations [148], examined the correlation between land use/land cover and various water quality variables [149], assessed water quality [150,151], and optimized wastewater treatment processes [152]. Kim et al. [146] compared ANN against SWAT and GWLF and found that ANN was computationally efficient and was capable of accurately mimicking the output estimations of SWAT and GWLF with relatively minor errors. One main identified problem about ANN is how to find the best combination of input variables, since it does not need assumption and detailed understanding of the physical characteristics of input parameters [153]. Other shortcomings of ANN include slow learning speed, convergence to local minimum, overfitting, and components of the model's complex structure [154,155], which could lead to poor model performance and high uncertainty of the model outputs [156].
SWAT remains to be the most popular watershed-scale hydrological model for many water quality studies, locally and globally. SWAT has proven, time and again, that it is a versatile and computationally efficient model to simulate the effects of changes in climate, land-use, and management practices on the transport of sediment and nutrient pollutants, pesticides, and loss of nutrients over time [157,158,159,160,161]. SWAT is part of the software modeling framework of the U.S. EPA BASINS (Better Assessment of Science Integrating Point and Nonpoint Sources). The USDA has used SWAT within the Conservation Effects Assessment Project. SWAT was also used to investigate scenarios of water management in the Hydrologic Modeling of the United States Project (HUMUS) [162]. In terms of its diverse applications, SWAT has been an effective tool in numerous agricultural watershed studies worldwide and in many disciplines and under different climate scenarios [158,160,163,164,165,166,167,168]. As of September 2018 from the SWAT Literature Database, over 3500 peer reviewed publications have used SWAT [169]. About 20 and 16 publications were specifically associated with modeling nitrogen and phosphorous pollutant loss and transport, respectively.
In the SWAT modeling approach, N and P inputs are handled separately, categorized in terms of nutrient forms and nutrient sources [143,185]. Table 6 shows the number of N input requirements for SWAT against two other commonly-used watershed hydrological models [170]. As a comparison, SWAT needs 33 input parameters coming from 11 nutrient sources. GWLF only needs 8 inputs from 7 sources, while HSPF needs 10 inputs from 4 nutrient sources. The main sources of N are mainly soil, runoff, groundwater, sediment, plant uptake, urban source, point source, fertilizer, septic system, surface water bodies, and atmosphere. Table 6 also shows that SWAT requires the highest number of P inputs (total of 18 from 10 sources) compared to the two other watershed hydrological models [170]. GWLF only needs 8 inputs from 7 nutrient sources, while HSPF needs 5 inputs from 4 sources. The main sources of P are mainly soil, runoff, groundwater, sediment, plant uptake, urban source, point source, fertilizer, septic system, and surface water bodies. Further, Table 7 shows the important differences in the number of N and P outputs, respectively, from the three models – SWAT, GWLF, and HSPF. Based on the groupings of N outputs, SWAT has the most comprehensive list with 28 N outputs from 8 transport processes in hydrological systems. HSPF gives 23 N outputs from 6 processes. For the groupings of P outputs, again, SWAT is the all-inclusive of the three models with 19 from 6 transport processes, while HSPF has 12 P outputs related to 6 transport processes.
Nitrogen | SWAT | GWLF | HSPF |
Number of input requirements | 33 | 8 | 10 |
Number of nutrient sources | 11 | 7 | 4 |
Phosphorus | |||
Number of input requirements | 18 | 8 | 5 |
Number of nutrient sources | 10 | 7 | 4 |
Nitrogen | SWAT | GWLF | HSPF |
Number of outputs | 28 | 6 | 23 |
Number of transport processes | 8 | 5 | 6 |
Phosphorus | |||
Number of outputs | 19 | 6 | 12 |
Number of transport processes | 6 | 5 | 6 |
Observed and downscaled climate data of precipitation, maximum and minimum temperature obtained from the climate modeling in each subwatersheds for both the current/past and future time periods are utilized as climate forcing inputs in the SWAT model. These dataset inputs are used to assess the impacts of future climate change on the N and P nutrients in the watershed. Further, these datasets are in addition to SWAT's basic input requirements, such as solar radiation and wind speed [171].
Čerkasova et al. [172] simulated the total N and total P loadings under the scenarios RCP4.5 and RCP8.5 using SWAT in a large-scale catchment situated mostly in Belarus. The study found significant seasonal increase of P load in December to March, and then a reduction in loads for the rest of the studied year. In general, an increase in the N load was observed for scenarios RCP4.5 and RCP8.5, with a more notable change detected in RCP4.5. Čerkasova et al. [172] concluded that if no mitigation is taken, climate change could have a substantial bearing on the crop phenology, practice of fertilization application, and human activity, with upsurge of N and P nutrient loads to the rivers as a consequence. An increase of P and N were also observed by Wagena et al. [35] when projecting to future scenarios 2045 to 2068 for the subwatershed of Mahantango Creek in east-central Pennsylvania. The increase of P loading was found to be related to the increased precipitation intensity and increased in winter/spring sediment-bound P. Similarly, the increase in N export annually was caused by the large surges in nitrification in the winter/spring seasons and increased runoff/soil moisture. Comparable findings were seen by Cho et al. [173] as total P and N loadings were higher during wet season due to increase of precipitation amount. Yang et al. [174] utilized 16 GCMs under RCP4.5 and RCP8.5 to project the discharge, transport, and transformation of N in the Upper Huai River Basin in China by using identified anthropogenic dataset coming from "industries, municipal sewage treatment plants, concentrated and scattered feedlot operations, rural households, and crop production". Yang et al. [174] found that RCP4.5 and RCP8.5 could raise the average and extreme total N loadings in the months of February, May, and November. The effects of climate change on the August total N loads were more uneven. Using five GCMs under RCP8.5, Lee et al. [175] quantified the increase of N and concluded that a temperature increase of 5 ℃ would increase N loads in Chesapeake Bay watershed in Maryland and Virginia by 66%. Greater increase of the rate of N was observed in regions of the watershed that have larger percent of cropland. In a study of N loads in the Upper Mississippi River Basin using 10 GCMs under the old A1B scenario, Jha et al. [176] anticipated N loadings to increase through all Illinois subwatersheds. In contrast, N loadings were anticipated to decline for over 40% of the Iowa sub-basins as a result to the future decrease in runoff [176].
In the case of the LM watershed, Tong et al. [43] modeled the states of future climate according to several scenarios to forecast the potential changes in water flow, total N and P, and sediment loads. The study used the climate change scenarios on the predicted results from IPCC and the UK Hadley Centre's climate models. Five theoretical climate change scenarios were used in the analysis. The reference case scenario was calculated by averaging the daily temperature values and precipitation amounts in the last 50 years (from 1945 to 1995). The scenarios were: "wet" (4℃ increase in temperature, 20% increase in precipitation); "wettest" (2℃ increase in temperature, 20% increase in precipitation); "dry" (2℃ increase in temperature, 20% decrease in precipitation); and "driest" (4℃ increase in temperature, 20% decrease in precipitation). The study found that increases in temperature did not have much effect on the annual runoff compared to the effect caused by changes in precipitation. This trend confirmed the results of Chiew et al. [177] and Gellens and Roulin [178].
While the strength of the model lies in having numerous physically-based operations that control intricate hydrological practices and nutrient processes, it has weaknesses as well. Uncertainties in modeling efforts varied from the parameterization and representation of the natural system to channel scale such as watershed width, depth, and slope [179]. There were also uncertainties identified from inputs in agricultural land management that differed between farmers, and also uncertainties in river flow and water quality monitoring strategies [180]. Moreover, the large number of system parameters required by the model could lead to over-calibration in some cases [140]. To improve modeling results on future climate studies, Glavan et al. [180] suggested integrating improved regional climate models with improved strategies in monitoring for P and N loadings. This is in addition to more examinations about the how, for instance, soil properties and erosive power of rainfall are interconnected.
The importance of this paper lies in the fact that it is one of a very few papers to review the interplay between nutrient pollution and climate change in the GM and LM watersheds. The results from this review have many potential applications. They are useful to environmental scientists and watershed managers to find optimum and adopt new strategies and tools to mitigate the impacts of climate change to the water quality in the watersheds. Attempts to conform with the CWA and manage all sources of pollution in a watershed is progressing as there have been substantial improvements in the water quality in the watersheds through enhancing the treatment of wastewaters. Also, through the CWA, other surface water quality services have expanded such as community-based and voluntary pollution control projects. However, there are still things to be done as publicly-available OEPA reports indicated that surpluses of N and P nutrients in the watershed are still occurring. At some instances, the elevated concentrations of nutrients detected in streams surpassed the recommended statewide nutrient target concentration standards.
It remains a hurdle on how to diminish nutrient pollution in the watersheds from agriculture and wastewater treatment facilities because of two main reasons: (1) large number of wastewater producers and (2) changing nature of the system of nutrient loading. Existing future projections from regional and global studies have shown that N and P contributions to surface waters from agriculture and other sources would continue to rise in the coming decades [31,172,181,182,183,184]. From 1960 to 1995, the use of N and P fertilizer has increased sevenfold and threefold, respectively, with another threefold projected to occur by year 2050 unless something is done to increase the efficiency of fertilizer-use [185]. EPA, through its current report for improving nutrient reduction at wastewater treatment plants [186], stressed that nutrient pollution is the costliest and most challenging environmental problem at present. As nutrient issues continue to persevere, the report proposed further treatment plant modifications to specifically target N and P through carefully designed biological and chemical processes [186].
Specific solutions to the problem of nutrient pollution would rest on the awareness and better understanding of these five factors: (1) nutrient loading processes, (2) nutrient sources, (3) transport pathways of nutrients, (4) nutrient interaction with climate, and (5) nutrient interconnection with various agricultural practices and land uses [17]. Scientists and policy makers have underscored the significance of targeted measures to moderate nutrient losses from agricultural areas to surface waters and to protect these surface waters from excessive nutrient contamination [187,188]. It is crucial to identify areas that contribute disproportionately high nutrient loadings in order to competently control nutrient losses at the watershed scale [189]. Numerous literatures have identified cost-effective approaches to help reduce nutrient pollution. Gitau et al. [190] employed farm-level optimization approach where the authors combined a watershed-level nonpoint-source model and best management practices (BMPs) to create alternative scenarios for the farm before the implementation of BMP. In another approach by Arabi et al. [191], the combination of a watershed model, BMPs, a financial factor, and a spatial search technique has shown to reduce the nutrient pollution load by a factor of 2. These approaches incorporated a vital component into the analysis: farmers' adoption of BMPs. A better understanding of the farmers' conservation operations and what affects them could better guide the formulation and execution of these approaches [192]. Also, a better understanding of the combined effects of changing land cover, climate, and atmospheric deposition is crucial. Model simulation scenarios from Gabriel et. al (2018) have shown that the combination of atmospheric N deposition and precipitation demonstrated the largest impact on N fate and transport in two watersheds in North Carolina, USA [193]. Furthermore, the Nutrient Reduction Strategy report recommended conducting more educational activities that include conferences, meetings, educational materials and newsletters on farm demonstration plots and research activities directed at appropriate nutrient utilization and water quality concerns [41].
Climate change could further complicate the nutrient reduction process in the watersheds, as large uncertainties are introduced into the approximation of future water resources, quality, and runoff risks. This complication is a big wall researchers have to hurdle to obtain information that are reliable and, ultimately, could guide them in the protection of watershed resources. Uncertainties that go along with future climate change prediction still remain high, although recently, there are improvements in the categorization of these uncertainties [82]. Further, the disagreements among current generation of climate models indicate how various models characterize future climate differently. Take for example Figures 9 and 10 for the GM and LM watersheds when a model does not concur on the sign of future changes of precipitation. Hence, the assessment of the influences of climate change on P and N nutrients based on one, two, or three GCMs could yield conflicting results. Thus, there is a need to develop an optimized climatic approach to watershed nutrient management, because if approaches would be based on current and existing studies, it is very hard to evaluate nutrient-related outcomes of emission pathways with high reliability and accuracy. It is essential to align adaptation measures in a holistic manner because the actual effects of climate change, whether in local or regional scale, would be the product of complex interactions of schemes and sectors [194]. The IPCC [82,83] calls it integrated assessment (IA); defined as "an interdisciplinary process that combines, interprets, and communicates knowledge from diverse scientific disciplines from the natural and social sciences to investigate and understand causal relationships within and between complicated systems." The scientific community continues to publish projected climate model simulations that recommend an IA to help reduce P and N loadings. This include improvement of facilities [174], increase implementation of nutrient monitoring, management of winter cover crops, and restoration of wetlands [175], increase management of floodplain landscapes [180], and evaluation of the political, budgetary and technical barriers [195].
As we underscored in the beginning of this review, Ohio EPA has not directed its efforts yet on the complex concerns of climate change in the 2018 Draft Integrated Report [25]. By missing to address the issue, OEPA emphasized in the report that it may be "prohibited from listing waters of the State as impaired without first demonstrating that the failure to meet applicable water quality standards is not due to the existence of naturally occurring conditions in the open waters." Nevertheless, OEPA has acknowledged the effects of climate change in the future of Ohio's open waters, in particular, how climate change could bring intensive rain events that could mobilize nutrient runoff, and the role it plays in the growth of algae blooms. In its latest MCD report [80], OEPA pointed out recent trends in hydrologic data for the GM watershed that indicated a tendency toward wetter than normal conditions, with above normal precipitation had occurred in seven out of the 10 years from 2000–2009. Also, OEPA mentioned that climate variability and changes in water use were contributory to the increasing trends in annual runoff, mean daily flows, and seven-day low flows for the GM watershed. Lastly, as climate change could challenge the management of watershed nutrients in the coming decades, we are optimistic that it would be addressed by environmental agencies in future reports.
Funding: The authors received no specific funding for this work.
The authors declare no conflict of interest.
[1] | Ohio Department of Development (ODD) Population and Housing: Ohio's population; Ohio Development Services Agency, 2010. Available from: https://development.ohio.gov/reports/reports_pop_proj_map.htm. |
[2] |
Tong STY, Sun Y, Ranatunga T, et al. (2012) Predicting plausible impacts of sets of climate and land use change scenarios on water resources. Appl Geogr 32: 477–489. doi: 10.1016/j.apgeog.2011.06.014
![]() |
[3] | Debrewer LM, Rowe GL, Reutter DC, et al. Environmental setting and effects on water quality in the Great and Little Miami River basins, Ohio and Indiana: Water-Resources Investigations Report, 2000. Available from: https://pubs.usgs.gov/wri/1999/4201/report.pdf. |
[4] | OEPA biological and water quality study of the Upper Little Miami River. Clark, Clinton, Greene, Madison, Montgomery, and Warren Counties, Ohio. OHIO EPA Technical Report: Division of Surface Water, 2011. Available from: https://epa.ohio.gov/Portals/35/documents/LMR_Upper_Basin_2011_TSD.pdf. |
[5] | Pitt R, Bozeman M. Water quality and biological effects of urban runoff in Coyote Creek, U.S. Ohio Environmental Protection Agency, 1980. Available from: https://nepis.epa.gov/Exe/ZyPURL.cgi?Dockey=30000C3M.TXT. |
[6] |
Tong STY (1990) The hydrologic effects of urban land use: A case study of the little Miami River Basin. Landsc and Urban Plan 19: 99–105. doi: 10.1016/0169-2046(90)90037-3
![]() |
[7] |
Turner RE, Rabalais NN (2004) Suspended sediment, C, N, P, and Si yields from the Mississippi River Basin. Hydrobiologia 511: 79–89. doi: 10.1023/B:HYDR.0000014031.12067.1a
![]() |
[8] | Rowe GL, Reutter DC, Runkle DL, et al. Water quality in the Great and Little Miami River Basins, Ohio and Indiana, 1999–2001, USGS, 2004. Available from: https://pubs.er.usgs.gov/publication/cir1229. |
[9] |
Kaushal SS, Groffman PM, Band LE, et al. (2008) Interaction between urbanization and climate variability amplifies watershed nitrate export in Maryland. Environ Sci Technol 42: 5872–5878. doi: 10.1021/es800264f
![]() |
[10] | OEPA Nutrient Mass Balance Study for Ohio's Major Rivers, Ohio Environmental Protection Agency, Division of Surface Water Modeling, Assessment and TMDL Section, 2016. Available from: https://epa.ohio.gov/Portals/35/documents/Nutrient%20Mass%20Balance%20Study%202018_F inal.pdf. |
[11] |
Yu S, Yu GB, Liu Y, et al. (2012) Urbanization impairs surface water quality: Eutrophication and metal stress in the Grand Canal of China. River Res Appl 28: 1135–1148. doi: 10.1002/rra.1501
![]() |
[12] | EPA National Nonpoint Source Program-a catalyst for water quality improvements, 2017. Available from: https://www.epa.gov/sites/production/files/2016-10/documents/nps_program_highlights_report-508.pdf. |
[13] | MCD Lower Great Miami River Nutrient Management Project, 2017. Available from: https://www.mcdwater.org/wp-content/uploads/2017/06/2017-LGMR_Report-FINAL_02-28-17_Compressed_Graphics.pdf. |
[14] |
Naramngam S, Tong STY (2013) Environmental and economic implications of various conservative agricultural practices in the Upper Little Miami River basin. Agric Water Manag 119: 65–79. doi: 10.1016/j.agwat.2012.12.008
![]() |
[15] |
Paul MJ, Meyer JL (2001) Streams in the urban landscape. Annu Rev Ecol Syst 32: 333–365. doi: 10.1146/annurev.ecolsys.32.081501.114040
![]() |
[16] | Center for Watershed Protection, Impacts of impervious cover on aquatic ecosystems, Watershed Protection Research Monograph No. 1. Center for Watershed Protection, 2003. Available from: https://clear.uconn.edu/projects/TMDL/library/papers/Schueler_2003.pdf. |
[17] | OEPA Ohio Nutrient Reduction Strategy, Ohio Environmental Protection Agency, 2013. Available from: https://epa.ohio.gov/Portals/35/wqs/ONRS_final_jun13.pdf. |
[18] |
Townsend AR, Howarth RW, Bazzaz FA, et al. (2003) Human health effects of a changing global nitrogen cycle. Front Ecol Environ 1: 240–246. doi: 10.1890/1540-9295(2003)001[0240:HHEOAC]2.0.CO;2
![]() |
[19] | Newcomber G, House J, Ho L, et al. Management strategies for cyanobacteria (blue-green algae) and their toxins: A guide for water utilities. Water Quality Research Australia Limited, 2009. Available from: https://www.researchgate.net/profile/Lionel_Ho/publication/242740698_Management_Strategie s_for_Cyanobacteria_Blue-Green_Algae_A_Guide_for_Water_Utilities/links/02e7e52d62273e8f70000000/Management-Strategies-for-Cyanobacteria-Blue-Green-Algae-A-Guide-for-Water-Utilities.pdf. |
[20] |
Graham J, Loftin K, Meyer M, et al. (2010) Cyanotoxin mixtures and taste-and-odor compounds in cyanobacterial blooms from the Midwestern United States. Environ Sc. Technol 44: 7361–7368. doi: 10.1021/es1008938
![]() |
[21] | MCD nitrogen and phosphorus concentrations and loads in the Great Miami River Watershed, Ohio 2005 – 2011, 2012. Available from: https://www.mcdwater.org/wp-content/uploads/PDFs/2012NutrientMonitoringReport_Final.pdf. |
[22] | OEPA biological and water quality study of the Middle and Lower Great Miami River and selected tributaries, 1995-Volume I, Ohio Environmental Protection Agency, 1997. Available from: https://epa.ohio.gov/portals/35/documents/lmgmr95.pdf. |
[23] | Smith JH. Miami River algae draws local attention, 2011. Available from: https://www.daytondailynews.com/news/local/miami-river-algae-draws-local- attention/Rh715SpRPOMoJsF6qMyghK. |
[24] | NPS Nonpoint Source Management Plan Update, Ohio Environmental Protection Agency, 2018. Available from: https://www.epa.ohio.gov/portals/35/nps/nps_mgmt_plan.pdf. |
[25] | OEPA Integrated Water Quality Monitoring and Assessment Report, Ohio Environmental Protection Agency, 2018. Available from: https://www.epa.ohio.gov/Portals/35/tmdl/2018intreport/2018IR_Final.pdf. |
[26] | Takle ES, Xia M, Guan F, et al. (2006) Upper Mississippi River basin modeling system part 4: climate change impacts on flow and water quality, In: Coastal Hydrology and Processes, Water Resources Publications, 135–142. |
[27] | Luo Y, Ficklin DL, Liu X, et al. (2013) Assessment of climate change impacts on hydrology and water quality with a watershed modeling approach. Sci Total Environ 450–451: 72–82. |
[28] |
Ekness P, Randhir TO (2015) Effect of climate and land cover changes on watershed runoff: A multivariate assessment for storm water management. J Geophys Res-Geosciences 120: 1785–1796. doi: 10.1002/2015JG002981
![]() |
[29] |
Rahman K, da Silva AG, Tejeda EM (2015) An independent and combined effect analysis of land use and climate change in the upper Rhone River watershed, Switzerland. Appl Geogr 63: 264–272. doi: 10.1016/j.apgeog.2015.06.021
![]() |
[30] |
Li Z, Fang H (2017) Modeling the impact of climate change on watershed discharge and sediment yield in the black soil region, northeastern China. Geomorphology 293: 255–271. doi: 10.1016/j.geomorph.2017.06.005
![]() |
[31] |
Barnett TP, Adam JC, Lettenmaier DP (2005) Potential impacts of a warming climate on water availability in snow-dominated regions. Nature 438: 303–309. doi: 10.1038/nature04141
![]() |
[32] |
Wang GQ, Zhang JY, Xuan YQ (2013) Simulating the Impact of Climate Change on Runoff in a Typical River Catchment of the Loess Plateau, China. J Hydrometeor 14: 1553–1561. doi: 10.1175/JHM-D-12-081.1
![]() |
[33] |
Barranco LM, Álvarez-Rodríguez J, Olivera F (2014) Assessment of the expected runoff change in Spain using climate simulations. J Hydrol Eng 19: 1481–1490. doi: 10.1061/(ASCE)HE.1943-5584.0000920
![]() |
[34] |
Demaria EMC, Roundy JK, Wi S (2016) The effects of climate change on seasonal snowpack and the hydrology of the Northeastern and Upper Midwest United States. J Climate 29: 6527–6541. doi: 10.1175/JCLI-D-15-0632.1
![]() |
[35] | Wagena MB, Collick AS, Ross AC (2018) Impact of climate change and climate anomalies on hydrologic and biogeochemical processes in an agricultural catchment of the Chesapeake Bay watershed, USA. Sci Total Environ 637–638: 1443–1454. |
[36] |
Gleick PH (1989) Climate change, hydrology, and water resources. Rev Geophys 27: 329–344. doi: 10.1029/RG027i003p00329
![]() |
[37] |
Dyer F, ElSawah S, Croke B, et al. (2014) The effects of climate change on ecologically-relevant flow regime and water quality attributes. Stoch Environ Res Risk Assess 28: 67–82. doi: 10.1007/s00477-013-0744-8
![]() |
[38] |
Ferrer J, Pérez-Martín MA, Jiménez S, et al. (2012) GIS-based models for water quantity and quality assessment in the Júcar River Basin, Spain, including climate change effects. Sci Total Environ 440: 42–59. doi: 10.1016/j.scitotenv.2012.08.032
![]() |
[39] | Van Liew M, Feng S, Pathak T (2013) Assessing climate change impacts on water balance, runoff, and water quality at the field scale for four locations in the heartland. Transactions of the ASABE 56: 833–900. |
[40] |
Butterbach-Bahl K, Dannenmann M (2011) Denitrification and associated soil N2O emissions due to agricultural activities in a changing climate. Curr Opin Env Sust 3: 389–395. doi: 10.1016/j.cosust.2011.08.004
![]() |
[41] | OEPA Ohio Nutrient Reduction Strategy, Ohio Environmental Protection Agency, 2016. Available from: https://epa.ohio.gov/Portals/35/wqs/ONRS_addendum.pdf. |
[42] | OEPA Ohio water resource inventory, executive summary: Summary, conclusions, and recommendations Division of Surface Water and Monitoring Assessment Section, Ohio Environmental Protection Agency, 1996. Available from: https://www.epa.ohio.gov/portals/35/documents/exsumm96.pdf. |
[43] |
Tong STY, Liu AJ, Goodrich JA (2007) Climate change impacts on nutrient and sediment loads in a Midwestern agricultural watershed. J Environ Inform 9: 18–28. doi: 10.3808/jei.200700084
![]() |
[44] | Rowe GL, Baker NT. National Water-Quality Assessment Program, Great and Little Miami River Basins, 1997. Available from: https://pubs.water.usgs.gov/circ1229. |
[45] | Cross WP, Drainage areas of Ohio streams, supplement to Gazetteer of Ohio streams; Ohio Water Plan Inventory Report: Ohio Department of Natural Resources, 1967, 61. |
[46] | OEPA East Fork Little Miami River comprehensive water quality report, Little Miami River Basin, Clinton, Highland, Brown, and Clermont Counties: Ohio Environmental Protection Agency, 1985. Available from: http://msdgc.org/downloads/initiatives/water_quality/2012_lmr_biological_water_quality_study. pdf. |
[47] | Rankin ET, Yoder CO, Mishne D. Ohio water resources inventory executive summary-Summary, conclusions and recommendation: Ohio Environmental Protection Agency, 1996, 75 p. Available from: https://www.epa.ohio.gov/portals/35/documents/exsumm96.pdf. |
[48] | Miami Conservancy District Flood protection dams: Miami Conservancy District, 2018. Available from: http://www.conservancy.com/dams.asp#dams. |
[49] | National Research Council Alternative agriculture, National Academy Press, 1989. Available from: https://www.nap.edu/catalog/1208/alternative-agriculture. |
[50] |
Dickey E, Shelton DP, Jasa P, et al. (1985) Soil erosion from tillage systems used in soybean and corn residues. T ASAE 28: 1124–1130. doi: 10.13031/2013.32399
![]() |
[51] | Walters D, Jasa P. Conservation tillage in the United States: An overview, 2018. Available from: http://agecon.okstate.edu/isct/labranza/walters/conservation.doc. |
[52] |
Tebrügge F, Düring R-A (1999) Reducing tillage intensity - a review of results from a long-term study in Germany. Soil Tillage Res 53: 15–28. doi: 10.1016/S0167-1987(99)00073-2
![]() |
[53] |
Armand R, Bockstaller C, Auzet A-V, et al. (2009) Runoff generation related to intra-field soil surface characteristics variability: Application to conservation tillage context. Soil Tillage Res 102: 27–37. doi: 10.1016/j.still.2008.07.009
![]() |
[54] |
Borie F, Rubio R, Ruanet JL, et al. (2006) Effects of tillage systems on soil characteristics, glomalin and mycorrhizal propagules in a Chilean Ultisol. Soil Tillage Res 88: 253–261. doi: 10.1016/j.still.2005.06.004
![]() |
[55] |
Rocha EO, Calijuri ML, Santiago AF, et al. (2012) The contribution of conservation practices in reducing runoff, soil loss, and transport of nutrients at the watershed level. Water Resour Manage 26: 3831–3852. doi: 10.1007/s11269-012-0106-1
![]() |
[56] |
Jarecki MK, Lal R (2003) Crop management for soil carbon sequestration. Crit Rev Plant Sci 22: 471–502. doi: 10.1080/713608318
![]() |
[57] | Mangalassery S, Sjögersten S, Sparkes DL, et al. (2014) To what extent can zero tillage lead to a reduction in greenhouse gas emissions from temperate soils? Sci Rep 4: 4586. |
[58] | Baker NT. Tillage practices in the conterminous United States, 1989–2004-Datasets aggregated by watershed, USGS, 2011, 22 p. Available from: https://pubs.usgs.gov/ds/ds573/pdf/dataseries573final.pdf. |
[59] |
Cox WJ, Zobel RW, van Es HM, et al. (1990) Growth development and yield of maize under three tillage systems in the northeastern U.S.A. Soil Tillage Res 18: 295–310. doi: 10.1016/0167-1987(90)90067-N
![]() |
[60] |
Ruffo ML, Bollero GA (2003) Modeling rye and hairy vetch residue decomposition as a function of degree-days and decomposition-days. Agron J 95: 900–907. doi: 10.2134/agronj2003.9000
![]() |
[61] |
Findlater PA, Carter DJ (1990) A model to predict the effects of prostrate ground cover on wind erosion. Aust J Soil Res 28: 609–622. doi: 10.1071/SR9900609
![]() |
[62] | Staver KW, Brinsfield RB (1998) Using cereal grain winter cover crops to reduce groundwater nitrate contamination in the mid-Atlantic coastal plain. J Soil Water Conserv 53: 230–240. |
[63] |
Whish JPM, Price L, Castor PA (2009) Do spring cover crops rob water and so reduce wheat yields in the northern grain zone of eastern Australia? Crop Pasture Sci 60: 517–525. doi: 10.1071/CP08397
![]() |
[64] | Fageria NK, Baligar VC, Bailey BA (2018) Role of cover crops in improving soil and row crop productivity. Commun Soil Sci Plant Anal 36: 19–20. |
[65] | Hoorman JJ. Using cover crops to convert to no-till, 2018. Available from: https://ohioline.osu.edu/factsheet/SAG-11. |
[66] |
Dabney SM, Delgado JA, Reeves DW (2001) Using winter cover crops to improve soil and water quality. Commun Soil Sci Plant Anal 32: 1221–1250. doi: 10.1081/CSS-100104110
![]() |
[67] | SARE- CTIC Report of the 2013–2014 Cover Crop Survey. Joint publication of the Conservation Technology Information Center and the North Central Region Sustainable Agriculture Research and Education Program.; Conservation Technology Information Center: West Lafayette, Indiana, 2014. Available from: https://www.sare.org/Learning-Center/From-the-Field/North-Central-SARE-From-the-Field/2013-14-Cover-Crops-Survey-Analysis. |
[68] |
Creamer NG, Baldwin KR (2000) An evaluation of summer cover crops for use in vegetable production systems in North Carolina. HortScience 35: 600–603. doi: 10.21273/HORTSCI.35.4.600
![]() |
[69] | SARE- CTIC Cover Crop survey report. Conservation Technology Information Center. 2014. Report of the 2013-14 Cover Crop Survey. Joint publication of the Conservation Technology Information Center and the North Central Region Sustainable Agriculture Research and Education Program; Conservation Technology Information Center: West Lafayette, Indiana, 2015. Available from: https://www.sare.org/Learning-Center/From-the-Field/North-Central-SARE-From-the-Field/2016-Cover-Crop-Survey-Analysis. |
[70] | Nokes S, Ward A. Surface water quality best management practices summary guide: Columbus, The Ohio State University Extension Factsheet, 1997. Available from: http://epa.ohio.gov/dsw/storm/technical_guidance. |
[71] |
Gorham T, Jia Y, Shum CK, et al. (2017) Ten-year survey of cyanobacterial blooms in Ohio's waterbodies using satellite remote sensing. Harmful Algae 66: 13–19. doi: 10.1016/j.hal.2017.04.013
![]() |
[72] | Goolsby DA, Battaglin WA, Lawrence GB, et al. Flux and sources of nutrients in the Mississippi-Atchafalaya River Basin: Topic 3 Report for the Integrated Assessment on Hypoxia in the Gulf of Mexico, 1999. Available from: http://www.cop.noaa.gov/pubs/das/das17.pdf. |
[73] | Baker DB, Richards RP, Kramer JW. Point source-nonpoint source trading: applicability to stream TMDLs in Ohio. In Proceedings of the Proceedings – Innovations in Reducing Nonpoint Source Pollution, 2006, 328–337. |
[74] |
Scavia D, Justic D, Bierman VJ (2004) Reducing hypoxia in the Gulf of Mexico: Advice from three models. Estuaries 27: 419–425. doi: 10.1007/BF02803534
![]() |
[75] |
Smith RA, Schwarz GE, Alexander RB (1997) Regional interpretation of water-quality monitoring data. Water Resour Res 33: 2781–2798. doi: 10.1029/97WR02171
![]() |
[76] | Battaglin WA, Goolsby DA. Spatial data in geographic information system format on agricultural chemical use, land use, and cropping practices in the United States, 1995. Available from: https://pubs.usgs.gov/wri/1994/4176/report.pdf. |
[77] |
Rabalais NN, Turner RE, Díaz RJ, et al. (2009) Global change and eutrophication of coastal waters. ICES J Mar Sci 66: 1528–1537. doi: 10.1093/icesjms/fsp047
![]() |
[78] | Midwest Biodiversity Institute Biological and water quality assessment of the Great Miami River and tributaries 2013 Hamilton County, Ohio, 2014, 121. Available from: https://midwestbiodiversityinst.org/reports/biological-and-water-quality-study-of-the-little-miami-river-and-tributaries-2012-hamilton-county-ohio/LMR%202012%20MSDGC%20FINAL%20REPORT%2020130930.pdf. |
[79] | OEPA Integrated Water Quality Monitoring and Assessment Report: Ohio Environmental Protection Agency, 2002. Available from: https://www.epa.ohio.gov/portals/35/tmdl/2002IntReport/Ohio2002IntegratedReport_100102.pdf. |
[80] | MCD Water Data Report, Great Miami River Watershed, Ohio, 2015. Available from: https://www.mcdwater.org/wp-content/uploads/PDFs/2015-Water-Data-Report-FINAL-reduced.pdf. |
[81] | Hayhoe K, Edmonds J, Kopp RE, et al. (2017) Climate models, scenarios, and projections: Climate Science Special Report: Fourth National Climate Assessment; U.S. Global Change Research Program, 133–160. |
[82] | IPCC Climate Change 2014: Synthesis Report. Contribution of Working Groups I, II and III to the Fifth Assessment Report of the Intergovernmental Panel on Climate Change: Geneva, Switzerland,. Available from: https://www.ipcc.ch/report/ar5/syr. |
[83] | IPCC Contribution of Working Groups I, II and III to the Fourth Assessment Report of the Intergovernmental Panel on Climate Change Core Writing Team; Geneva, Switzerland, 2007. Available from: https://www.ipcc.ch/report/ar4/syr. |
[84] |
Meehl GA, Tebaldi C (2004) More intense, more frequent, and longer lasting heat waves in the 21st century. Science 305: 994–997. doi: 10.1126/science.1098704
![]() |
[85] |
Rosenzweig C, Major DC, Demong K, et al. (2007) Managing climate change risks in New York City's water system: assessment and adaptation planning. Mitig Adapt Strat Glob Change 12: 1391–1409. doi: 10.1007/s11027-006-9070-5
![]() |
[86] | Karl TR, Melillo JM, Peterson TC. Global climate change impacts in the United States, Cambridge University Press: UK, 2009. Available from: http://www.nrc.gov/docs/ML1006/ML100601201.pdf. |
[87] | Lawrimore JH, Menne MJ, Gleason BE, et al. (2011) An overview of the Global Historical Climatology Network monthly mean temperature data set, version 3. JGR Atmospheres 2011, 116. |
[88] |
Vörösmarty CJ, McIntyre PB, Gessner MO, et al. (2010) Global threats to human water security and river biodiversity. Nature 467: 555–561. doi: 10.1038/nature09440
![]() |
[89] | Lettenmaier DP, Milly PCD (2009) Land waters and sea level. Nature Geoscience 2009, 2, 3. |
[90] | Hatfield JL, Prueger JH (2004) Impacts of changing precipitation patterns on water quality. J Soil Water Conserv 59: 51–58. |
[91] |
Prowse TD, Beltaos S, Gardner JT, et al. (2006) Climate change, flow regulation and land-use effects on the hydrology of the Peace-Athabasca-Slave System; Findings from the Northern Rivers Ecosystem Initiative. Environ Monit Assess 113: 167–197. doi: 10.1007/s10661-005-9080-x
![]() |
[92] |
Zhang A, Zhang C, Fu G, et al. (2012) Assessments of impacts of climate change and human activities on runoff with SWAT for the Huifa River Basin, Northeast China. Water Resour Manage 26: 2199–2217. doi: 10.1007/s11269-012-0010-8
![]() |
[93] |
Ye L, Grimm NB (2013) Modelling potential impacts of climate change on water and nitrate export from a mid-sized, semiarid watershed in the US Southwest. Climatic Change 120: 419–431. doi: 10.1007/s10584-013-0827-z
![]() |
[94] |
Zahabiyoun B, Goodarzi MR, Bavani ARM, et al. (2013) Assessment of climate change impact on the Gharesou River Basin using SWAT hydrological model. CLEAN – Soil, Air, Water 41: 601–609. doi: 10.1002/clen.201100652
![]() |
[95] | Backlund,P, Janetos A Schimel D. The effects of climate change on agriculture, land resources, water resources, and biodiversity in the United States. Synthesis and Assessment Product 4.3. Washington, DC: U.S. Environmental Protection Agency, Climate Change Science Program, 2008, 240. |
[96] |
Peterson TC, Heim RR, Hirsch R, et al. (2013) Monitoring and understanding changes in heat waves, cold waves, floods, and droughts in the United States: State of knowledge. Bull Amer Meteor Soc 94: 821–834. doi: 10.1175/BAMS-D-12-00066.1
![]() |
[97] |
Dudula J, Randhir TO (2016) Modeling the influence of climate change on watershed systems: Adaptation through targeted practices. J Hydrol 541: 703–713. doi: 10.1016/j.jhydrol.2016.07.020
![]() |
[98] |
Davis MB, Shaw RG (2001) Range shifts and adaptive responses to quaternary climate change. Science 292: 673–679. doi: 10.1126/science.292.5517.673
![]() |
[99] |
Feeley KJ, Silman MR (2010) Land-use and climate change effects on population size and extinction risk of Andean plants. Glob Chang Biol 16: 3215–3222. doi: 10.1111/j.1365-2486.2010.02197.x
![]() |
[100] |
Parmesan C (2006) Ecological and evolutionary responses to recent climate change. Annu Rev Ecol Evol Syst 37: 637–669. doi: 10.1146/annurev.ecolsys.37.091305.110100
![]() |
[101] |
Poloczanska ES, Brown CJ, Sydeman WJ, et al. (2013) Global imprint of climate change on marine life. Nature Clim Chang 3: 919–925. doi: 10.1038/nclimate1958
![]() |
[102] |
Runion GB (2003) Climate change and plant pathosystems: Future disease prevention starts here. New Phytol 159: 531–533. doi: 10.1046/j.1469-8137.2003.00868.x
![]() |
[103] |
Smith DL, Almaraz JJ (2004) Climate change and crop production: contributions, impacts, and adaptations. Can J Plant Pathol 26: 253–266. doi: 10.1080/07060660409507142
![]() |
[104] |
Ashmore M, Toet S, Emberson L (2006) Ozone – a significant threat to future world food production? New Phytol 170: 201–204. doi: 10.1111/j.1469-8137.2006.01709.x
![]() |
[105] |
Alexander JM, Diez JM, Levine JM (2015) Novel competitors shape species' responses to climate change. Nature 525: 515–518. doi: 10.1038/nature14952
![]() |
[106] | Duku C, Zwart SJ, Hein L (2018) Impacts of climate change on cropping patterns in a tropical, sub-humid watershed. PLOS ONE 13, e0192642. |
[107] |
Aguilera R, Marcé R, Sabater S (2015) Detection and attribution of global change effects on river nutrient dynamics in a large Mediterranean basin. Biogeosciences 12: 4085–4098. doi: 10.5194/bg-12-4085-2015
![]() |
[108] |
Cousino LK, Becker RH, Zmijewski KA (2015) Modeling the effects of climate change on water, sediment, and nutrient yields from the Maumee River watershed. J Hydrol Reg Stud 4: 762–775. doi: 10.1016/j.ejrh.2015.06.017
![]() |
[109] | Cordovil C, Cruz S, Brito A, et al. (2018) A simplified nitrogen assessment in Tagus River Basin: A management focused review. Water 10, 406. |
[110] |
Easterling DR (2002) Recent changes in frost days and the frost-free season in the united states. Bull Amer Meteor Soc 83: 1327–1332. doi: 10.1175/1520-0477-83.9.1327
![]() |
[111] |
Easterling DR, Evans JL, Groisman PY, et al. (2000) Observed variability and trends in extreme climate events: A brief review. Bull Amer Meteor Soc 81: 417–426. doi: 10.1175/1520-0477(2000)081<0417:OVATIE>2.3.CO;2
![]() |
[112] | Williamson S, Lakhey S, Karetnikov D, et al. Economic impacts of climate change on Ohio; Center for Integrative Environmental Research, University of Maryland: College Park, Maryland, USA, 2008, 20. |
[113] |
Kundzewicz ZW, Mata LJ, Arnell NW, et al. (2008) The implications of projected climate change for freshwater resources and their management. Hydrolog Sci J 53: 3–10. doi: 10.1623/hysj.53.1.3
![]() |
[114] |
Milly PCD, Dunne KA, Vecchia AV (2005) Global pattern of trends in streamflow and water availability in a changing climate. Nature 438: 347–350. doi: 10.1038/nature04312
![]() |
[115] |
Wu S-Y (2010) Potential impact of climate change on flooding in the Upper Great Miami River Watershed, Ohio, USA: a simulation-based approach. Hydrolog Sci J 55: 1251–1263. doi: 10.1080/02626667.2010.529814
![]() |
[116] | Jha M, Pan Z, Takle ES, et al. (2004) Impacts of climate change on streamflow in the Upper Mississippi River Basin: A regional climate model perspective. JGR Atmospheres 2004, 109. |
[117] |
Pathak P, Kalra A, Ahmad S (2017) Temperature and precipitation changes in the Midwestern United States: implications for water management. Int J Water Resour Dev 33: 1003–1019. doi: 10.1080/07900627.2016.1238343
![]() |
[118] |
Milly PCD, Dunne KA (2001) Trends in evaporation and surface cooling in the Mississippi River Basin. Geophys Res Lett 28: 1219–1222. doi: 10.1029/2000GL012321
![]() |
[119] | WorldClim - Global Climate Data, Free climate data for ecological modeling and GIS. Available from: http://www.worldclim.org. |
[120] |
Gent PR, Danabasoglu G, Donner LJ, et al. (2011) The Community Climate System Model Version 4. J Climate 24: 4973–4991. doi: 10.1175/2011JCLI4083.1
![]() |
[121] |
Watanabe M, Suzuki T, O'ishi R, et al. (2010) Improved climate simulation by MIROC5: Mean States, Variability, and Climate Sensitivity. J Climate 23: 6312–6335. doi: 10.1175/2010JCLI3679.1
![]() |
[122] |
Collins WJ, Bellouin N, Doutriaux-Boucher M, et al. (2011) Development and evaluation of an Earth-System model – HadGEM2. Geosci Model Dev 4: 1051–1075. doi: 10.5194/gmd-4-1051-2011
![]() |
[123] | Taylor KE, Stouffer RJ, Meehl GA (2011) An overview of CMIP5 and the experiment design. Bull Amer Meteor Soc 93: 485–498. |
[124] | Sheffield J, Barrett AP, Colle B, et al. (2013) North American climate in CMIP5 experiments. Part I: Evaluation of historical simulations of continental and regional climatology. J Climate 26: 9209–9245. |
[125] |
van Vuuren DP, Edmonds J, Kainuma M, et al. (2011) The representative concentration pathways: an overview. Climatic Change 109: 5. doi: 10.1007/s10584-011-0148-z
![]() |
[126] |
Knutti R, Sedláček J (2013) Robustness and uncertainties in the new CMIP5 climate model projections. Nat Clim Chang 3: 369–373. doi: 10.1038/nclimate1716
![]() |
[127] |
Zwiers FW, Kharin VV (1998) Changes in the extremes of the climate simulated by CCC GCM2 under CO2 doubling. J Climate 11: 2200–2222. doi: 10.1175/1520-0442(1998)011<2200:CITEOT>2.0.CO;2
![]() |
[128] |
Kharin VV, Zwiers FW (2000). Changes in the extremes in an ensemble of transient climate simulations with a coupled atmosphere–ocean GCM. J Climate 13: 3760–3788. doi: 10.1175/1520-0442(2000)013<3760:CITEIA>2.0.CO;2
![]() |
[129] | Cubash U, Meehl GA. Projections of future climate change. In Climate Change 2001: The Scientific Basis.; Contribution of Working Group 1 to the Third IPCC Scientific Assessment; Cambridge University Press: UK, 2001: 524–582. |
[130] |
Semenov V, Bengtsson L (2002) Secular trends in daily precipitation characteristics: greenhouse gas simulation with a coupled AOGCM. Clim Dyn 19: 123–140. doi: 10.1007/s00382-001-0218-4
![]() |
[131] | Murdoch PS, Baron JS, Miller TL (2000) Potential effects of climate change on surface water quality in North America 1. JAWRA 36: 347–366. |
[132] |
Srinivasan R, Arnold JG, Jones CA (1998) Hydrologic modelling of the United States with the Soil and Water Assessment Tool. Int J Water Resour Dev 14: 315–325. doi: 10.1080/07900629849231
![]() |
[133] | Arnold JG, Williams JR, Srinivasan R, et al. SWAT-Soil and Water Assessment Tool User Manual, 2018. Available from: https://swat.tamu.edu/media/69296/swat-io-documentation-2012.pdf. |
[134] |
Pease LM, Oduor P, Padmanabhan G (2010) Estimating sediment, nitrogen, and phosphorous loads from the Pipestem Creek watershed, North Dakota, using AnnAGNPS. Comput Geosci 36: 282–291. doi: 10.1016/j.cageo.2009.07.004
![]() |
[135] | Borah DK, Xia R, Bera M. DWSM−A dynamic watershed simulation model for studying agricultural nonpoint−source pollution. Paper number 012028, ASAE Annual Meeting St. Joseph, Michigan, 2001. |
[136] | Bicknell BR, Imhoff JC, Kittle JL, et al. Hydrological Simulation Program--Fortran, User's manual for version 11: U.S. Environmental Protection Agency; U.S. Environmental Protection Agency: National Exposure Research Laboratory, 1997, 755. |
[137] |
Singh VP, Frevert DK (2002) Mathematical models of large watershed hydrology. J Hydrol Eng 7: 270–292. doi: 10.1061/(ASCE)1084-0699(2002)7:4(270)
![]() |
[138] |
Shen Z, Liao Q, Hong Q, Gong Y (2012) An overview of research on agricultural non-point source pollution modelling in China. Sep Pur Technol 84: 104–111. doi: 10.1016/j.seppur.2011.01.018
![]() |
[139] | Weller DE, Baker ME (2014) Cropland riparian buffers throughout Chesapeake Bay Watershed: Spatial patterns and effects on nitrate loads delivered to streams. JAWRA 50: 696–712. |
[140] |
Sharifi A, Lang MW, McCarty GW, et al. (2016) Improving model prediction reliability through enhanced representation of wetland soil processes and constrained model auto calibration – A paired watershed study. J Hydrol 541: 1088–1103. doi: 10.1016/j.jhydrol.2016.08.022
![]() |
[141] |
Borah DK, Bera M (2003) Watershed-scale hydrologic and nonpoint-source pollution models: Review of mathematical bases. T ASAE 46: 1553–1566. doi: 10.13031/2013.15644
![]() |
[142] |
Robson BJ (2014) State of the art in modelling of phosphorus in aquatic systems: Review, criticisms and commentary. Environ Modell Softw 61: 339–359. doi: 10.1016/j.envsoft.2014.01.012
![]() |
[143] |
Rinke A, Dethloff K, Cassano JJ, et al. (2006) Evaluation of an ensemble of Arctic regional climate models: spatiotemporal fields during the SHEBA year. Clim Dyn 26: 459–472. doi: 10.1007/s00382-005-0095-3
![]() |
[144] |
Mohammed IN, Bomblies A, Wemple BC (2015) The use of CMIP5 data to simulate climate change impacts on flow regime within the Lake Champlain Basin. J Hydrol Reg Stud 3: 160–186. doi: 10.1016/j.ejrh.2015.01.002
![]() |
[145] |
Nour MH, Smith DW, El-Din MG, et al. (2008) Effect of watershed subdivision on water-phase phosphorus modelling: An artificial neural network modelling application. J Environ Eng Sci 7: 95–108. doi: 10.1139/S08-043
![]() |
[146] |
Kim RJ, Loucks DP, Stedinger JR (2012) Artificial neural network models of watershed nutrient loading. Water Resour Manage 26: 2781–2797. doi: 10.1007/s11269-012-0045-x
![]() |
[147] | Tang X, Xia M, Guan F, et al. (2016) Spatial distribution of soil nitrogen, phosphorus and potassium stocks in Moso bamboo forests in subtropical China. Forests 7, 267. |
[148] |
Mohanty S, Jha MK, Kumar A, et al. (2010) Artificial neural network modeling for groundwater level forecasting in a river island of eastern India. Water Resour Manage 24: 1845–1865. doi: 10.1007/s11269-009-9527-x
![]() |
[149] |
Kalin L, Isik S, Schoonover JE, et al. (2010) Predicting water quality in unmonitored watersheds using artificial neural networks. J Environ Qual 39: 1429–1440. doi: 10.2134/jeq2009.0441
![]() |
[150] |
Fogelman S, Blumenstein M, Zhao H (2006) Estimation of chemical oxygen demand by ultraviolet spectroscopic profiling and artificial neural networks. Neural Comput Applic 15: 197–203. doi: 10.1007/s00521-005-0015-9
![]() |
[151] |
Dogan E, Sengorur B, Koklu R (2009) Modeling biological oxygen demand of the Melen River in Turkey using an artificial neural network technique. J Environ Manag 90: 1229–1235. doi: 10.1016/j.jenvman.2008.06.004
![]() |
[152] |
Iliadis LS, Maris F (2007) An artificial neural network model for mountainous water-resources management: The case of Cyprus mountainous watersheds. Environ Modell Softw 22: 1066–1072. doi: 10.1016/j.envsoft.2006.05.026
![]() |
[153] |
Noori N, Kalin L (2016) Coupling SWAT and ANN models for enhanced daily streamflow prediction. J Hydrol 533: 141–151. doi: 10.1016/j.jhydrol.2015.11.050
![]() |
[154] |
Okkan U, Serbes ZA (2012) Rainfall–runoff modeling using least squares support vector machines. Environmetrics 23: 549–564. doi: 10.1002/env.2154
![]() |
[155] |
Huang S, Chang J, Huang Q, Chen Y (2014) Monthly streamflow prediction using modified EMD-based support vector machine. J Hydrol 511: 764–775. doi: 10.1016/j.jhydrol.2014.01.062
![]() |
[156] | Jajarmizadeh M, Harun S, Salarpour M. An assessment of a proposed hybrid neural network for daily flow prediction in arid climate, 2014. Available from: https://www.hindawi.com/journals/mse/2014/635018. |
[157] | Gassman P, Reyes M, Green C, Arnold J (2007) The Soil and Water Assessment Tool: Historical development, applications, and future research directions. T ASABE 7: 1211–1250. |
[158] | Niraula R, Kalin L, Wang R, et al. (2012) Determining nutrient and sediment critical source areas with SWAT model: effect of lumped calibration. T ASABE 55: 137–147. |
[159] | White M, Gambone M, Yen H, et al. (2015) Regional blue and green water balances and use by selected crops in the U.S. JAWRA 51: 1626–1642. |
[160] |
Wang R, Bowling LC, Cherkauer KA (2016) Estimation of the effects of climate variability on crop yield in the Midwest USA. Agric For Meteorol 216: 141–156. doi: 10.1016/j.agrformet.2015.10.001
![]() |
[161] | Yen H, White MJ, Arnold JG, et al. (2016) Western Lake Erie Basin: Soft-data-constrained, NHDPlus resolution watershed modeling and exploration of applicable conservation scenarios. Sci Total Environ 569–570: 1265–1281. |
[162] |
Arnold JG, Fohner N (2005) Current capabilities and research opportunities in applied watershed modeling. Hydrol Process 19: 563–572. doi: 10.1002/hyp.5611
![]() |
[163] | Santhi C, Arnold JG, Williams JR, et al. (2001) Validation of the Swat Model on a Large Rwer Basin with Point and Nonpoint Sources. JAWRA 37: 1169–1188. |
[164] |
Bosch DD, Sheridan JM, Batten HL, et al. (2004) Evaluation of the SWAT model on a coastal plain agricultural watershed. T ASAE 47: 1493–1506. doi: 10.13031/2013.17629
![]() |
[165] |
Saleh A, Du B (2004) Evaluation of SWAT and HSPF within BASINS program for the Upper North Bosque River watershed in central Texas. T ASAE 47: 1039–1049. doi: 10.13031/2013.16577
![]() |
[166] |
Tripathi MP, Panda RK, Raghuwanshi NS (2005) Development of effective management plan for critical subwatersheds using SWAT model. Hydrol Process 19: 809–826. doi: 10.1002/hyp.5618
![]() |
[167] | Grunwald S, Qi C (2006) Gis-Based Water Quality Modeling in the Sandusky Watershed, Ohio, USA. JAWRA 42: 957–973. |
[168] | Setegn SG, Srinivasan R, Dargahi B (2008) Hydrological Modelling in the Lake Tana Basin, Ethiopia Using SWAT Model. TOHJ, 2: 49–62. |
[169] | SWAT Literature Database SWAT Literature Database for Peer-Reviewed Journal Articles Available from: https://www.card.iastate.edu/swat_articles. |
[170] |
Tuo Y, Chiogna G, Disse M, et al. (2015) A multi-criteria model selection protocol for practical applications to nutrient transport at the catchment scale. Water 7: 2851–2880. doi: 10.3390/w7062851
![]() |
[171] |
Krysanova V, Hattermann F, Huang S, et al. (2015) Modelling climate and land-use change impacts with SWIM: lessons learnt from multiple applications. Hydrol Sci J 60: 606–635. doi: 10.1080/02626667.2014.925560
![]() |
[172] |
Čerkasova N, Umgiesser G, Ertürk A (2018) Development of a hydrology and water quality model for a large transboundary river watershed to investigate the impacts of climate change – A SWAT application. Ecol Eng 124: 99–115. doi: 10.1016/j.ecoleng.2018.09.025
![]() |
[173] | Cho J, Oh C, Choi J, et al. (2018) Climate change impacts on agricultural non-point source pollution with consideration of uncertainty in CMIP5. Irrig Drain 65: 209–220. |
[174] |
Yang X, Warren R, He Y, et al. (2018) Impacts of climate change on TN load and its control in a River Basin with complex pollution sources. Sci Total Environ 615: 1155–1163. doi: 10.1016/j.scitotenv.2017.09.288
![]() |
[175] |
Lee S, Yeo I-Y, Sadeghi AM, et al. (2018) Comparative analyses of hydrological responses of two adjacent watersheds to climate variability and change using the SWAT model. Hydrol Earth Syst Sci 22: 689–708. doi: 10.5194/hess-22-689-2018
![]() |
[176] |
Jha MK, Gassman PW, Panagopoulos Y (2015) Regional changes in nitrate loadings in the Upper Mississippi River Basin under predicted mid-century climate. Reg Environ Change 15: 449–460. doi: 10.1007/s10113-013-0539-y
![]() |
[177] |
Chiew FHS, McMahon TA (1993) Detection of trend or change in annual flow of Australian rivers. Int J Clim 13: 643–653. doi: 10.1002/joc.3370130605
![]() |
[178] |
Gellens D, Roulin E (1998) Streamflow response of Belgian catchments to IPCC climate change scenarios. J Hydrol 210: 242–258. doi: 10.1016/S0022-1694(98)00192-9
![]() |
[179] | Jha M, Gassman PW, Secchi S, et al. (2004) Effect of watershed subdivision on Swat flow, sediment, and nutrient predictions. JAWRA 40: 811–825. |
[180] |
Glavan M, Ceglar A, Pintar M (2015) Assessing the impacts of climate change on water quantity and quality modelling in small Slovenian Mediterranean catchment – lesson for policy and decision makers. Hydrol Process 29: 3124–3144. doi: 10.1002/hyp.10429
![]() |
[181] |
Kroeze C, Seitzinger SP (1998) Nitrogen inputs to rivers, estuaries and continental shelves and related nitrous oxide emissions in 1990 and 2050: a global model. Nutr Cycl Agroecosys 52: 195–212. doi: 10.1023/A:1009780608708
![]() |
[182] |
Seitzinger SP, Kroeze C, Bouwman AF, et al. (2002) Global patterns of dissolved inorganic and particulate nitrogen inputs to coastal systems: Recent conditions and future projections. Estuaries 25: 640–655. doi: 10.1007/BF02804897
![]() |
[183] |
Galloway JN, Dentener FJ, Capone DG, et al. (2004) Nitrogen cycles: Past, present, and future. Biogeochemistry 70: 153–226. doi: 10.1007/s10533-004-0370-0
![]() |
[184] | Bouwman AF, Drecht GV, Knoop JM, et al. (2005) Exploring changes in river nitrogen export to the world's oceans. Global Biogeochem Cy 19: GB1002. |
[185] |
Tilman D, Fargione J, Wolff B, et al. (2001) Forecasting agriculturally driven global environmental change. Science 292: 281–284. doi: 10.1126/science.1057544
![]() |
[186] | Fisher R (2013) Cost estimates of phosphorus removal at wastewater treatment plants.; Ohio Environmental Protection Agency, p. 50. |
[187] |
Kronvang B, Bechmann M, Pedersen ML, et al. (2003) Phosphorus dynamics and export in streams draining micro-catchments: Development of empirical models. J Plant Nutr Soil Sci 166: 469–474. doi: 10.1002/jpln.200321164
![]() |
[188] |
Osmond D, Meals D, Hoag D, et al. (2012). Improving conservation practices programming to protect water quality in agricultural watersheds: Lessons learned from the National Institute of Food and Agriculture–Conservation Effects Assessment Project. J Soil Water Conserv 67: 122–127. doi: 10.2489/jswc.67.2.122
![]() |
[189] | Gburek WJ, Drungil CC, Srinivasan MS, et al. (2002) Variable-source-area controls on phosphorus transport: Bridging the gap between research and design. J Soil Water Conserv 57: 534–543. |
[190] |
Gitau MW, Veith TL, Gburek WJ (2004) Farm-level optimization of BMP placement for cost-effective pollution reduction. T ASAE 47: 1923–1931. doi: 10.13031/2013.17805
![]() |
[191] | Arabi M, Govindaraju RS, Hantush MM (2006) Cost‐effective allocation of watershed management practices using a genetic algorithm - Arabi. Water Resouce Res 42: W10429. |
[192] | Caswell M, Fuglie K, Ingram C, et al. Adoption of agricultural production practices. Economic Research Service, Agricultural Economic Report No. 792, USDA, 2001. |
[193] |
Gabriel M, Knightes C, Cooter E, et al. (2018) Modeling the combined effects of changing land cover, climate, and atmospheric deposition on nitrogen transport in the Neuse River Basin. J Hydrol Reg Stud 18: 68–79. doi: 10.1016/j.ejrh.2018.05.004
![]() |
[194] |
Holman IP, Rounsevell MDA, Shackley S, et al. (2005) A regional, multi-sectoral and integrated assessment of the impacts of climate and socio-economic change in the UK. Climatic Change 71: 9–41. doi: 10.1007/s10584-005-5927-y
![]() |
[195] |
Dowd BM, Press D, Huertos ML (2008) Agricultural nonpoint source water pollution policy: The case of California's Central Coast. Agr Ecosyst Environ 128: 151–161. doi: 10.1016/j.agee.2008.05.014
![]() |
1. | Karina Yew-Hoong Gin, Zhi Yang Sim, Kwan Chien Goh, Jerome Wai Kit Kok, Shu Harn Te, Ngoc Han Tran, Wenxuan Li, Yiliang He, Novel cyanotoxin-producing Synechococcus in tropical lakes, 2021, 192, 00431354, 116828, 10.1016/j.watres.2021.116828 | |
2. | Eric Ariel L. Salas, Sakthi Kumaran Subburayalu, Analysis of phosphorus and nitrogen concentrations in the Great Miami and Little Miami basins in Ohio, USA from 2015 to 2017, 2020, 36, 1535-1459, 1345, 10.1002/rra.3604 | |
3. | O. O. Seminskaya, M. N. Balakina, D. D. Kucheruk, A. V. Nanieva, Effect of Concomitant Ions on Nanofiltration Dephosphatation of Water, 2020, 42, 1063-455X, 196, 10.3103/S1063455X20030091 | |
4. | Cheryl R. Dykstra, Jeffrey L. Hays, Melinda M. Simon, Ann R. Wegman, Laura R. Dykstra, Kelly A. Williams, Habitat and weather conditions influence reproductive rates of suburban and rural Red‐shouldered Hawks Buteo lineatus , 2021, 163, 0019-1019, 623, 10.1111/ibi.12877 | |
5. | Kelly A. Williams, Jacob P. Sieg, Laura R. Dykstra, Cheryl R. Dykstra, Sitting in the open: how nest microclimate influences incubation behavior in an open‐cup nesting passerine, 2025, 2025, 0908-8857, 10.1111/jav.03385 |
Land cover | 2001 | 2006 | 2011 |
Water body | 0.98% | 0.99% | 1.01% |
Urban area | 17.32% | 17.58% | 17.81% |
Forested | 11.55% | 11.59% | 11.54% |
Agricultural (crops, pasture, and hay) | 68.57% | 68.25% | 68.06% |
Other | 1.58% | 1.58% | 1.59% |
Land cover | 2001 | 2006 | 2011 |
Water body | 1.0% | 0.97% | 0.98% |
Urban area | 17.8% | 18.45% | 18.74% |
Forested | 23.7% | 23.64% | 23.49% |
Agricultural (crops, pasture, and hay) | 56.2% | 55.60% | 55.41% |
Other | 1.4% | 1.35% | 1.37% |
Crop | Acres | NT | MT (30% residue) | RDT (15–30% residue) | CVT (0–15% residue) |
Soybean | 4,630,915 | 63.7% | 9.0% | 8.3% | 19.0% |
Corn | 3,527,939 | 23.5% | 9.9% | 13.1% | 53.4% |
High nutrient flux | Watershed (HUC 8#) | N Flux (kg/km2) | P Flux (kg/km2) |
N&P | Upper Wabash (05120101)* | 2159 | 254 |
N | Upper Great Miami (05080001)* | 1790 | 50 |
N | Upper Scioto (05060001)* | 1786 | 88 |
N&P | Lower Great Miami (05080002)* | 1735 | 127 |
N | Little Miami (05090202) | 1653 | 99 |
N | Paint (05060003) | 1405 | 65 |
N&P | Mill Creek (05090203) | 1345 | 174 |
P | Ohio Brush-Whiteoak (05090201) | 1218 | 126 |
Stream category | Drainage area (m2) | EWH TP (mg/L) | WWH TP (mg/L) | EWH Nitrate + Nitrite (mg/L) | WWH Nitrate + Nitrite (mg/L) |
Headwater | < 20 | 0.05 | 0.08 | 0.50 | 1.0 |
Wadable | 20 to < 200 | 0.05 | 0.10 | 0.50 | 1.0 |
Small river | 200 to < 1000 | 0.10 | 0.17 | 1.0 | 1.5 |
Large river | > 1000 | 0.15 | 0.30 | 1.5 | 2.0 |
Nitrogen | SWAT | GWLF | HSPF |
Number of input requirements | 33 | 8 | 10 |
Number of nutrient sources | 11 | 7 | 4 |
Phosphorus | |||
Number of input requirements | 18 | 8 | 5 |
Number of nutrient sources | 10 | 7 | 4 |
Nitrogen | SWAT | GWLF | HSPF |
Number of outputs | 28 | 6 | 23 |
Number of transport processes | 8 | 5 | 6 |
Phosphorus | |||
Number of outputs | 19 | 6 | 12 |
Number of transport processes | 6 | 5 | 6 |
Land cover | 2001 | 2006 | 2011 |
Water body | 0.98% | 0.99% | 1.01% |
Urban area | 17.32% | 17.58% | 17.81% |
Forested | 11.55% | 11.59% | 11.54% |
Agricultural (crops, pasture, and hay) | 68.57% | 68.25% | 68.06% |
Other | 1.58% | 1.58% | 1.59% |
Land cover | 2001 | 2006 | 2011 |
Water body | 1.0% | 0.97% | 0.98% |
Urban area | 17.8% | 18.45% | 18.74% |
Forested | 23.7% | 23.64% | 23.49% |
Agricultural (crops, pasture, and hay) | 56.2% | 55.60% | 55.41% |
Other | 1.4% | 1.35% | 1.37% |
Crop | Acres | NT | MT (30% residue) | RDT (15–30% residue) | CVT (0–15% residue) |
Soybean | 4,630,915 | 63.7% | 9.0% | 8.3% | 19.0% |
Corn | 3,527,939 | 23.5% | 9.9% | 13.1% | 53.4% |
High nutrient flux | Watershed (HUC 8#) | N Flux (kg/km2) | P Flux (kg/km2) |
N&P | Upper Wabash (05120101)* | 2159 | 254 |
N | Upper Great Miami (05080001)* | 1790 | 50 |
N | Upper Scioto (05060001)* | 1786 | 88 |
N&P | Lower Great Miami (05080002)* | 1735 | 127 |
N | Little Miami (05090202) | 1653 | 99 |
N | Paint (05060003) | 1405 | 65 |
N&P | Mill Creek (05090203) | 1345 | 174 |
P | Ohio Brush-Whiteoak (05090201) | 1218 | 126 |
Stream category | Drainage area (m2) | EWH TP (mg/L) | WWH TP (mg/L) | EWH Nitrate + Nitrite (mg/L) | WWH Nitrate + Nitrite (mg/L) |
Headwater | < 20 | 0.05 | 0.08 | 0.50 | 1.0 |
Wadable | 20 to < 200 | 0.05 | 0.10 | 0.50 | 1.0 |
Small river | 200 to < 1000 | 0.10 | 0.17 | 1.0 | 1.5 |
Large river | > 1000 | 0.15 | 0.30 | 1.5 | 2.0 |
Nitrogen | SWAT | GWLF | HSPF |
Number of input requirements | 33 | 8 | 10 |
Number of nutrient sources | 11 | 7 | 4 |
Phosphorus | |||
Number of input requirements | 18 | 8 | 5 |
Number of nutrient sources | 10 | 7 | 4 |
Nitrogen | SWAT | GWLF | HSPF |
Number of outputs | 28 | 6 | 23 |
Number of transport processes | 8 | 5 | 6 |
Phosphorus | |||
Number of outputs | 19 | 6 | 12 |
Number of transport processes | 6 | 5 | 6 |