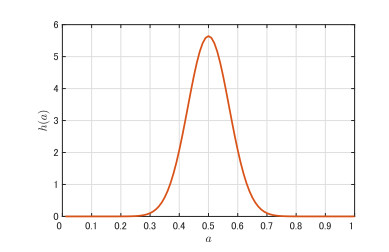
Citation: Edward F. Durner. Fall nitrogen enhances spring nitrogen enhanced flowering in the long day strawberry cultivar ‘Elan’[J]. AIMS Agriculture and Food, 2017, 2(2): 149-164. doi: 10.3934/agrfood.2017.2.149
[1] | Sara R Robertson, Robert JC McLean . Beneficial biofilms. AIMS Bioengineering, 2015, 2(4): 437-448. doi: 10.3934/bioeng.2015.4.437 |
[2] | Ana Meireles, Ana L. Gonçalves, Inês B. Gomes, Lúcia Chaves Simões, Manuel Simões . Methods to study microbial adhesion on abiotic surfaces. AIMS Bioengineering, 2015, 2(4): 297-309. doi: 10.3934/bioeng.2015.4.297 |
[3] | Amreeta Sarjit, Sin Mei Tan, Gary A. Dykes . Surface modification of materials to encourage beneficial biofilm formation. AIMS Bioengineering, 2015, 2(4): 404-422. doi: 10.3934/bioeng.2015.4.404 |
[4] | Arindam Mitra, Suman Mukhopadhyay . Biofilm mediated decontamination of pollutants from the environment. AIMS Bioengineering, 2016, 3(1): 44-59. doi: 10.3934/bioeng.2016.1.44 |
[5] | Frank R. Bengelsdorf, Christina Gabris, Lisa Michel, Manuel Zak, Marian Kazda . Syntrophic microbial communities on straw as biofilm carrier increase the methane yield of a biowaste-digesting biogas reactor. AIMS Bioengineering, 2015, 2(3): 264-276. doi: 10.3934/bioeng.2015.3.264 |
[6] | Chiara Ceresa, Maurizio Rinaldi, Letizia Fracchia . Synergistic activity of antifungal drugs and lipopeptide AC7 against Candida albicans biofilm on silicone. AIMS Bioengineering, 2017, 4(2): 318-334. doi: 10.3934/bioeng.2017.2.318 |
[7] | Wiebke Wesseling . Beneficial biofilms in marine aquaculture? Linking points of biofilm formation mechanisms in Pseudomonas aeruginosa and Pseudoalteromonas species. AIMS Bioengineering, 2015, 2(3): 104-125. doi: 10.3934/bioeng.2015.3.104 |
[8] | Liwei Chen, Jaslyn Lee, Wei Ning Chen . The use of metabolic engineering to produce fatty acid-derived biofuel and chemicals in Saccharomyces cerevisiae: a review. AIMS Bioengineering, 2016, 3(4): 468-492. doi: 10.3934/bioeng.2016.4.468 |
[9] | Yung-Hua Li, Xingxing Huang, Xiao-Lin Tian . Recent advances in dental biofilm: impacts of microbial interactions on the biofilm ecology and pathogenesis. AIMS Bioengineering, 2017, 4(3): 335-350. doi: 10.3934/bioeng.2017.3.335 |
[10] | Frank B Dazzo, Youssef G Yanni, Ashley Jones, Abdelgawad Y Elsadany . CMEIAS bioimage informatics that define the landscape ecology of immature microbial biofilms developed on plant rhizoplane surfaces. AIMS Bioengineering, 2015, 2(4): 469-486. doi: 10.3934/bioeng.2015.4.469 |
In this paper, we study the following general class of functional differential equations with distributed delay and bistable nonlinearity,
{x′(t)=−f(x(t))+∫τ0h(a)g(x(t−a))da,t>0,x(t)=ϕ(t),−τ≤t≤0. | (1.1) |
Many mathematical models issued from ecology, population dynamics and other scientific fields take the form of Eq (1.1) (see, e.g., [1,2,3,4,5,6,7,8,9,10,11,12,13,14,15] and references therein). When a spatial diffusion is considered, many models are studied in the literature (see, e.g., [16,17,18,19,20,21,22]).
For the case where g(x)=f(x) has not only the trivial equilibrium but also a unique positive equilibrium, Eq (1.1) is said to be a problem with monostable nonlinearity. In this framework, many authors studied problem (1.1) with various monostable nonlinearities such as Blowflies equations, where f(x)=μx and g(x)=βxe−αx, and Mackey-Glass equations, where f(x)=μx and g(x)=βx/(α+xn) (see, e.g., [1,3,6,7,9,10,11,12,13,21,23,24,25,26,27]).
When g(x) allows Eq (1.1) to have two positive equilibria x∗1 and x∗2 in addition to the trivial equilibrium, Eq (1.1) is said to be a problem with bistable nonlinearity. In this case, Huang et al. [5] investigated the following general equation:
x′(t)=−f(x(t))+g(x(t−τ)). |
The authors described the basins of attraction of equilibria and obtained a series of invariant intervals using the decomposition domain. Their results were applied to models with Allee effect, that is, f(x)=μx and g(x)=βx2e−αx. We point out that the authors in [5] only proved the global stability for x∗2≤M, where g(M)=maxs∈R+g(s). In this paper, we are interested in the dynamics of the bistable nonlinearity problem (1.1). More precisely, we will present some attracting intervals which will enable us to give general conditions on f and g that ensure global asymptotic stability of equilibria x∗1 and x∗2 in the both cases x∗2<M and x∗2≥M.
The paper is organized as follows: in Section 2, we give some preliminary results including existence, uniqueness and boundedness of the solution as well as a comparison result. We finish this section by proving the global asymptotic stability of the trivial equilibrium. Section 3 is devoted to establish the attractive intervals of solutions and to prove the global asymptotic stability of the positive equilibrium x∗2. In Section 4, we investigate an application of our results to a model with Allee effect. In Section 5, we perform numerical simulation that supports our theoretical results. Finally, Section 6 is devoted to the conclusion.
In the whole paper, we suppose that the function h is positive and
∫τ0h(a)da=1. |
We give now some standard assumptions.
(T1) f and g are Lipschitz continuous with f(0)=g(0)=0 and there exists a number B>0 such that maxv∈[0,s]g(v)<f(s) for all s>B.
(T2) f′(s)>0 for all s≥0.
(T3) g(s)>0 for all s>0 and there exists a unique M>0 such that g′(s)>0 for 0<s<M, g′(M)=0 and g′(s)<0 for s>M.
(T4) There exist two positive constants x∗1 and x∗2 such that g(x)<f(x) if x∈(0,x∗1)∪(x∗2,∞) and g(x)>f(x) if x∈(x∗1,x∗2) and g′(x∗1)>f′(x∗1).
Let C:=C([−τ,0],R) be the Banach space of continuous functions defined in [−τ,0] with ||ϕ||=supθ∈[−τ,0]|ϕ(θ)| and C+={ϕ∈C;ϕ(θ)≥0,−τ≤θ≤0} is the positive cone of C. Then, (C,C+) is a strongly ordered Banach space. That is, for all ϕ,ψ∈C, we write ϕ≥ψ if ϕ−ψ∈C+, ϕ>ψ if ϕ−ψ∈C+∖{0} and ϕ>>ψ if ϕ−ψ∈Int(C+). We define the following ordered interval:
C[ϕ,ψ]:={ξ∈C;ϕ≤ξ≤ψ}. |
For any χ∈R, we write χ∗ for the element of C satisfying χ∗(θ)=χ for all θ∈[−τ,0]. The segment xt∈C of a solution is defined by the relation xt(θ)=x(t+θ), where θ∈[−τ,0] and t≥0. In particular, x0=ϕ. The family of maps
Φ:[0,∞)×C+→C+, |
such that
(t,ϕ)→xt(ϕ) |
defines a continuous semiflow on C+ [28]. For each t≥0, the map Φ(t,.) is defined from C+ to C+ which is denoted by Φt:
Φt(ϕ)=Φ(t,ϕ). |
The set of equilibria of the semiflow, which is generated by (1.1), is given by
E={χ∗∈C+;χ∈Randg(χ)=f(χ)}. |
In this section, we first provide existence, uniqueness and boundedness of solution to problem (1.1). We then present a Lyapunov functional and show the global asymptotic stability of the trivial equilibrium. We begin by recalling a useful theorem related to a comparison principle (see Theorem 1.1 in page 78 in [29]).
We consider the following problem:
{x′(t)=F(xt),t>0,x(t)=ϕ(t),−τ≤t≤0, | (2.1) |
where F:Ω→R is continuous on Ω, which is an open subset of C. We write x(t,ϕ,F) for the maximal defined solution of problem (2.1). When we need to emphasize the dependence of a solution on initial data, we write x(t,ϕ) or x(ϕ).
Theorem 2.1. Let f1, f2 :Ω→R be continuous, Lipschitz on each compact subset of Ω, and assume that either f1 or f2 is a nondecreasing function, with f1(ϕ)≤f2(ϕ) for all ϕ∈Ω. Then
x(t,ϕ,f1)≤x(t,ϕ,f2), |
holds for all t≥0, for which both are defined.
The following lemma states existence, uniqueness and boundedness of the positive solution to problem (1.1). For the proof, see [15,30,31].
Lemma 2.2. Suppose that (T1) holds. For any ϕ∈C+, the problem (1.1) has a unique positive solution x(t):=x(t,ϕ) on [0,∞) satisfying x0=ϕ, provided ϕ(0)>0. In addition, we have the following estimate:
0≤lim supt→∞x(t)≤B. |
Moreover, the semiflow Φt admits a compact global attractor, which attracts every bounded set in C+.
The following lemma can be easily proved (see also the proof of Theorem 1.1 in [29]).
Lemma 2.3. Let ϕ∈C be a given initial condition, x(ϕ) be the solution of problem (1.1) and xϵ(ϕ), ϵ>0 be the solution of problem (1.1) when replacing f by f±ϵ. Then
xϵ(t,ϕ)→x(t,ϕ) as ϵ→0, for all t∈[0,∞). |
We focus now on the global stability of the trivial equilibrium. For this purpose, we suppose that
f(s)>g(s) for all s∈(0,x∗1) and f(x∗1)=g(x∗1). | (2.2) |
Lemma 2.4. Assume that (T1) and condition (2.2) hold. For a given ϕ∈C[0,x∗1]∖{x∗1}, the solution x(ϕ) of problem (1.1) satisfies
lim supt→∞x(t)<x∗1, | (2.3) |
provided that one of the following hypotheses holds:
(i) g is a nondecreasing function over (0,x∗1).
(ii) f is a nondecreasing function over (0,x∗1).
Proof. Without loss of generality, we assume that ϕ(0)<x∗1. Suppose that (ⅰ) holds. We first claim that x(t)<x∗1 for all t≥0. Let xϵ(ϕ) be the solution of problem (1.1) by replacing f by f+ϵ. We prove that xϵ(t):=xϵ(t,ϕ)≤x∗1 for all t>0. Suppose, on the contrary, that there exists t1>0 such that xϵ(t1)=x∗1, xϵ(t)≤x∗1 for all t≤t1 and x′ϵ(t1)≥0. Then, using the equation of xϵ(t1), we have
0≤x′ϵ(t1)=−f(xϵ(t1))−ϵ+∫τ0h(a)g(xϵ(t1−a))da,<−f(x∗1)+g(x∗1)=0, |
which leads to a contradiction. Now, applying Lemma 2.3, we obtain x(t)≤x∗1 for all t≥0. Next, set X(t)=x∗1−x(t). We then have
X′(t)=f(x(t))−f(x∗1)+∫τ0h(a)(g(x∗1)−g(x(t−a)))da. |
Since x(t)≤x∗1 for all t≥0 and g is a nondecreasing function, we have, for t≥τ,
X′(t)≥−LX(t), |
where L is the Lipschitz constant of f. Consequently, X(t)≥X(0)e−Lt and the claim is proved. Finally, to prove inequality (2.3), we suppose, on the contrary, that there exist an increasing sequence (tn)n, tn→∞, t0≥τ and a nondecreasing sequence (x(tn))n, such that x(tn)→x∗1 as tn→∞, x(tn)<x∗1 for some n and x(tn)=maxt∈[0,tn]x(t). Then, in view of condition (2.2), the equation of x(tn) satisfies
0≤x′(tn)=−f(x(tn))+∫τ0h(a)g(x(tn−a))da,≤−f(x(tn))+g(x(tn))<0, |
which is a contradiction.
Suppose now that (ⅱ) holds. Let xϵ(t) be the solution of problem (1.1) by replacing f(s) by f(s)+ϵ and g(s) by g+(s)=maxσ∈[0,s]g(σ).
Then, again by contradiction, suppose that there exists t1>0 such that xϵ(t1)=x∗1, xϵ(t)≤x∗1 for all t≤t1 and x′ϵ(t1)≥0. Then, arguing as above, we obtain
0≤x′ϵ(t1)=−f(xϵ(t1))−ϵ+∫τ0h(a)g+(xϵ(t−a))da,<−f(x∗1)+g+(x∗1). | (2.4) |
Since f is a nondecreasing function and g+ is the smallest nondecreasing function that is greater than g, we obtain f(s)>g+(s) for all s∈(0,x∗1). This contradicts with inequality (2.4). Further, by combining Theorem 2.1 and Lemma 2.3, we get x(t)≤x∗1 for all t≥0. Finally, following the same arguments as in the first part of this proof, the lemma is proved.
We now prove the global asymptotic stability of the trivial equilibrium.
Theorem 2.5. Assume that (T1) and condition (2.2) hold. Suppose also that ϕ∈C[0,x∗1]∖{x∗1}. The trivial equilibrium is globally asymptotically stable if one of the following hypotheses holds:
(i) g is a nondecreasing function over (0,x∗1).
(ii) f is a nondecreasing function over (0,x∗1).
Proof. Suppose that (ⅰ) holds. Let V be the Lyapunov functional defined by
V(s)=s+∫τ0ψ(a)g(ϕ(−a))da, | (2.5) |
where ψ(a)=∫τah(σ)dσ. The derivative of V along the solution of problem (1.1) gives
dV(xt)dt=g(x(t))−f(x(t))∀t>0. |
From condition (2.2) and Lemma 2.4, we have dV(xt)/dt<0 and thus, the result is reached by classical Lyapunov theorem (see [6]). Next, suppose that (ⅱ) holds. Let the function V be defined in (2.5) by replacing g(s) by g+(s):=maxσ∈[0,s]g(σ). Since g+(s)<f(s) for all s∈(0,x∗1), we can employ the same argument as above to get dV(xt)/dt<0. Finally the result is obtained by applying Theorem 2.1 and Lemma 2.4. This completes the proof.
Now, suppose that
f(s)>g(s) for all s>0. | (2.6) |
Using the same proof as in Theorem 2.5, we immediately obtain the following theorem.
Theorem 2.6. Assume that (T1) and condition (2.6) hold. The trivial equilibrium is globally asymptotically stable for all ϕ∈C+.
The following theorem concerns the global stability of x∗2 in the case where g is a nondecreasing function.
Theorem 3.1. Suppose that ϕ∈C[x∗1,sups∈[−τ,0]ϕ]∖{x∗1} and g is a nondecreasing function. Assume also that (T1) and (T4) hold. Then, the positive equilibrium x∗2 is globally asymptotically stable.
Proof. Without loss of generality, suppose that ϕ(0)>x∗1. We first claim that lim inft→∞x(t)>x∗1. For this, let xϵ(t):=xϵ(t;ϕ) be the solution of problem (1.1) when replacing f by f−ϵ. To reach the claim, we begin by proving that xϵ(t)>x∗1 for all t≥0. Otherwise, there exists t1>0 such that xϵ(t1)=x∗1, xϵ(t)≥x∗1 for all t≤t1 and x′ϵ(t1)≤0. Then, the equation of xϵ(t1) satisfies
0≥x′ϵ(t1)=−f(xϵ(t1))+ϵ+∫τ0h(a)g(xϵ(t1−a))da>−f(x∗1)+g(x∗1)=0. |
This reaches a contradiction and thus xϵ(t)>x∗1 for all t>0. This result, together with Lemma 2.3, gives x(t)≥x∗1 for all t≥0.
Next, for X(t)=x(t)−x∗1 and since f(x∗1)=g(x∗1), the equation of X(t) satisfies
X′(t)=f(x∗1)−f(x(t))+∫τ0h(a)(g(x(t−a))−g(x∗1))da, |
this leads to
X′(t)≥−LX(t), |
where L is the Lipschitz constant of f. Consequently x(t)>x∗1 for all t>0.
Now, suppose that there exist an increasing sequence (tn)n, tn→∞, t0≥τ and a nonincreasing sequence (x(tn))n, such that x(tn)→x∗1 as tn→∞, x(tn)>x∗1 for some n and x(tn)=mint∈[0,tn]x(t). In view of (T4), the equation of x(tn) satisfies
0≥x′(tn)=−f(x(tn))+∫τ0h(a)g(x(tn−a))da,≥−f(x(tn))+g(x(tn))>0, |
which is a contradiction. The claim is proved.
To prove that x∗2 is globally asymptotically stable, we consider the following Lyapunov functional
V(ϕ)=∫ϕ(0)x∗2[g(s)−g(x∗2)]ds+12∫τ0ψ(a)[g(ϕ(−a))−g(x∗2)]2da, |
with ψ(a)=∫τah(σ)dσ. By a straightforward computation, the derivative of V along the solution of problem (1.1) gives, for all t>0,
dV(xt)dt=[g(x(t))−g(x∗2)]x′(t)−12∫τ0ψ(a)∂∂a[g(x(t−a))−g(x∗2)]2da=[g(x(t))−g(x∗2)][−f(x(t))+∫τ0h(a)g(x(t−a))da]−12{ψ(τ)[g(x(t−τ))−g(x∗2)]2−ψ(0)[g(x(t))−g(x∗2)]2−∫τ0ψ′(a)[g(x(t−a))−g(x∗2)]2da}=[g(x(t))−g(x∗2)][−f(x(t))+g(x(t))]+[g(x(t))−g(x∗2)][−g(x(t))+∫τ0h(a)g(x(t−a))da]+12∫τ0h(a)da[g(x(t))−g(x∗2)]2−12∫τ0h(a)[g(x(t−a))−g(x∗2)]2da=[g(x(t))−g(x∗2)][−f(x(t))+g(x(t))]+12∫τ0h(a){2[g(x(t))−g(x∗2)][−g(x(t))+g(x(t−a))]+[g(x(t))−g(x∗2)]2−[g(x(t−a))−g(x∗2)]2}da=[g(x(t))−g(x∗2)][−f(x(t))+g(x(t))]−12∫τ0h(a)[g(x(t))−g(x(t−a))]2da. |
Note that ψ(τ)=0. In view of (T4), we have dV(xt)/dt≤0. If g is an increasing function, then the result is reached by using a classical Lyapunov theorem (see, e.g., [30]). If g is a nondecreasing function, then the result is proved by using the same argument as in the proof of Theorem 2.6 in [31]. This completes the proof.
We focus now on the case where g is non-monotone. Suppose that there exists ˆG(x) such that ˆG(x)=ˆg−1og(x), where ˆg(.) denotes the restriction of g to the interval [M,∞). Then, ˆG(x)=x for x∈[M,∞) and ˆG(x)>M>x for x∈[0,M).
Lemma 3.2. Assume that (T1)–(T4) hold. For a given ϕ∈C[x∗1,ˆG(x∗1)]∖{x∗1}, let x be the solution of problem (1.1). Then, the following assertions hold:
(i) if x∗2<M, then x∗1<x(t)<ˆG(x∗1) for all t>0.
(ii) if x∗2≥M and f(ˆG(x∗1))>g(M), then x∗1<x(t)<ˆG(x∗1) for all t>0.
Proof. Without loss of generality, suppose that x∗1<ϕ(0)≤ˆG(x∗1). First, observe that, for a given ϕ∈C[x∗1,ˆG(x∗1)]∖{x∗1}, we have f(ˆG(x∗1))>f(x∗1)=g(x∗1)=g(ˆG(x∗1)), and thus, there exists ϵ>0 such that f(ˆG(x∗1))−ϵ>g(ˆG(x∗1)). We begin by proving that x∗1≤x(t)≤ˆG(x∗1) for all t>0. To this end, in view of Lemma 2.3, we only need to prove that x∗1<xϵ(t):=xϵ(t;ϕ)≤ˆG(x∗1) for all t>0, where xϵ(t):=xϵ(t;ϕ) is the solution of problem (1.1) when replacing f by f−ϵ. Let yϵ:=yϵ(ϕ) be the solution of
{y′ϵ(t)=−f(yϵ(t))+ϵ+∫τ0h(a)g+(yϵ(t−a))da,t>0,yϵ(t)=ϕ(t),−τ≤t≤0. | (3.1) |
with g+(s)=maxσ∈[0,s]g(σ). Since f is an increasing function, we have f(ˆG(x∗1))−ϵ>g+(ˆG(x∗1)). Accordingly, the function ˆG(x∗1) is a super-solution of problem (3.1). Finally, in view of Theorem 2.1, we obtain xϵ(t)≤yϵ(t)≤ˆG(x∗1) for all t≥0.
We now prove that xϵ(t)>x∗1. Suppose, on the contrary, that there exists t1>0 such that xϵ(t1)=x∗1, xϵ(t)≥x∗1 for all t≤t1, and thus, x′ϵ(t1)≤0. Then, the equation of xϵ(t1) satisfies
x′ϵ(t1)=−f(xϵ(t1))+ϵ+∫τ0h(a)g(xϵ(t1−a))da>−f(x∗1)+g(x∗1)=0, |
since g(s)≥g(x∗1) for all s∈[x∗1,ˆG(x∗1)]. This reaches a contradiction. Further, from Lemma 2.3, we obtain x(t)≥x∗1. The claim is proved.
Next, let X(t)=x(t)−x∗1. Since f(x∗1)=g(x∗1), the equation of X(t) satisfies
X′(t)=f(x∗1)−f(x(t))+∫τ0h(a)(g(x(t−a))−g(x∗1))da. |
We know that g(s)≥g(x∗1) for all s∈[x∗1,ˆG(x∗1)]. We then have
X′(t)≥−LX(t), |
where L is the Lipschitz constant of f. Consequently x(t)>x∗1 for all t>0.
Next, we prove that x(t)<ˆG(x∗1) for all t>0. Suppose, on the contrary, that there exists t1>0 such that x(t1)=ˆG(x∗1), x(t)≤ˆG(x∗1) for all t≤t1 and x′(t1)≥0. Then
0≤x′(t1)=−f(x(t1))+∫τ0h(a)g(x(t1−a))da,≤−f(ˆG(x∗1))+g(M). | (3.2) |
Since f is an increasing function and ˆG(x∗1)>M, we have
0≤x′(t1)≤−f(M)+g(M). | (3.3) |
Now, the assertion x∗2<M implies that g(M)<f(M), which leads to a contradiction.
When x∗2≥M, inequality (3.2) gives a contradiction by hypothesis. The Lemma is proved.
Using Lemma 3.2, we next prove the following lemma.
Lemma 3.3. Suppose that ϕ∈C[x∗1,ˆG(x∗1)]∖{x∗1}. Assume also that (T1)–(T4) hold. Let x be the solution of problem (1.1). Then, we have
lim inft→∞x(t)>x∗1, |
provided that one of the following assertions holds:
(i) x∗2<M.
(ii) x∗2≥M and f(ˆG(x∗1))>g(M).
Proof. Firstly, from Lemma 3.2, both assertions imply that x∗1<x(t)<ˆG(x∗1) for all t≥0. Next, observe that there exists ϵ>0 such that
g(s)>f(x∗1+ϵ)for alls∈[x∗1+ϵ,θϵ], |
with θϵ∈(M,ˆG(x∗1)) and g(θϵ)=g(x∗1+ϵ). Indeed, in view of (T3), minσ∈[x∗1+ϵ,θϵ]g(σ)=g(θϵ)=g(x∗1+ϵ) and from (T4), we have g(x∗1+ϵ)>f(x∗1+ϵ).
Now, consider the following nondecreasing function:
g_(s)={g(s),for 0<s<ˉm,f(x∗1+ϵ),for ˉm<s<ˆG(x∗1), | (3.4) |
where x∗1<ˉm<x∗1+ϵ, which is a constant satisfying g(ˉm)=f(x∗1+ϵ). From (T2) and (T4), we get g_(s)>f(s) for x∗1<s<x∗1+ϵ and g_(s)<f(s) for s>x∗1+ϵ. Let y(ϕ) be the solution of problem (1.1) when replacing g by g_. Then, according to Theorem 2.1, we have y(t;ϕ)≤x(t;ϕ). In addition, using Theorem 3.1, we obtain
x∗1<limt→∞y(t;ϕ)=x∗1+ϵ≤lim inft→∞x(t;ϕ). |
The lemma is proved.
Under Lemma 3.3, we prove the following theorem on the global asymptotic stability of the positive equilibrium x∗2<M.
Theorem 3.4. Suppose that ϕ∈C[x∗1,ˆG(x∗1)]∖{x∗1} and x∗2<M. Assume also that (T1)–(T4) hold. Then, the positive equilibrium x∗2 is globally asymptotically stable.
Proof. We first claim that there exists T>0 such that x(t)≤M for all t≥T. Indeed, let xϵ:=xϵ(ϕ) be the solution of problem (1.1) when replacing f by f+ϵ. First, suppose that there exists T>0 such that xϵ(t)≥M for all t≥T. So, since x∗2<M, we have from (T4) that g(M)<f(M). Combining this with (T2), the equation of xϵ satisfies
x′ϵ(t)≤−f(M)−ϵ+g(M)≤−ϵ, |
which contradicts with xϵ(t)≥M. Hence there exists T>0 such that xϵ(T)<M. We show that xϵ(t)<M for all t≥T. In fact, at the contrary, if there exists t1>T such that xϵ(t1)=M and so x′ϵ(t1)≥0 then,
x′ϵ(t1)=−f(M)−ϵ+g(M)<0, |
which is a contradiction. Further, according to Lemma 2.3, there exists T>0 such that x(t)≤M for all t≥T and the claim is proved. Finally, since g is a nondecreasing function over (0,M), the global asymptotic stability of x∗2 is proved by applying Theorem 3.1.
Remark 3.5. In the case where g(s)>g(x∗1) for all s>x∗1, the above theorem holds true. In fact, it suffices to replace ˆG(x∗1) in C[x∗1,ˆG(x∗1)]∖{x∗1} by sups∈[−τ,0]ϕ(s).
We focus now on the case where x∗2≥M. To this end, suppose that there exists a unique constant A such that
A≥M and g(A)=f(M). | (3.5) |
Lemma 3.6. Assume that (T1)–(T4) hold. Suppose also that x∗2≥M and f(A)≥g(M). For a given ϕ∈C[x∗1,ˆG(x∗1)]∖{x∗1} and the solution x:=x(ϕ) of problem (1.1), there exists T>0 such that
M≤x(t)≤A for all t≥T. |
Proof. We begin by claiming that x∗2≤A. On the contrary, suppose that x∗2>A≥M. Then, due to (T2) and (T3), we have g(x∗2)<g(A)=f(M)<f(x∗2)=g(x∗2), which is a contradiction. The claim is proved.
Next, for a given ϕ∈C[x∗1,ˆG(x∗1)]∖{x∗1}, let xϵ:=xϵ(ϕ) be the solution of problem (1.1) when replacing f by f+ϵ. Since xϵ converges to x as ϵ tends to zero, we only need to prove that there exists T>0 such that xϵ(t)<A for all t≥T. To this end, let yϵ:=yϵ(ϕ) be the solution of problem (1.1), when replacing f by f+ϵ and g by g+ with g+(s)=maxσ∈[0,s]g(σ). By Theorem 2.1, we have xϵ(t)≤yϵ(t) for all t≥0, and thus, we only need to show that yϵ(t)<A for all t≥T. On the contrary, we suppose that yϵ(t)≥A for all t>0. Then, combining the equation of yϵ and the fact that g+(s)=g(M) for all s≥M, we obtain
y′ϵ(t)≤g(M)−f(A)−ϵ<0, |
which is a contradiction. Then, there exists T>0 such that yϵ(T)<A. We further claim that yϵ(t)<A for all t≥T. Otherwise, there exists t1>T such that yϵ(t1)=A and y′ϵ(t1)≥0. Substituting yϵ(t1) in Eq (1.1), we get
0≤y′ϵ(t1)=−f(yϵ(t1))−ϵ+∫τ0h(a)g+(yϵ(t1−a))da,<−f(A)+g(M)≤0, |
which is a contradiction. Consequently, by passing to the limit in ϵ, we obtain that x(t)≤A for all t≥T.
We focus now on the lower bound of x. First, define the function
g_(s)={g(s),for 0<s<ˉm,f(M),for ˉm<s≤A, | (3.6) |
where x∗1<ˉm<M, which is the constant satisfying g(ˉm)=f(M). Note that, due to (T2)–(T4) and condition (3.5), the function g_ is nondecreasing over (0,A) and satisfies
{g_(s)≤g(s),0≤s≤A,g_(s)>f(s),x∗1<s<M,g_(s)<f(s),M<s≤A. |
Let y(ϕ) be the solution of problem (1.1) when replacing g by g_. From Theorem 2.1, we have y(t;ϕ)≤x(t;ϕ) for all t>0, and from Theorem 3.1, we have limt→∞y(t;ϕ)=M. Consequently, lim inft→∞x(t)≥M. In addition, if, for all T>0, there exists (tn)n such that tn>T, y(tn)=M, ˉm<y(s)≤A for all 0<s≤tn and y′(tn)<0, then, for tn>T+τ,
y′(tn)=−f(M)+f(M)=0, |
which is a contradiction. The lemma is established.
Denote ˉf=f|[M,A] the restriction function of f over [M,A] and G(s):=ˉf−1(g(s)) for s∈[M,A]. Now, we are ready to state our main theorem related to x∗2≥M.
Theorem 3.7. Under the assumptions of Lemma 3.6, the positive equilibrium x∗2 of problem (1.1) is globally asymptotically stable, provided that one of the following conditions holds:
(H1) fg is a nondecreasing function on [M,A].
(H2) f+g is a nondecreasing function over [M,A].
(H3) (GoG)(s)s is a nonincreasing function over [M,x∗2],
(H4) (GoG)(s)s is a nonincreasing function over [x∗2,A],
Proof. Denote x∞:=lim inft→∞x(t) and x∞:=lim supt→∞x(t). First, suppose that either x∞≤x∗2 or x∞≥x∗2. For x∞≤x∗2, we introduce the following function:
g_(s)={minσ∈[s,x∗2]g(σ)forx∗1<s<x∗2,g(x∗2)forx∗2≤s≤A. |
Let y(ϕ) be the solution of problem (1.1) when replacing g by g_. Since g_(s)≤g(s) for all x∗1≤s≤x∗2, we have y(t)≤x(t) for all t>0. Further, in view of Theorem 3.1, we obtain
limt→∞y(t)=x∗2≤lim supt→∞x(t)=x∞≤x∗2. |
The local stability is obtained by using the same idea as in the proof of Theorem 2.6 in [31].
Now, for x∞≥x∗2, we introduce the function
ˉg(s)={minσ∈[s,x∗2]g(σ)forx∗1<s≤x∗2,maxσ∈[x∗2,s]g(σ)forx∗2<s≤A, | (3.7) |
and let y(ϕ) be the solution of problem (1.1) when replacing g by ˉg. As above, we have x(t)≤y(t) for all t>0 and y(t) converges to x∗2 as t goes to infinity. Next, we suppose that x∞<x∗2<x∞ and we prove that it is impossible. Indeed, according to Lemma 3.6, we know that, for every solution x of problem (1.1), we have M≤x∞≤x∞≤A.
Now, using the fluctuation method (see [28,32]), there exist two sequences tn→∞ and sn→∞ such that
limn→∞x(tn)=x∞, x′(tn)=0, ∀n≥1, |
and
limn→∞x(sn)=x∞, x′(sn)=0, ∀n≥1. |
Substituting x(tn) in problem (1.1), it follows that
0=−f(x(tn))+∫τ0h(a)g(x(tn−a))da. | (3.8) |
Since g is nonincreasing over [M,A], we obtain, by passing to the limit in Eq (3.8), that
f(x∞)≤g(x∞). | (3.9) |
Similarly, we obtain
f(x∞)≥g(x∞). | (3.10) |
Multiplying the expression (3.9) by g(x∞) and combining with inequality (3.10), we get
f(x∞)g(x∞)≤f(x∞)g(x∞). |
This fact, together with the hypothesis (H1), gives x∞≤x∞, which is a contradiction. In a similar way, we can conclude the contradiction for (H2). Now, suppose that (H3) holds. First, notice that G makes sense, that is, for all s∈[M,A], the range of g is contained in [ˉf(M),ˉf(A)] since ˉf is strictly increasing over [M,A]. In fact, for all s∈[M,A] and since g is non-increasing over [M,A], we have g(A)≤g(s)≤g(M). Now, using the fact that f(A)≥f(M), we show that
ˉf(M)≤g(s)≤ˉf(A)for alls∈[M,A]. |
Therefore, the function G is nonincreasing and maps [M,A] to [M,A]. In view of inequalities (3.9) and (3.10) and the monotonicity of ˉf, we arrive at
x∞≤G(x∞), | (3.11) |
and
x∞≥G(x∞), | (3.12) |
with G(s)=ˉf−1(g(s)). Now, applying the function G to inequalities (3.11) and (3.12), we find
x∞≥G(x∞)≥(GoG)(x∞), |
which gives
(GoG)(x∞)x∞≤1=(GoG)(x∗2)x∗2. | (3.13) |
Due to (H3), it ensures that x∗2≤x∞, which is impossible. Using the same arguments as above, we obtain a contradiction for (H4). The theorem is proved.
Remark 3.8. In the case where g(s)>g(A) for all s>M, the two above results hold true. In fact, it suffices to replace A in Lemma 3.6 and Theorem 3.7 by B defined in (T1).
For the tangential case where two positive equilibria x∗1 and x∗2 are equal, we have the following theorem
Theorem 3.9. Suppose that (T1)–(T3) hold. Suppose that, in addition to the trivial equilibrium, problem (1.1) has a unique positive equilibrium x∗1. Then
(i) for ϕ∈C+, there exists T>0 such that 0≤x(t)≤M for all t≥T.
(ii) ϕ∈C[x∗1,ˆG(x∗1)]∖{x∗1} implies that x∗1<x(t)≤M for all t≥T.
(iii) if ϕ∈C[x∗1,ˆG(x∗1)], then x∗1 attracts every solution of problem (1.1) and x∗1 is unstable.
Proof. The uniqueness of the positive equilibrium implies that x∗1≤M and g(x)<f(x) for all x≠x∗1. For (ⅰ), suppose that there exists t0>0 such that x(t)≥M for all t≥t0. Then, by substituting x in Eq (1.1), we get
x′(t)≤−f(M)+g(M)<0. |
This is impossible and then there exists T>0 such that x(T)<M. Next, if there exists t1>T such that x(t1)=M and x(t)≤M for all t≤t1, then
0≤x′(t1)≤−f(M)+g(M)<0. |
Consequently (ⅰ) holds. We argue as in the proof of Lemma 3.2 (ⅰ), to show (ⅱ). Concerning (ⅲ), we consider the following Lyapunov functional
V(ϕ)=∫ϕ(0)x∗1(g(s)−g(x∗1))ds+12∫τ0ψ(a)(g(ϕ(−a))−g(x∗1))2da, |
where ψ(a)=∫τah(σ)dσ. As in the proof of Theorem 3.1, the derivative of V along the solution of problem (1.1) gives
dV(xt)dt=−12∫τ0h(a)[g(x(t))−g(x(t−a))]2da+[g(x(t))−g(x∗1)][g(x(t))−f(x(t))],∀t>0. |
Since g(s)<f(s) for all s≠x∗1 and g(s)≥g(x∗1) for all s∈[x∗1,M], we have dV(xt)/dt≤0. By LaSalle invariance theorem, x∗1 attracts every solution x(ϕ) of problem (1.1) with ϕ∈C[x∗1,ˆG(x∗1)]. From Theorems 2.5 and 3.4, we easily show that x1 is unstable.
In this section, we apply our results to the following distributed delay differential equation:
x′(t)=−μx(t)+∫τ0h(a)kx2(t−a)1+2x3(t−a)da, for t≥0, | (4.1) |
where μ, k are positive constants. The variable x(t) stands for the maturated population at time t and τ>0 is the maximal maturation time of the species under consideration. h(a) is the maturity rate at age a. In this model, the death function f(x)=μx and the birth function g(x)=kx2/(1+2x3) reflect the so called Allee effect. Obviously, the functions f and g satisfy the assumptions (T1)–(T3) and g reaches the maximum value k/3 at the point M=1. The equilibria of Eq (4.1) satisfies the following equation:
μx=kx21+2x3. | (4.2) |
Analyzing Eq (4.2), we obtain the following proposition.
Proposition 4.1. Equation (4.1) has a trivial equilibrium x=0. In addition
(i) if μ>2133k, then Eq (4.1) has no positive equilibrium;
(ii) if μ=2133k, then Eq (4.1) has exactly one positive equilibrium x∗1;
(iii) if 0<μ<2133k, then Eq (4.1) has exactly two positive equilibria x∗1<x∗2. Moreover,
(iii)-a if k3<μ<2133k, then 0<x∗1<x∗2<1;
(iii)-b if 0<μ≤k3, then 0<x∗1<1≤x∗2.
Using Theorem 2.6, we obtain
Theorem 4.2. If μ>2133k, then the trivial equilibrium of Eq (4.1) is globally asymptotically stable for all ϕ∈C+.
For the case (ⅲ)-a in Proposition 4.1 we have the following result
Theorem 4.3. Suppose that k3<μ<2133k. Then
(i) The trivial equilibrium of Eq (4.1) is globally asymptotically stable for all ϕ∈C[0,x∗1]∖{x∗1}.
(ii) The positive equilibrium x∗1 is unstable and the positive equilibrium x∗2 is globally asymptotically stable for all ϕ∈C[x∗1,ˆG(x∗1)]∖{x∗1}, where ˆG(x∗1)∈[1,∞) satisfies g(ˆG(x∗1))=g(x∗1).
(iii) There exists two heteroclinic orbits X(1) and X(2) connecting 0 to x∗1 and x∗1 to x∗2, respectively.
Proof. (ⅰ) and (ⅱ) directly follow from Proposition 4.1, (ⅲ)-a and Theorems 2.5 and 3.4.
For (ⅲ), we follow the same proof as in Theorem 4.2 in [5]. See also [18]. For the sake of completeness, we rewrite it. Let K={x∗1}. Clearly, K is an isolated and unstable compact invariant set in C[0,x∗1] and C[x∗1,ˆG(x∗1)].
By applying Corollary 2.9 in [33] to Φ|R+×C[0,x∗1] and Φ|R+×C[x∗1,ˆG(x∗1)], respectively, there exist two pre-compact full orbits X(1):R→C[0,x∗1]∖{0} and X(2):R→C[x∗1,ˆG(x∗1)]∖{0} such that α(X1)=α(X2)=K. This together with statements (i) and (ii) gives ω(X(1))={0} and ω(X(2))={x∗2}. In other words, there exist two heteroclinic orbits X(1) and X(2), which connect 0 to x∗1 and x∗1 to x∗2, respectively. This completes the proof.
For the case (ⅲ)-b in Proposition 4.1, we first show that
f(A)≥g(M) with g(A)=f(M) and A≥M. | (4.3) |
Lemma 4.4. Suppose that f(s)=μs. Condition (4.3) holds if and only if M≤1μg(1μg(M)).
Proof. It follows that f(A)≥g(M) if and only if A≥f−1(g(M))≥M. Since g is a decreasing function over [M,∞), we have g(A)≤g(f−1(g(M)))=g(1μ(g(M))). Moreover, since g(A)=f(M), we have M≤1μg(1μg(M)). The lemma is proved.
Lemma 4.5. For Eq (4.1), condition (4.3) holds if and only if
0<μ≤k3. | (4.4) |
Proof. By a straightforward computation, we have f−1(x)=x/μ. For G(x)=g(x)/μ, we obtain
(GoG)(x)=x(2px6+px3)8x9+(12+2p)x6+6x3+1, |
where p=k3/μ3. By applying Lemma 4.4, it is readily to see that 1≤(GoG)(1) holds if and only if
1≤3p2p+27, |
and thus, 0<μ≤k/3.
Theorem 4.6. Suppose that 0<μ≤k3. Then, the positive equilibrium x∗2 of Eq (4.1) is globally asymptotically stable for all ϕ∈C[x∗1,ˆG(x∗1)]∖{x∗1}, where ˆG(x∗1)∈[1,∞) satisfies g(ˆG(x∗1))=g(x∗1).
Proof. Observe that, in view of Lemmas 4.4 and 4.5, all hypotheses of Lemma 3.6 are satisfied. Now, in order to apply Theorem 3.7, we only show that (GoG)(x)x is nonincreasing over [M,A]. Indeed, by a simple computation, we have
((GoG)(x)x)′=−48px14−48px11−6p2x8+12px5+3px2[8x9+(12+2p)x6+6x3+1]2, |
where p=k3/μ3. Finally, observe that
((GoG)(x)x)′<0, |
for x≥1. In this case, both of (H3) and (H4) in Theorem 3.7 hold. This completes the proof.
Finally, for the tangential case, we have
Theorem 4.7. Suppose that μ=2133k. Then
(i) The trivial equilibrium of Eq (4.1) is globally asymptotically stable for all ϕ∈C[0,x∗1]∖{x∗1}.
(ii) If ϕ∈C[x∗1,ˆG(x∗1)]∖{x∗1}, then the unique positive equilibrium x∗1 is unstable and attracts every solution of Eq (4.1).
Proof. The proof of this theorem follows immediately from Theorems 2.5 and 3.9.
In this section, we perform numerical simulation that supports our theoretical results. As in Section 4, we assume that
f(x)=μx,g(x)=kx21+2x3, |
and confirm the validity of Theorems 4.2, 4.3, 4.6 and 4.7. In what follows, we fix
τ=1,k=1,h(a)=e−(a−0.5τ)2×102∫τ0e−(σ−0.5τ)2×102dσ | (5.1) |
and change μ and ϕ. Note that 2133k≈0.42 and h is a Gaussian-like distribution as shown in Figure 1.
First, let μ=0.5. In this case, μ>2133k and thus, by Theorem 4.2, the trivial equilibrium is globally asymptotically stable. In fact, Figure 2 shows that x(t) converges to 0 as t increases for different initial data.
Next, let μ=0.37. In this case, k3<μ<2133k and x∗1≈0.43, x∗2≈0.89 and ˆG(x∗1)≈3.09 (Figure 3). Hence, by Theorem 4.3, we see that the trivial equilibrium is globally asymptotically stable for ϕ∈C[0,x∗1]∖{x∗1}, whereas the positive equilibrium x∗2 is so for ϕ∈C[x∗1,ˆG(x∗1)]∖{x∗1}. In fact, Figure 4 shows such a bistable situation. Moreover, heteroclinic orbits X(1) and X(2), which were stated in Theorem 4.3 (ⅲ), are shown in Figure 5.
Thirdly, let μ=0.27<k3≈0.33. In this case, we have x∗1≈0.28, x∗2≈1.2 and ˆG(x∗1)≈6.52 (Figure 6). Thus, by Theorem 4.6, we see that the positive equilibrium x∗2 is globally asymptotically stable for ϕ∈C[x∗1,ˆG(x∗1)]∖{x∗1}. In fact, Figure 7 shows that x(t) converges to x∗2 as t increases for different initial data.
Finally, let μ=2133k≈0.42. In this case, we have x∗1≈0.63 and ˆG(x∗1)≈1.72 (Figure 8). By Theorem 4.7, we see that the trivial equilibrium is globally asymptotically stable for ϕ∈C[0,x∗1]∖{x∗1}, whereas x∗1 is unstable and attracts all solutions for ϕ∈C[x∗1,ˆG(x∗1)]∖{x∗1}. In fact, Figure 9 shows such two situations.
In this paper, we studied the bistable nonlinearity problem for a general class of functional differential equations with distributed delay, which includes many mathematical models in biology and ecology. In contrast to the previous work [5], we considered both cases x∗2<M and x∗2≥M, and obtained sufficient conditions for the global asymptotic stability of each equilibrium. The general results were applied to a model with Allee effect in Section 4, and numerical simulation was performed in Section 5. It should be pointed out that our theoretical results are robust for the variation of the form of the distribution function h. This might suggest us that the distributed delay is not essential for the dynamical system of our model. We conjecture that our results still hold for τ=∞, and we leave it for a future study. Extension of our results to a reaction-diffusion equation could also be an interesting future problem.
We deeply appreciate the editor and the anonymous reviewers for their helpful comments to the earlier version of our manuscript. The first author is partially supported by the JSPS Grant-in-Aid for Early-Career Scientists (No.19K14594). The second author is partially supported by the DGRSDT, ALGERIA.
All authors declare no conflicts of interest in this paper.
[1] | Johnny's Selected Seeds. Strawberry. 2017, Available from: http: //www.johnnyseeds.com/c-247-strawberries.aspx?source=W_fruit_ddcat_092016 |
[2] | Bentvelsen GC, Bouw B, Veldhuyzen E, et al. (1996) Breeding strawberries (Fragaria ananassa Duch.) from seed. Acta Hortic 439: 149-153. |
[3] | Bentvelsen GC, Bouw B (2006) Breeding ornamental strawberries. Acta Hortic 708: 455-457. |
[4] | Bentvelsen GC, Bouw B (2002) Breeding strawberry F1-hybrids for vitamin C and sugar content. Acta Hortic 567: 813-814. |
[5] |
Durner EF (2016) Enhanced Flowering of The F1 Long-day Strawberry Cultivar 'Elan' via Nitrogen and Daylength Manipulation. AIMS Agric Food 1: 4-19. doi: 10.3934/agrfood.2016.1.4
![]() |
[6] |
Heide OM, Stavang JA, Sonsteby A (2013) Physiology and genetics of flowering in cultivated and wild strawberries – a review. J Hortic Sci Biotechnol 88: 1-18. doi: 10.1080/14620316.2013.11512930
![]() |
[7] | Larson KD (1994) Strawberry. In: Handbook of environmental physiology of fruit crops. Volume I. Eds. B Schaffer and P.C. Anderson. CRC Press, Boca Raton, FL. 271-297. |
[8] | Durner EF, Barden JA, Himelrick DG, et al. (1984) Photoperiod and temperature effects on flower and runner development in day neutral, Junebearing and everbearing strawberries. J Amer Soc Hort Sci 109: 396 400. |
[9] |
Sonsteby A, Heide OM (2007) Quantitative long-day flowering response in the perpetual-flowering F1 strawberry cultivar 'Elan'. J Hortic Sci Biotechnol 82: 266-274. doi: 10.1080/14620316.2007.11512228
![]() |
[10] |
Sonsteby A, Heide OM (2007) Long-day control of flowering in everbearing strawberries. J Hortic Sci Biotechnol 82: 875-884. doi: 10.1080/14620316.2007.11512321
![]() |
[11] |
Durner EF (2017) Enhanced flowering of the F1 long-day strawberry cultivars 'Tarpan' and 'Gasana' with nitrogen and daylength management. AIMS Agric Food 2: 1-15. doi: 10.3934/agrfood.2017.1.1
![]() |
[12] |
Sonsteby A, Opstad N, Myrheim U, et al. (2009) Interaction of short day and timing of nitrogen fertilization on growth and flowering of 'Korona' strawberry (Fragaria x ananassa Duch.). Sci Hortic-Amsterdam 123: 204-209. doi: 10.1016/j.scienta.2009.08.009
![]() |
[13] | Desmet EM, Verbraeken L, Baets W (2009) Optimisation of nitrogen fertilization prior to and during flowering process on performance of short day strawberry 'Elsanta'. Acta Hortic 842: 675-678. |
[14] | Yamasaki A, Yano T (2009) Effect of supplemental application of fertilizers on flower bud initiation and development of strawberry – possible role of nitrogen. Acta Hortic 842: 765-768. |
[15] |
Sonsteby A, Opstad N, Heide OM (2013) Environmental manipulation for establishing high yield potential of strawberry forcing plants. Sci Hortic-Amsterdam 157: 65-73. doi: 10.1016/j.scienta.2013.04.014
![]() |
[16] |
Yamasaki A (2013) Recent progress if strawberry year-round production technology in Japan. Jpn Agric Res Q 47: 37-42. doi: 10.6090/jarq.47.37
![]() |
[17] | Lieten F (2002) The effect of nutrition prior to and during flower differentiation on phyllody and plant performance of short day strawberry 'Elsanta'. Acta Hortic 567: 345-348. |
[18] | Guttridge CG (1985) Fragaria x ananassa. In: Halevy, A.H. (Ed.), Handbook of Flowering, vol 3. CRC Press, Boca Raton, FL. 16-33. |
[19] | Mochizuki T (1995) Past and present strawberry breeding programs in Japan. Adv Strawb Res 14: 9-17. |
[20] | Mochizuki T, Yoshida Y, Yanagi T, et al. (2009) Forcing culture of strawberry in Japan - production technology and cultivars. Acta Hortic 842: 107-110. |
[21] | Van den Muijzenberg EWB (1942) De invloed van licht en temperatuur op de periodieke ontwikkeling van de aardbei en de beteekenis daarvan voor de teelt [The influence of light and temperature on the periodic development of the strawberry and its significance in cultivation]. Ph.D. thesis, Laboratorium voor Tuinbouwplantenteelt, Wageningen, the Netherlands. 160. |
[22] | Furuya S, Yamashita M, Yamasaki A (1988) Effects of nitrogen content on the flower bud initiation induced by chilling under dark condition in strawberries. Bulletin of the National Research Institute of Vegetables, Ornamental Plants and Tea. Series D. Kurume (Japan). |
[23] | Matsumoto O (1991) Studies on the control of flower initiation and dormancy in the cultivation of strawberry, Fragaria X ananassa Duch. Spec Bullet Yamaguchi Agric Exp Stn 31: 1102. |
[24] |
Neri D, Baruzzi G, Massetani F, et al. (2012) Strawberry production in forced and protected culture in Europe as a response to climate change. Can J Plant Sci 92: 1021-1036. doi: 10.4141/cjps2011-276
![]() |
[25] | Yamasaki A, Yoneyama T, Tanaka F, et al. (2002) Tracer studies on the allocation of carbon and nitrogen during flower induction of strawberry plants as affected by the nitrogen level. Acta Hortic 567: 349-352. |
[26] | Strik BC (1985) Flower bud initiation in strawberry cultivars. Fruit Var J 39: 59. |
[27] | Yoshida Y (1992) Studies on flower and fruit development in strawberry with special reference to fruit malformation in 'Ai-Berry'. Memo Fac Agric Kagawa Univ 57: 194. |
[28] |
Barthelemy D, Caraglio Y (2007) Plant architecture: a dynamic, multilevel and comprehensive approach to plant form, structure and ontogeny. Ann Bot 99: 375-407. doi: 10.1093/aob/mcl260
![]() |
[29] | Savini G, Neri D (2004) Strawberry architectural model. Acta Hortic 649: 169-176. |
[30] | Melis P, Flower bud analysis of strawberry plants: predicting flower trusses and their spreading potential. 2013, Available from: http://www.hoogstraten.eu/congress/data/scientific/Predicting%20potential%20flower%20trusses%20and%20their%20spread%20through%20flower%20bud%20analysis%20of%20strawberry%20plants.pdf |
[31] | Gianluca S, Letouze A, Sabbadini C, et al. (2006) Evaluation of tray-plant quality in the propagation phase. Acta Hortic 708: 231-236. |
[32] | Bosca JP, Neri D, Massetani F, et al. (2012) Relationship between plant architecture and fruit production of the short-day strawberry cultivar Gariguette. J Berry Res 2: 105-111. |
[33] | Wobbrock JO, Findlater L, Gergle D, et al. (2011) The aligned rank transform for nonparametric factorial analyses using only anova procedures. In Proceedings of the SIGCHI Conference on Human Factors in Computing Systems (pp. 143-146). ACM. |
[34] | Durner EF (2016) Photoperiod and Temperature Conditioning of 'Sweet Charlie' Strawberry (Fragaria X ananassa Duch.) Plugs Enhances Off-Season Production. Sci Hortic-Amsterdam 201: 184-189. |
[35] |
van Delm T, Melis P, Stoffels K, et al. (2013) Pre-harvest night-interruption on everbearing cultivars in out-of-soil strawberry cultivation in Belgium. Int J Fruit Sci 13: 217-226. doi: 10.1080/15538362.2012.698176
![]() |
[36] | Le Miere P, Hadley P, Darby J, et al. (1996) The effect of temperature and photoperiod on the rate of flower initiation and the onset of dormancy in strawberry (Fragaria x ananassa Duch.). J Hortic Sci 71: 261-2711. |
[37] |
Durner EF, Poling EB (1987) Flower bud induction, initiation, differentiation and development in the 'Earliglow' strawberry. Sci Hortic-Amsterdam 31: 61-69. doi: 10.1016/0304-4238(87)90107-5
![]() |
[38] | Hytonen T, Palonen P, Mouhu K, et al. (2004) Crown branching and cropping potential in strawberry (Fragaria x ananassa Duch.) can be enhanced by daylength treatments. J Hortic Sci Biotechnol 79: 466-471. |
[39] | Anderson HM, Guttridge CG (1982) Strawberry truss morphology and the fate of high-order flower buds. Crop Res 22: 105-122. |
[40] | Fujimoto K, Kimura M (1970) Studies on flowering of strawberry. III. Effect of nitrogen on flower bud differentiation and development. Abstracts of the Japanese Society for Horticultural Science Spring Meeting 174-175 (In Japanese). |
1. | Jampala Annie Modestra, Gokuladoss Velvizhi, Kamaja Vamshi Krishna, Kotakonda Arunasri, Piet N. L. Lens, YarlagaddaVenkata Nancharaiah, S. Venkata Mohan, 2017, Chapter 6, 978-3-319-58621-2, 165, 10.1007/978-3-319-58622-9_6 | |
2. | Hai The Pham, Biosensors based on lithotrophic microbial fuel cells in relation to heterotrophic counterparts: research progress, challenges, and opportunities, 2018, 4, 2471-1888, 567, 10.3934/microbiol.2018.3.567 | |
3. | Nan Xiao, P. Ravi Selvaganapathy, Rong Wu, Jinhui Jeanne Huang, Influence of wastewater microbial community on the performance of miniaturized microbial fuel cell biosensor, 2020, 302, 09608524, 122777, 10.1016/j.biortech.2020.122777 | |
4. | D. Revelo-Romo, M. Guerrero-Flórez, A. Ordóñez, I. A. Sánchez-Ortiz, N. Pusapaz-Villota, O. C. Yela, L. A. Galeano, Bacterial diversity of leachates retained in adsorbents regenerated by wet catalytic peroxide oxidation: potential driving bioelectrochemical systems, 2021, 1735-1472, 10.1007/s13762-020-03058-4 | |
5. | Huang Yao, Jialong Xiao, Xinhua Tang, Microbial Fuel Cell-Based Organic Matter Sensors: Principles, Structures and Applications, 2023, 10, 2306-5354, 886, 10.3390/bioengineering10080886 | |
6. | Maira Anam, Sameen Yousaf, Iqra Sharafat, Zargona Zafar, Kamran Ayaz, Naeem Ali, Comparing Natural and Artificially Designed Bacterial Consortia as Biosensing Elements for Rapid Non-Specific Detection of Organic Pollutant through Microbial Fuel Cell, 2017, 12, 14523981, 2836, 10.20964/2017.04.49 | |
7. | Mingyang Lai, Jiteng Hong, Xiaolong Xu, Yuying Li, Changyu Liu, Jianbo Jia, Tiangang Luan, The effect of inoculum source of different pollution levels on the performances of microbial fuel cell biosensors, 2025, 422, 09254005, 136614, 10.1016/j.snb.2024.136614 |