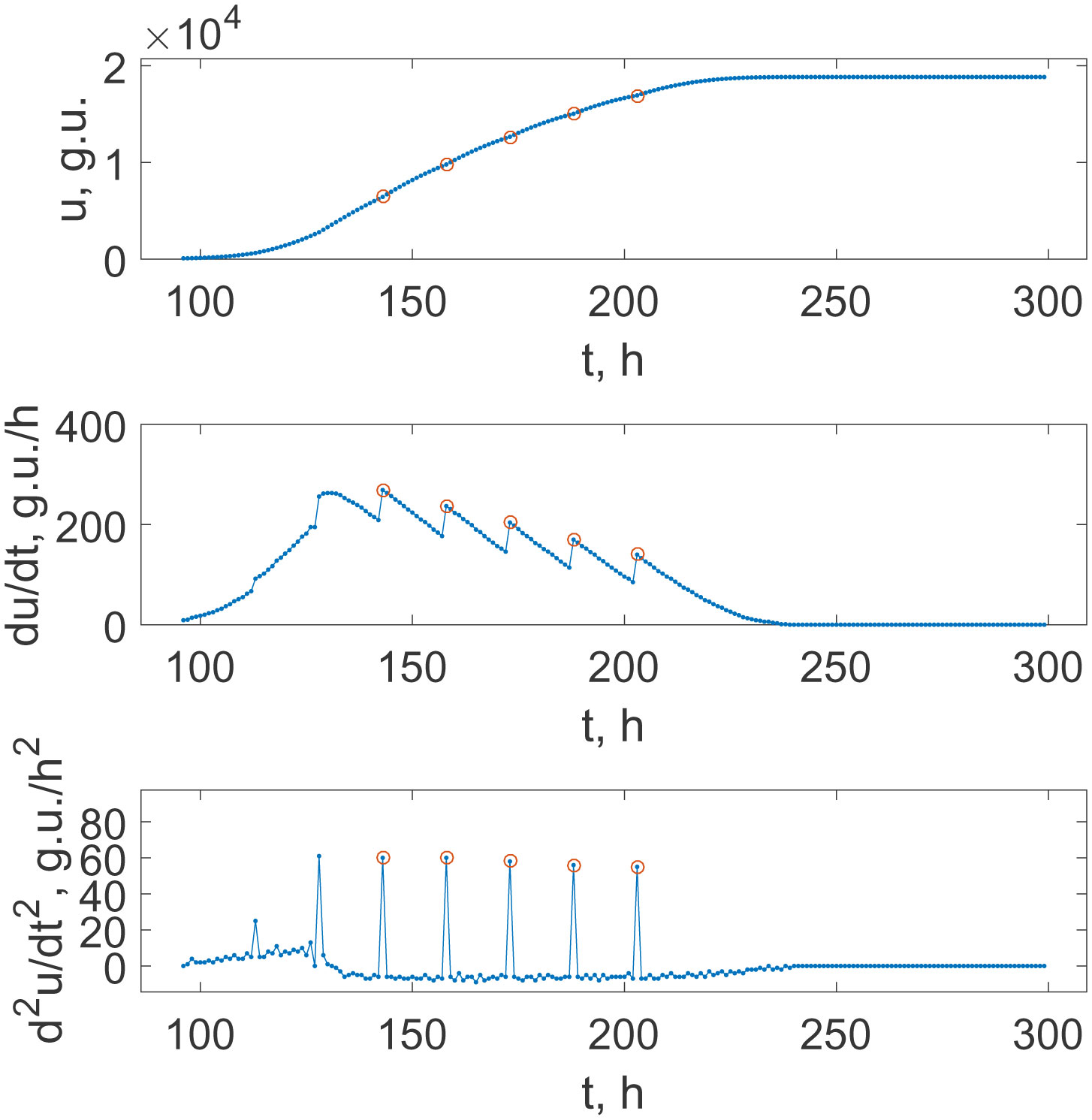
Zeolitic imidazole framework-8 (ZIF-8) represents a notable subtype of metal-organic frameworks (MOFs), characterized by tetrahedral and zeolite-like structures interconnected through Imidazolate anions. ZIF-8's outstanding attributes, including its expansive intra-crystalline surface area and robust chemical and thermal stability, have positioned it as a promising contender for carbon dioxide (CO2) capture applications. The application of ZIF-8 in the membrane and composite fields involves utilizing ZIF-8 in the development and enhancement of membranes and composite materials for gas separation, catalysis, and sensing. This article serves as a comprehensive exploration of contemporary CO2 capture technologies, elucidating their respective merits and demerits. Moreover, the review offers insights into the prevailing CO2 adsorption techniques implemented across industries. Delving into ZIF-8 synthesis methods, the discourse encompasses diverse synthetic pathways. Experimental evidence, furnished through X-Ray diffraction patterns and scanning electron microscopy, validates ZIF-8's structure-activity correlation and morphological characteristics. We extend this review to encapsulate the parameters governing CO2 adsorption by ZIF-8, delineating the key factors influencing its capture efficacy. Notably, we encompass CO2 measurement protocols and techniques specific to ZIF-8. Additionally, we appraise the CO2 adsorption potential of ZIF-8 within various composite and filter systems composed of distinct ZIFs. Culminating with an emphasis on ZIF-8's exceptional advantages for CO2 capture, this review serves as a repository of insights into the unparalleled potential of ZIF-8 as a foundational material. Providing a succinct yet comprehensive overview, this article facilitates a rapid understanding of ZIF-8's transformative role in the realm of CO2 capture.
Citation: Angaraj Singh, Ajitanshu Vedrtnam, Kishor Kalauni, Aman Singh, Magdalena Wdowin. Synthesis routes of zeolitic imidazolate framework-8 for CO2 capture: A review[J]. AIMS Materials Science, 2025, 12(1): 118-164. doi: 10.3934/matersci.2025009
[1] | George O Lugonzo, Ezekiel M. Njeru, William Songock, Albert A. Okumu, Eric M. Ndombi . Epidemiology of multi-drug resistant Tuberculosis in the western region of Kenya. AIMS Microbiology, 2024, 10(2): 273-287. doi: 10.3934/microbiol.2024014 |
[2] | Sabrine Balti, Yassine Mabrouk, Mouna Souihi, Imen Hemissi, Ismail Amri, Ethan Humm, Noor Khan, Ann M. Hirsch . Combined inoculation of rhizobacteria with Mesorhizobium promotes growth, nutrient contents, and protects chickpea against Fusarium redolens. AIMS Microbiology, 2025, 11(2): 318-337. doi: 10.3934/microbiol.2025015 |
[3] | Hripsime Petrosyan, Karen Trchounian . Growth characteristics, redox potential changes and proton motive force generation in Thermus scotoductus K1 during growth on various carbon sources. AIMS Microbiology, 2024, 10(4): 1052-1067. doi: 10.3934/microbiol.2024045 |
[4] | Napapohn Kajadpai, Jirameth Angchuan, Pannida Khunnamwong, Nantana Srisuk . Diversity of duckweed (Lemnaceae) associated yeasts and their plant growth promoting characteristics. AIMS Microbiology, 2023, 9(3): 486-517. doi: 10.3934/microbiol.2023026 |
[5] | Mohammed M. M. Abdelrahem, Mohamed E. Abouelela, Nageh F. Abo-Dahab, Abdallah M. A. Hassane . Aspergillus-Penicillium co-culture: An investigation of bioagents for controlling Fusarium proliferatum-induced basal rot in onion. AIMS Microbiology, 2024, 10(4): 1024-1051. doi: 10.3934/microbiol.2024044 |
[6] | Alaa Fathalla, Amal Abd el-mageed . Salt tolerance enhancement Of wheat (Triticum Asativium L) genotypes by selected plant growth promoting bacteria. AIMS Microbiology, 2020, 6(3): 250-271. doi: 10.3934/microbiol.2020016 |
[7] | Yuya Uehara, Yuko Shimamura, Chika Takemura, Shiori Suzuki, Shuichi Masuda . Effects of cosmetic ingredients on growth and virulence factor expression in Staphylococcus aureus: a comparison between culture medium and in vitro skin model medium. AIMS Microbiology, 2025, 11(1): 22-39. doi: 10.3934/microbiol.2025002 |
[8] | Amira ElBaradei, Dalia Ali Maharem, Ola Kader, Mustafa Kareem Ghareeb, Iman S. Naga . Fecal carriage of ESBL-producing Escherichia coli in Egyptian patients admitted to the Medical Research Institute hospital, Alexandria University. AIMS Microbiology, 2020, 6(4): 422-433. doi: 10.3934/microbiol.2020025 |
[9] | Jorge Barriuso . Quorum sensing mechanisms in fungi. AIMS Microbiology, 2015, 1(1): 37-47. doi: 10.3934/microbiol.2015.1.37 |
[10] | Ogueri Nwaiwu, Chiugo Claret Aduba . An in silico analysis of acquired antimicrobial resistance genes in Aeromonas plasmids. AIMS Microbiology, 2020, 6(1): 75-91. doi: 10.3934/microbiol.2020005 |
Zeolitic imidazole framework-8 (ZIF-8) represents a notable subtype of metal-organic frameworks (MOFs), characterized by tetrahedral and zeolite-like structures interconnected through Imidazolate anions. ZIF-8's outstanding attributes, including its expansive intra-crystalline surface area and robust chemical and thermal stability, have positioned it as a promising contender for carbon dioxide (CO2) capture applications. The application of ZIF-8 in the membrane and composite fields involves utilizing ZIF-8 in the development and enhancement of membranes and composite materials for gas separation, catalysis, and sensing. This article serves as a comprehensive exploration of contemporary CO2 capture technologies, elucidating their respective merits and demerits. Moreover, the review offers insights into the prevailing CO2 adsorption techniques implemented across industries. Delving into ZIF-8 synthesis methods, the discourse encompasses diverse synthetic pathways. Experimental evidence, furnished through X-Ray diffraction patterns and scanning electron microscopy, validates ZIF-8's structure-activity correlation and morphological characteristics. We extend this review to encapsulate the parameters governing CO2 adsorption by ZIF-8, delineating the key factors influencing its capture efficacy. Notably, we encompass CO2 measurement protocols and techniques specific to ZIF-8. Additionally, we appraise the CO2 adsorption potential of ZIF-8 within various composite and filter systems composed of distinct ZIFs. Culminating with an emphasis on ZIF-8's exceptional advantages for CO2 capture, this review serves as a repository of insights into the unparalleled potential of ZIF-8 as a foundational material. Providing a succinct yet comprehensive overview, this article facilitates a rapid understanding of ZIF-8's transformative role in the realm of CO2 capture.
Biophysical methods focused on the rapid and accurate quantification of bacterial growth and its response to antibacterial drugs have recently attracted significant attention [1], [2]. Among these methods, the approaches addressing the optical indication of chemical reactions play an important role [3]–[5] because of their non-invasive character, reporting about biochemical content changes due to the metabolism of cells during their growth and proliferation. Therewith, such approaches take into account an amount of viable micro-organisms only that is impossible, say, for methods, which use the optical density measurements.
One of the most reliable processes for such studies is the bacterial respiration. The search for methods, which correlate the oxygen content in a medium, where bacteria grow, and characteristics of their vital activity was started at the dawn of quantitative experimental microbiology [6] and is continuing up to present, including such modern challenges as studying drug susceptibility and resistance [7]. Such an approach is based on two reasons: i) Oxygen consumption by breathing is a direct marker of different metabolic processes in living and dividing bacteria [8], [9]; ii) a dynamical change of the concentration of oxygen dissolved in a liquid medium can be accurately and effectively detected, registered and quantified by methods of analytical chemistry. It is possible due to the existence of a variety of neutral chemicals with photo-physical properties that drastically change in response to the content of oxygen dissolved in the medium under investigation. This approach finds applications to a wide range of systems: from aerodynamic flows and combustion problems to biological processes [10]–[12].
In particular, one of the most powerful methods, introduced about two decades ago [13]–[15] and widespread now as a standard in the field of investigations related to the bacterial cultures of M. tuberculosis, is the BACTEC MGIT 960 system. Although it operates with an indirect indication of the growth process, there exists a proportionality of the indicated growth units to the viable bacterial population; see e.g. [16], [17].
Its principle of operation can be summarized as follows: The Mycobacteria Growth Indicator Tube (MGIT) contains 7 mL of Middlebrook 7H9 medium supporting the growth of mycobacteria and silicon rubber impregnated with Tris(4,7-diphenyl-1,10-phenanthroline)ruthenium(II) dichloride complex, which is a fluorescence quenching based oxygen sensor. An activity of this fluorochrome is quenched by oxygen dissolved in the liquid broth. During the bacterial growth, the dissolved oxygen is consumed and, subsequantly, replaced with carbon dioxide. As a result, the fluorochrome inhibition by free oxygen gradually diminishes, and its fluorescence in response to the ultraviolet illuminations can be detected by the BACTEC's sensor. This procedure is carried out by the BACTEC MGIT 960 in an automated regime with the time step of one hour. However, the BACTEC MGIT 960 is used in conventional studies for a binary decision only: whether the fluorescence intensity overcomes some empirically prescribed boundary (this indicates a growing culture) or not (this is interpreted as a non-growing culture). In addition, the time required to cross the boundary mentioned can be taken into account.
At the same time, the BACTEC MGIT 960 can provide much more information, which can be analyzed and potentially may give important biophysical and biochemical insights, if one takes into account the rich data series with a fine time resolution. In particular, the promising target is the detailed study of Mycobacterium tuberculosis culture growth under conditions which lead to synchronous cell division. Note that this phenomenon for different bacteria, including M. tuberculosis, was detected by the counts of numbers of viable units (either cells or colonies) in earlier works [18], [19]. It exhibits itself as the sequential jumps in this quantity separated by the stable fixed time intervals that reflect simultaneous divisions. Later, it was discussed that the cell division is regulated by time but not the cell size [20], and the minimal doubling time is equal to 14.7 h in batch culture for the standard reference strain H37Rv [21].
The analysis of the full curve of the respiration-induced fluorescence supplied with “a marriage” of mathematical models of analytical chemistry addressing oxygen-quenched chemical reactions [12], [22], [23] and biophysical models of bacterial dynamics can unravel detailed vital processes of bacterial growth. Thus, the goal of our work is to understand when and under which conditions the synchronization regime occurs and how the bacterial population physiologically behaves within the inter-division time intervals. Another question is whether it is possible to reveal a correspondence and transitions between such regimes and typical bacterial growth curves (e.g., Verhulst's and Gompertz's) well-established for typical smooth population growth with saturation.
All data on fluorescence curves, which will be discussed below, were obtained with BACTEC MGIT 960 (Becton Dickinson) for the case of the standard strain H37Rv of M. tuberculosis growing under conditions of the standard protocol [24], [25].
The initial inoculum further refereed to as “Dilution 1” is estimated as 7.5 × 106 cells/mL. The control of the growth positivity corresponded to the instrument's indicator.
For further processing, the default output of the apparatus, which allows exporting recorded data in the format of Excel spreadsheets, was used. The program code operating with the default structure of such set-up-generated files for reading time series and their subsequent fitting can be found online at https://github.com/postnicov/readprocbactec
The general shape, magnitude, and time duration of the recordings do not contain any peculiarities. However, as was mentioned in the Introduction, the standard interpretation of such data is threshold-based and does not address the detailed time dynamics of these curves' shape evolution completely. On the contrary, the principal point of our interest and the main message of this section is “what new can be found and interpreted in the old-known curves when processing them with specially constructed methods.”
An additional test of the revealed dynamical peculiarities (see Discussion for details) was carried out using the resazurin reduction assay [26], [27], and quantitative growth curves were obtained photometrically using a portable colorimetric analyzer [28].
The BACTEC fluorescence growth curves, which indicate the growth of the bacterial population, look like a kind of logistic curves at large scales and are often not perfectly smooth, as they comprise periodically repeated concave sub-intervals. We hypothesize that such a behaviour is a possible direct consequence of synchronization processes, previous qualitative indication of which were mentioned above. Note that the high-frequency (with the time step of 1 hour) continuous monitoring of the oxygen-quenching fluorescence opens sufficiently wider opportunities for a more detailed study. To reveal this property precisely, we analyze not only the growth curves themselves but also their first and second derivatives (respectively, to the unit time step Δt = 1 h), represented via the first
and the second
finite differences , which resemble the local growth speed and acceleration measured in g.u. · h−1 and g.u. · h−2, respectively.
Figures 1–3 illustrate this strategy for bacterial cultures prepared with different initial dilutions. Figure 1 relates to the case of the standard protocol of mycobacterial growth detection [25]. One can see that the growth is already non-monotonous, and, after some transient regime, the growth curve is subdivided into a stable periodic sequence of growth processes till the last stage leading to the saturated value.
Each periodically repeated stage is characterized by a jump-like initiation of the growth, which is followed by the practically linear decay of the growth speed, as is seen in Figure 1 (middle panel). The localization of these jumps can be easily performed by considering the second finite difference (3.2), which demonstrates a set of outliers, see Figure 1 (lower panel) where the corresponding points are highlighted by markers. The same markers subdivide the original BACTEC signal curve in Figure 1 (upper panel).
This procedure may be motivated as follows. The function u(t) is continuous but has salient points, where the derivative du/dt has breaks. Respectively, the derivatives of such discontinuous function d2u/dt2 = d/dt(du/dt) are equal to the Dirac delta-functions for the case of ideal continuous functions u(t) and sufficiently large values for the case of discrete samples. Since the experimental records u(tn) are sampled equispaced with respect to time, Eq (3.2) resembles such a second derivative.
One can see that the time distances between these outliers in the growth regime, which starts after some transient time and continues up to the last stage of the growth before the saturation, are quite stable and equal to Td = 15 hours for all curves in Figure 1. The absolute error of this quantity determination is ΔTd = 1 hours; that follows from the frequency of data measurements. Note that this value is quite close to the value of M. tuberculosis duplication time under conditions most favoured for the culture growth. Thus, we can conclude that this feature of the registered fluorescence curve argues in favour of the conclusion that bacteria in the culture are in the regime of synchronized growth and division.
Since synchronization as a rule requires a sufficiently dense packing of micro-organisms that makes available quorum sensing, the experiments were repeated with the initial seed culture under different degrees of dilution. Figure 2 demonstrates the growth situation for 102 times diluted initial seed. One can see that these conditions result in a generally smooth curve, and the clearly expressed staged periodicity vanishes, which is confirmed by the plots of the first and second derivatives. Figure 2 (middle panel) does not demonstrate a saw-like pattern, and the data in Figure 2 (lower panel) do not exhibit significant point-wise jumps regularly exceeding those fluctuations along the whole curves, which are consequences of the non-smoothed finite difference procedure applied to the realistic record. Although some of the largest peaks in this plot of the second derivative may be leftovers of the sequential jumps, their magnitude does not significantly exceed the background noise (in contrast to Figure 1, where such a difference reaches almost two orders of magnitude). Therefore, it is not reasonable to explore them in detail.
The qualitatively same picture of a smooth growth is seen in the case of the 104 times dilution of the initial seed culture, too; see Figure 3. Moreover, the plot of the second derivative in Figure 3 (lower panel) is more uniform in the magnitudes of fluctuations. They are associated with the equipment noise and leave no room for the staged growth process completely.
Thus, we can conclude that the emergence of periodic time-limited stages in the growth curve indicated by the overall fluorescence is connected with the density of the growing bacterial culture. The next step of the analysis was the quantifying these qualitatively distinct processes and associating them to the specificity of chemical reactions.
First of all, let us consider the case corresponding to Figure 1. Due to the non-monotonous character of the growth curve u(t), where we identify breaking points of the first derivative localized at the time moments tb as the moments of synchronized cells divisions, we fit these points only at the first step. As it is shown in Figure 4 (upper panel), the points follow a straight line when the following special transformation is applied:
where K = max(u(t)) is the saturated value of complete sigmoidal growth curve, and r and tm are positive constants whose meanings will be explained below.
The dashed curve in Figure 4 (lower panel) shows the function
obtained from Eq (3.3) and plotted as a continuous dependence on time but with the same parameters as in the discrete version (3.3). It is an explicit solution of the Verhulst equation, i.e., it defines the standard population growth limited by the carrying capacity of the environment with respect to the population size. Therefore, r is the population growth rate, and tm is the time of inflection, i.e., the moment when the population size reaches one half of the maximal (saturated) value K. The dashed curve (Figure 4, (lower panel)) accurately goes through the circles but deviates from the dotted curve denoting the fluorescent signal between and after them (and does not have sense before the first circle). Thus, the moments denoted by circles can be already associated with the population multiplication, while the remaining intervals originates from the specificity of chemical reactions governing the oxygen-quenched fluorescence that may be connected with the oxygen consumption by breathing bacteria.
The next case is the bacterial culture growth from 102 times diluted seed. Figure 5 (upper panel) demonstrates the plot with the same representation by Eq (3.3) but now for the entire sequence of time moments t instead of a limited array of tb, since the curve is completely smooth, as shown in Figure 2. It should be noted that, for the sake of visibility, ×'s in Figure 5 are separated by the time intervals of 5 hours, but the actual computations did all use 1 hour-separated data points. One can see that that the middle part of the full curve in such representation follows the straight line. Moreover, the curve given by Eq (3.4) and shown as the dashed curve in Figure 5 (lower panel) practically coincides over the whole time region, and the deviation is small even in the initial and final regions, where the experimental markers in Figure 5 (upper panel) deviates from the fitting straight line.
At the same time, the data within the initial transient range can be approximated with another dependence in the form
(see Figure 5 (middle panel)), which defines Gompertz's sigmoidal growth
which is shown as the dash-dotted line in Figure 5 (lower panel). Note that it reproduces the experimental recordings better than Verhulst's within the initial stage of growth only and then drastically deviates from the actual data. This can be explained by the fact that the cells in the initially diluted bacterial suspension are not synchronized with respect to their divisions yet. When this synchronization occurs, the growth dynamics changes to the logistic one, as for the cells' numerical multiplication points in Figure 4.
However, when taking two orders more diluted initial suspension, then this asynchronous regime expands for more time, practically up to saturation; see the experimental data and their fitting by the function (3.5) in Figure 6 (upper panel). The respective analytical growth curve, the dash-dotted line in Figure 6 (lower panel), is practically indistinguishable from the recorded BACTEC's curve.
Table 1 lists the numerical parameters r, tm, and K included into Verhulst's and Gompertz's functions, (3.4) and (3.9), respectively.
One can see that both cases of the Verhulst growth curves, either with the clearly expressed jumps indicating the synchronization of divisions or without such jumps for more diluted culture, give the doubling times Td = ln/r equal, respectively, to Td = 14.9 h and 14.1 h, which completely agreed with the inter-jumps time intervals in Figure 1 equal to Td = (15 ± 1) h, taking into account the instrumental and fitting uncertainties. Thus, this confirms the conclusion that these jumps already correspond to the synchronized proliferation of bacteria.
On the other hand, the Gompertz's growth parameters revealed for the initial growth stage at the moderate (102 times) dilution and for the full time of the process for the high (104 times) dilution are also very close to each other. This result argues in favour of the conclusion that the Gompertz law of growth reflects the bacterial population's growth in the case when the culture density is too small to realize the synchronized duplication of M. tuberculosis in the whole community.
Initial dilution | r/h−1 | tm/h | K/g.u. |
1 | 0.0466 | 157.1 | 18831 |
102 | 0.049* | 265.7* | 23242 |
102 | 0.022** | 261.0** | 23242 |
104 | 0.021 | 347.3 | 9881 |
* for the Verhulst growth law component
** for the Gompertz growth law component
As is revealed by the analysis of growth curves in Figures 4–6, different dilutions of the initial seed culture leads to different growth laws. The high density of cells results in the growth curve (3.4), which resembles the logistic growth governed by the Verhulst equation
if we consider time moments, when the fluorescence value corresponds to the moments of cell number multiplications.
At the same time, Eq (3.4) resembles the Stern-Volmer law for the fluorescence signal records under conditions of the oxygen-quenching kinetics
Here, we take into account that the signal u itself is the fluorescence signal recorded by the BACTEC system as a response to the diminishing oxygen quenching by oxygen dissolved in the medium. Comparing Eq (3.8) and Eq 3.4, we can conclude that the quenching oxygen density decays exponentially with time: Q(t) ~ exp(−rt). For the case of concentrated bacterial suspensions (Figure 4), this condition is fulfilled accurately at the points of the population's multiplication; for the less concentrated suspensions (Figure 6), it is the case for practically the whole time interval. Such a decaying dependence seems to be natural from the biophysical and chemical points of view, since vital bacteria already consume oxygen while breathing.
At the same time, Eq (3.7) implies that the growth rate of the multiplicative growth du/dt = R(u)u is limited, i.e., the per capita growth rate is a function R = r(1 – u/K) of the local cell density u, and stops when the local carrying capacity is reached. Thus, we can conclude that the growth is limited primarily by the organisms' packing reasons, not by the deficit of oxygen. This is also in accordance with the microbiology of M. tuberculosis, which are well-known as bacteria actively forming colonies and biofilms. In the case of a dense placement of such colonies formed by actively breathing newborn growing (but not yet dividing) cells in sediment in the bottom part of the tube, in the vicinity of the fluorescent sensor, they formed an oxygen-impoverished surrounding medium. This part induces the inter-division fluorescence level higher than Verhulst's growth curve. At the same time, the oxygen consumption rate decays when cells are maturing and synchronizing due to cells being born at the same time; see Figure 1 (middle panel). Therefore, oxygen from the upper part of the tube can diffuse to its bottom and to damp the extra fluorescence. Note that a similar concurrence of processes is revealed recently for some other model chemical reactions when characteristic reaction and diffusion times are comparable [29].
On the contrary, the initially highly diluted culture may not form such densely packed agglomerations such that this plays the role of a primary growth limiting factor. The growth process takes sufficiently longer time (see Figure 6) i.e., the amount of the consumed oxygen is larger. Finally, there are no indications of any division synchronization processes. As a consequence, we can consider the population (and the fluorescence growth curve) as reflecting a growth process averaged over the whole sample du/dt = Ru whose growth rate is governed by the concentration s of dissolved oxygen R = R(s) ∝ s. Taking the latter as exponentially decaying, the resulting system of equations takes the form
which can be represented as a single equation:
which is the Gompertz equation.
Thus, our analysis showed that bacteria colonies may not grow smoothly but can exhibit a staged growth described by a curve with jumps in its derivative. However, this staged character disappears with dependence on the initial medium dilution. It should be noted that the time interval for cells' division can vary in the range 13–15 h. It corresponds to experimental data obtained for the strain of wild-type Mycobacterium tuberculosis H37Rv growing on Middlebrook 7H9 medium at 37 °C [30]. Although the authors used the monotonously growing fitting curve in this case, the exploration of their direct experimental data points clearly shows the existence of step-wise sequential growth patterns separated by around a 15 h interval. Note that the time resolution contains 3–4 dots per such time interval. At the same time, for the case of cold-sensitive mutants, some of which have longer duplication periods, there was revealed not only the sequence of several concentration curves concave upward with decaying DNA concentration growth rate between subsequent divisions but the synchronization of this process, too.
To make an additional test aimed at clarifying that the revealed staged growth curve is a feature of bacterial growth but not an instrumental artefact, we carried out an additional experiment using an alternative method for the growth registration: namely, the resazurin reduction assays. The reduction of blue resazurin to pink resorufin is connected with the respiratory activity of micro-organisms and belongs to the standard tests of their viability. The initial concentration of the pathogen suspension was the same as used for the experimental procedure in the BACTEC equipment (Dilution 1). The respective color change compared to pure resazurin (the control) was determined by applying the automated setup, registering the colour content by the transmitted light intensity. The details of the setup and principle of its operation can be found in the work [28]. The obtained experimental recordings are shown as markers in Figure 7; to highlight the interval of interest, the time scale origin is shifted in such a way that t = t0 corresponds to the beginning of the detectable colour change ≈ 3 days.
The time series of the data was not a smooth growth curve but a staged growth similar to Figure 1. To highlight this similarity to the data obtained by the BACTEC MGIT 960, the curves obtained by fitting the data to functions exponentially tending to saturation within subsequent 15 h subintervals are shown in Figure 7 with the dashed lines. Due to high scattering of photo-colorimetric data and limiting time resolution, it may not be logical to estimate a fitting coefficient. Figure 7 provides qualitative information and confirms that the staged growth with diminishing magnitudes of the growth rate's jumps detected with BACTEC MGIT 960 should be associated with peculiarities of the concentrated mycobacterial growing culture but not with the artefacts of the fluorescence registration, since the alternative method, not addressing fluorescence, exhibits similar behavior. There are a lot of phenomenological models of bacterial growth [31], but most used classical models, which generate sigmoidal curves resembling the great majority of data are Verhulst's and Gompertz's. In addition, they have direct interpretations from the point of view of chemical kinetics [32], mimicking a set of reactions, in which non only population density but also components of surrounding media can be included. However, it should be pointed out that more detailed study of such interactions [33] leads to the conclusion that a change of environmental conditions affects not only the growth rate but also other model parameters and even its type.
As for the possible effect of synchronization on the emergence of the staged growth, we note that it is known that one does not need to assume a perfectly synchronously dividing culture, which is definitely too strong of a condition compared to the considered experimental system. Rubinow's maturity-time/population growth time theory [34] demonstrated that such a kind of growth curve can be obtained (and detected for some micro-organisms different from M. tuberculosis) even if the growth rates and subsequent division times are probabilistically distributed. In this case, if the culture starts to grow at approximately at the same time (this condition is fulfilled by the procedure of sample preparation in our experiments), there is a small number of jumps in the growth curves, the amplitudes of which diminish due to desynchronizing. After this, the curve goes to the saturation smoothly, as is shown in the work [35], generalizing this model in the case of a saturated growth. It is practically the same behavior as revealed in our system.
It should be pointed out that some works [36]–[38], which analyzed the microscopic picture leading to the Gompertz growth law, argued that this kind of dynamics originates from stochasticity in the system of multiplying agents. This stochasticity can include a probabilistic distribution of the instant growth stages in the ensemble or arise from small fluctuations in growth rates. This property distinguishes it form the the Verhulst (logistic) growth, which can be considered as a strong averaged description of a well-mixed population growing under fixed environmental conditions.
Our results, which demonstrate the transition between these two kinds of growth curves emerging due to the system's dilution and visible extinction of features indicating a presence of the synchronization reflected in the oxygen quenching reaction in the medium, confirm these model conclusions by the direct experimental data.
Thus, we have analyzed chemical changes in medium obtained using the special system BACTEC MGIT 960. This system detects fluorescence based on the oxygen quenching sensor responding to the oxygen decrease due to active cell division. Our analysis allowed for revealing and detailed tracing biochemical processes accompanying the growth of a culture of M. tuberculosis.
We have shown that staged or continuous growth depends on the initial culture dilution which may be explained by the effect of quorum sensing. This means that there exists a certain level of a colony's density in a liquid broth, which leads to the synchronization of cellular metabolism processes responsible for inter-division mass growth and coordinated division itself. Moreover, the respective chemical response demonstrates the possibility of quantitative characterization of both processes with respect to oxygen consumption. This makes available the use of these data for the further building of similar biochemical models of bacterial metabolism with accurate timing. In particular, it is shown that the classic problem of choice between Verhulst's and Gompertz's models can be associated with the specific process, which provides a leading output during the stages of population vital activity and the synchronization rate between micro-organisms.
Finally, it should be pointed out that the metabolism of M. tuberculosis is not an exception from general biophysics and biochemistry of bacterial cultures. Therefore, the obtained results can be transferred to the studies of different microbiological cultures' growths with similar conditions.
[1] |
Keshavarz L, Ghaani MR, MacElroy JMD, et al. (2021) A comprehensive review on the application of aerogels in CO2-adsorption: Materials and characterisation. Chem Eng J 412: 128604. https://doi.org/10.1016/j.cej.2021.128604 doi: 10.1016/j.cej.2021.128604
![]() |
[2] |
Rybak A, Rybak A, Boncel S, et al. (2022) Hybrid organic-inorganic membranes based on sulfonated poly (ether ether ketone) matrix and iron-encapsulated carbon nanotubes and their application in CO2 separation. RSC Adv 12: 13367–13380. https://doi.org/10.1039/d2ra01585d doi: 10.1039/d2ra01585d
![]() |
[3] |
Sun Z, Dong J, Chen C, et al. (2021) Photocatalytic and electrocatalytic CO2 conversion: From fundamental principles to design of catalysts. J Chem Technol Biotechnol 96: 1161–1175. https://doi.org/10.1002/jctb.6653 doi: 10.1002/jctb.6653
![]() |
[4] |
Goda MN, Abdelhamid HN, Said AEAA (2020) Zirconium oxide sulfate-carbon (ZrOSO4@C) derived from carbonized UiO-66 for selective production of dimethyl ether. ACS Appl Mater Interfaces 12: 646–653. https://doi.org/10.1021/acsami.9b17520 doi: 10.1021/acsami.9b17520
![]() |
[5] |
Takht Ravanchi M, Sahebdelfar S (2021) Catalytic conversions of CO2 to help mitigate climate change: Recent process developments. Process Saf Environ Prot 145: 172–194. https://doi.org/10.1016/j.psep.2020.08.003 doi: 10.1016/j.psep.2020.08.003
![]() |
[6] |
Abdelhamid HN, Mathew AP (2022) Cellulose-based nanomaterials advance biomedicine: A review. Int J Mol Sci 23: 5405. https://doi.org/10.3390/ijms23105405 doi: 10.3390/ijms23105405
![]() |
[7] |
Younas M, Rezakazemi M, Daud M, et al. (2020) Recent progress and remaining challenges in post-combustion CO2 capture using metal-organic frameworks (MOFs). Prog Energy Combust Sci 80: 100849. https://doi.org/10.1016/j.pecs.2020.100849 doi: 10.1016/j.pecs.2020.100849
![]() |
[8] |
Kayal S, Sun B, Chakraborty A (2015) Study of metal-organic framework MIL-101(Cr) for natural gas (methane) storage and compare with other MOFs (metal-organic frameworks). Energy 91: 772–781. https://doi.org/10.1016/j.energy.2015.08.096 doi: 10.1016/j.energy.2015.08.096
![]() |
[9] |
Qin JS, Yuan S, Alsalme A, et al. (2017) Flexible zirconium MOF as the crystalline sponge for coordinative alignment of dicarboxylates. ACS Appl Mater Interfaces 9: 33408–33412. https://doi.org/10.1021/acsami.6b16264 doi: 10.1021/acsami.6b16264
![]() |
[10] |
Joharian M, Morsali A (2019) Ultrasound-assisted synthesis of two new fluorinated metal-organic frameworks (F-MOFs) with the high surface area to improve the catalytic activity. J Solid State Chem 270: 135–146. https://doi.org/10.1016/j.jssc.2018.10.046 doi: 10.1016/j.jssc.2018.10.046
![]() |
[11] |
Bux H, Liang F, Li Y, et al. (2009) Zeolitic imidazolate framework membrane with molecular sieving properties by microwave-assisted solvothermal synthesis. J Am Chem Soc 131: 16000–16001. https://doi.org/10.1021/ja907359t doi: 10.1021/ja907359t
![]() |
[12] |
Park KS, Ni Z, Côté AP, et al. (2006) Exceptional chemical and thermal stability of zeolitic imidazolate frameworks. Proc Natl Acad Sci USA 103: 10186–10191. https://doi.org/10.1073/pnas.0602439103 doi: 10.1073/pnas.0602439103
![]() |
[13] |
Sorribas S, Zornoza B, Teílez C, et al. (2012) Ordered mesoporous silica-(ZIF-8) core-shell spheres. Chem Commun 48: 9388–9390. https://doi.org/10.1039/C2CC34893D doi: 10.1039/C2CC34893D
![]() |
[14] |
Sánchez-Laínez J, Zornoza B, Friebe S, et al. (2016) Influence of ZIF-8 particle size in the performance of polybenzimidazole mixed matrix membranes for pre-combustion CO2 capture and its validation through interlaboratory test. J Membr Sci 515: 45–53. https://doi.org/10.1016/j.memsci.2016.05.039 doi: 10.1016/j.memsci.2016.05.039
![]() |
[15] |
Kim D, Kim W, Buyukcakir O, et al. (2017) Highly hydrophobic ZIF-8/carbon nitride foam with hierarchical porosity for oil capture and chemical fixation of CO2. Adv Funct Mater 27: 1700706. https://doi.org/10.1002/adfm.201700706 doi: 10.1002/adfm.201700706
![]() |
[16] |
Gong X, Wang Y, Kuang T (2017) ZIF-8-based membranes for carbon dioxide capture and separation. ACS Sustain Chem Eng 5: 11204–11214. https://doi.org/10.1021/acssuschemeng.7b03613 doi: 10.1021/acssuschemeng.7b03613
![]() |
[17] |
Zhang Z, Xian S, Xi H, et al. (2011) Improvement of CO2 adsorption on ZIF-8 crystals modified by enhancing basicity of surface. Chem Eng Sci 66: 4878–4888. https://doi.org/10.1016/j.ces.2011.06.051 doi: 10.1016/j.ces.2011.06.051
![]() |
[18] |
Yahia M, Phan Le QN, Ismail N, et al. (2021) Effect of incorporating different ZIF-8 crystal sizes in the polymer of intrinsic microporosity, PIM-1, for CO2/CH4 separation. Microporous Mesoporous Mater 312: 110761. https://doi.org/10.1016/j.micromeso.2020.110761 doi: 10.1016/j.micromeso.2020.110761
![]() |
[19] |
Bux H, Chmelik C, Krishna R, et al. (2011) Ethene/ethane separation by the MOF membrane ZIF-8: Molecular correlation of permeation, adsorption, diffusion. J Membr Sci 369: 284–289. https://doi.org/10.1016/j.memsci.2010.12.001 doi: 10.1016/j.memsci.2010.12.001
![]() |
[20] |
Shi Q, Song Z, Kang X, et al. (2012) Controlled synthesis of hierarchical zeolitic imidazolate framework-GIS (ZIF-GIS) architectures. Cryst Eng Comm 14: 8280–8285. https://doi.org/10.1039/C2CE26170G doi: 10.1039/C2CE26170G
![]() |
[21] |
Lai LS, Yeong YF, Ani NC, et al. (2014) Effect of synthesis parameters on the formation of Zeolitic Imidazolate Framework 8 (ZIF-8) nanoparticles for CO2 adsorption. Sep Sci Technol 49: 520–528. https://doi.org/10.1080/02726351.2014.920445 doi: 10.1080/02726351.2014.920445
![]() |
[22] |
Zeng X, Huang L, Wang C, et al. (2016) Sonocrystallization of ZIF-8 on electrostatic spinning TiO2 nanofibers surface with enhanced photocatalysis property through synergistic effect. ACS Appl Mater Interfaces 8: 20274–20282. https://doi.org/10.1021/acsami.6b05746 doi: 10.1021/acsami.6b05746
![]() |
[23] |
Venna SR, Jasinski JB, Carreon MA (2010) Structural evolution of Zeolitic Imidazolate Framework-8. J Am Chem Soc 132: 7. https://doi.org/10.1021/ja109268m doi: 10.1021/ja109268m
![]() |
[24] |
Xiang L, Sheng L, Wang C, et al. (2017) Amino-functionalized ZIF-7 nanocrystals: Improved intrinsic separation ability and interfacial compatibility in mixed-matrix membranes for CO2/CH4 separation. Adv Mater 29: 1–8. https://doi.org/10.1002/adma.201606999 doi: 10.1002/adma.201606999
![]() |
[25] |
Abdelhamid HN (2020) UiO-66 as a catalyst for hydrogen production via the hydrolysis of sodium borohydride. Dalton Trans 49: 10851. https://doi.org/10.1039/D0DT01688H doi: 10.1039/D0DT01688H
![]() |
[26] |
Abdelhaleem A, Abdelhamid HN, Ibrahim MG, et al. (2022) Photocatalytic degradation of paracetamol using photo-Fenton-like metal-organic framework-derived CuO@C under visible LED. J Clean Prod 379: 134571. https://doi.org/10.1016/j.jclepro.2022.134571 doi: 10.1016/j.jclepro.2022.134571
![]() |
[27] |
Madejski P, Chmiel K, Subramanian N, et al. (2022) Methods and techniques for CO2 capture: Review of potential solutions and applications in modern energy technologies. Energies 15: 887. https://doi.org/10.3390/en15030887 doi: 10.3390/en15030887
![]() |
[28] |
Gładysz P, Stanek W, Czarnowska L, et al. (2018) Thermo-ecological evaluation of an integrated MILD oxy-fuel combustion power plant with CO2 capture, utilization, and storage—A case study in Poland. Energy 144: 379–392. https://doi.org/10.1016/j.energy.2017.11.133 doi: 10.1016/j.energy.2017.11.133
![]() |
[29] |
Tramošljika B, Blecich P, Bonefačić I, et al. (2021) Advanced ultra-supercritical coal-fired power plant with post-combustion carbon capture: Analysis of electricity penalty and CO2 emission reduction. Sustainability 13: 1–20. https://doi.org/10.3390/su13020801 doi: 10.3390/su13020801
![]() |
[30] |
Holz F, Scherwath T, Crespo del Granado P, et al. (2021) A 2050 perspective on the role for carbon capture and storage in the European power system and industry sector. Energy Econ 104: 105631. https://doi.org/10.1016/j.eneco.2021.105631 doi: 10.1016/j.eneco.2021.105631
![]() |
[31] |
Prat D, Wells A, Hayler J, et al. (2015) CHEM21 selection guide of classical-and less classical-solvents. Green Chem 18: 288. https://doi.org/10.1039/C5GC01008J doi: 10.1039/C5GC01008J
![]() |
[32] |
Kato M, Essaki K, Nakagawa K, et al. (2005) CO2 absorption properties of lithium ferrite for application as a high-temperature CO2 absorbent. J Ceram Soc Jpn 113: 684–686. https://doi.org/10.2109/jcersj.113.684 doi: 10.2109/jcersj.113.684
![]() |
[33] |
Samanta A, Zhao A, Shimizu GKH, et al. (2012) Post-combustion CO2 capture using solid sorbents: A review. Ind Eng Chem Res 51: 1438–1463. https://doi.org/10.1021/ie200686q doi: 10.1021/ie200686q
![]() |
[34] |
Omoregbe O, Mustapha AN, Steinberger-Wilckens R, et al. (2020) Carbon capture technologies for climate change mitigation: A bibliometric analysis of the scientific discourse during 1998–2018. Energy Rep 6: 1200–1212. https://doi.org/10.1016/j.egyr.2020.05.003 doi: 10.1016/j.egyr.2020.05.003
![]() |
[35] |
Yamauchi K, Murayama N, Shibata J (2007) Absorption and release of carbon dioxide with various metal oxides and hydroxides. Mater Trans 48: 2739–2742. https://doi.org/10.2320/matertrans.M-MRA2007877 doi: 10.2320/matertrans.M-MRA2007877
![]() |
[36] |
Spataru CI, Ianchis R, Petcu C, et al. (2016) Synthesis of non-toxic silica particles stabilized by molecular complex oleic-acid/sodium oleate. Int J Mol Sci 17: 4–8. https://doi.org/10.3390/ijms17111936 doi: 10.3390/ijms17111936
![]() |
[37] |
Pimenidou P, Dupont V (2015) Dolomite study for in situ CO2 capture for chemical looping reforming. Int J Ambient Energy 36: 170–182. https://doi.org/10.1080/01430750.2013.841590 doi: 10.1080/01430750.2013.841590
![]() |
[38] |
Bhown AS, Freeman BC (2011) Analysis and status of post-combustion carbon dioxide capture technologies. Environ Sci Technol 45: 23. https://doi.org/10.1021/es104291d doi: 10.1021/es104291d
![]() |
[39] |
Feron PHM, Hendriks CA (2005) CO2 capture process principles and costs. Oil Gas Sci Technol 60: 451–459. https://doi.org/10.2516/ogst:2005027 doi: 10.2516/ogst:2005027
![]() |
[40] |
Yampolskii Y, Topchiev AV (2012) Polymeric gas separation membranes. Russ Chem Rev 81: 483–500. https://doi.org/10.1021/ma300213b doi: 10.1021/ma300213b
![]() |
[41] |
Siqueira RM, Freitas GR, Peixoto HR, et al. (2017) Carbon dioxide capture by pressure swing adsorption. Energy Procedia 114: 2182–2192. https://doi.org/10.1016/j.egypro.2017.03.1355 doi: 10.1016/j.egypro.2017.03.1355
![]() |
[42] |
Yang MW, Chen NC, Huang CH, et al. (2014) Temperature swing adsorption process for CO2 capture using polyaniline solid sorbent. Energy Procedia 63: 2351–2358. https://doi.org/10.1016/j.egypro.2014.11.256 doi: 10.1016/j.egypro.2014.11.256
![]() |
[43] |
Saenz Cavazos PA, Hunter-Sellars E, Iacomi P, et al. (2023) Evaluating solid sorbents for CO2 capture: Linking material properties and process efficiency via adsorption performance. Front Energy Res 11: 1–22. https://doi.org/10.3389/fenrg.2023.1167043 doi: 10.3389/fenrg.2023.1167043
![]() |
[44] |
Tsai CW, Langner EHG, Harris RA (2019) Computational study of ZIF-8 analogues with electron donating and withdrawing groups for CO2 adsorption. Microporous Mesoporous Mater 288: 109613. https://doi.org/10.1016/j.micromeso.2019.109613 doi: 10.1016/j.micromeso.2019.109613
![]() |
[45] |
Junaidi MUM, Khoo CP, Leo CP, et al. (2014) The effects of solvents on the modification of SAPO-34 zeolite using 3-aminopropyl trimethoxy silane for the preparation of asymmetric polysulfone mixed matrix membrane in the application of CO2 separation. Microporous Mesoporous Mater 192: 52–59. https://doi.org/10.1016/j.micromeso.2013.10.006 doi: 10.1016/j.micromeso.2013.10.006
![]() |
[46] |
Song C, Liu Q, Deng S, et al. (2019) Cryogenic-based CO2 capture technologies: State-of-the-art developments and current challenges. Renew Sustain Energy Rev 101: 265–278. https://doi.org/10.1016/j.rser.2018.11.018 doi: 10.1016/j.rser.2018.11.018
![]() |
[47] |
Ahmed R, Liu G, Yousaf B, et al. (2020) Recent advances in carbon-based renewable adsorbent for selective carbon dioxide capture and separation—A review. J Clean Prod 242: 118409. https://doi.org/10.1016/j.jclepro.2019.118409 doi: 10.1016/j.jclepro.2019.118409
![]() |
[48] |
Zulkurnai NZ, Mohammad Ali UF, Ibrahim N, et al. (2017) Carbon dioxide (CO2) adsorption by activated carbon functionalized with deep eutectic solvent (DES). IOP Conf Ser Mater Sci Eng 206: 012030. https://dx.doi.org/10.1088/1757-899X/206/1/012001 doi: 10.1088/1757-899X/206/1/012001
![]() |
[49] |
Ngoy JM, Wagner N, Riboldi L, et al. (2014) A CO2 capture technology using multi-walled carbon nanotubes with polyaspartamide surfactant. Energy Procedia 63: 2230–2248. https://doi.org/10.1016/j.egypro.2014.11.242 doi: 10.1016/j.egypro.2014.11.242
![]() |
[50] |
Singh S, Varghese AM, Reinalda D, et al. (2021) Graphene-based membranes for carbon dioxide separation. J CO2 Util 49: 101544. https://doi.org/10.1016/j.jcou.2021.101544 doi: 10.1016/j.jcou.2021.101544
![]() |
[51] |
Agrafioti E, Bouras G, Kalderis D, et al. (2013) Biochar production by sewage sludge pyrolysis. J Anal Appl Pyrolysis 101: 72–78. https://doi.org/10.1016/j.jaap.2013.02.010 doi: 10.1016/j.jaap.2013.02.010
![]() |
[52] |
Liu Y, Ren Y, Ma H, et al. (2022) Advanced organic molecular sieve membranes for carbon capture: Current status, challenges and prospects. Adv Membr 2: 100028. https://doi.org/10.1016/j.advmem.2022.100028 doi: 10.1016/j.advmem.2022.100028
![]() |
[53] |
Yin M, Zhang L, Wei X, et al. (2022) Detection of antibiotics by electrochemical sensors based on metal-organic frameworks and their derived materials. Microchem J 183: 107946. https://doi.org/10.1016/j.microc.2022.107946 doi: 10.1016/j.microc.2022.107946
![]() |
[54] |
Rui Z, James JB, Lin YS (2018) Highly CO2 perm-selective metal-organic framework membranes through CO2 annealing post-treatment. J Membr Sci 555: 97–104. https://doi.org/10.1016/j.memsci.2018.03.036 doi: 10.1016/j.memsci.2018.03.036
![]() |
[55] |
Lee YR, Kim J, Ahn WS (2013) Synthesis of metal-organic frameworks: A mini review. Korean J Chem Eng 30: 1667–1680. https://doi.org/10.1007/s11814-013-0140-6 doi: 10.1007/s11814-013-0140-6
![]() |
[56] |
Demir H, Aksu GO, Gulbalkan HC, et al. (2022) MOF membranes for CO2 capture: Past, present and future. Carbon Capture Sci Technol 2: 100026. https://doi.org/10.1016/j.ccst.2021.100026 doi: 10.1016/j.ccst.2021.100026
![]() |
[57] |
Schaate A, Roy P, Godt A, et al. (2011) Modulated synthesis of Zr-based metal-organic frameworks: From nano to single crystals. Chem Eur J 17: 6643–6651. https://doi.org/10.1002/chem.201003211 doi: 10.1002/chem.201003211
![]() |
[58] |
Bux H, Feldhoff A, Cravillon J, et al. (2011) Oriented zeolitic imidazolate framework-8 membrane with sharp H2/C3H8 molecular sieve separation. Chem Mater 23: 2262–2269. https://doi.org/10.1021/cm200555s doi: 10.1021/cm200555s
![]() |
[59] |
Chen Y, Tang S (2019) Solvothermal synthesis of porous hydrangea-like zeolitic imidazolate framework-8 (ZIF-8) crystals. J Solid State Chem 276: 68–74. https://doi.org/10.1016/j.jssc.2019.04.034 doi: 10.1016/j.jssc.2019.04.034
![]() |
[60] |
Ejeromedoghene O, Oderinde O, Okoye CO, et al. (2022) Microporous metal-organic frameworks based on deep eutectic solvents for adsorption of toxic gases and volatile organic compounds: A review. Chem Eng J Adv 12: 100361. https://doi.org/10.1016/j.ceja.2022.100361 doi: 10.1016/j.ceja.2022.100361
![]() |
[61] |
Park JH, Park SH, Jhung SH (2009) Microwave-syntheses of zeolitic imidazolate framework material, ZIF-8. J Korean Chem Soc 53: 553–559. https://doi.org/10.5012/jkcs.2009.53.5.553 doi: 10.5012/jkcs.2009.53.5.553
![]() |
[62] |
Cravillon J, Münzer S, Lohmeier SJ, et al. (2009) Rapid room-temperature synthesis and characterization of nanocrystals of a prototypical zeolitic imidazolate framework. Chem Mater 21: 1410–1412. https://doi.org/10.1021/cm900166h doi: 10.1021/cm900166h
![]() |
[63] | Mullin JW (2001) Crystallization: Chapter 5—Nucleation, In: Mullin JW, Crystallization, Oxford: Butterworth-Heinemann, 181–215. https://doi.org/10.1016/B978-075064833-2/50007-3 |
[64] |
Bazzi L, Ayouch I, Tachallait H, et al. (2022) Ultrasound and microwave assisted-synthesis of ZIF-8 from zinc oxide for the adsorption of phosphate. Results Eng 13: 100378. https://doi.org/10.1016/j.rineng.2022.100378 doi: 10.1016/j.rineng.2022.100378
![]() |
[65] |
Beldon PJ, Fábián L, Stein RS, et al. (2010) Rapid room-temperature synthesis of zeolitic imidazolate frameworks by using mechanochemistry. Angew Chem Int Ed 49: 9640–9643. https://doi.org/10.1002/anie.201005547 doi: 10.1002/anie.201005547
![]() |
[66] |
Bang JH, Suslick KS (2010) Applications of ultrasound to the synthesis of nanostructured materials. Adv Mater 22: 1039–1059. https://doi.org/10.1002/adma.200904093 doi: 10.1002/adma.200904093
![]() |
[67] |
Cho HY, Kim J, Kim SN, et al. (2012) High yield 1-L scale synthesis of ZIF-8 via a sonochemical route. Microporous Mesoporous Mater 156: 171–177. https://doi.org/10.1016/j.micromeso.2012.11.012 doi: 10.1016/j.micromeso.2012.11.012
![]() |
[68] |
Chalati T, Horcajada P, Gref R, et al. (2010) Optimisation of the synthesis of MOF nanoparticles made of flexible porous iron fumarate MIL-88A. J Mater Chem 20: 7676–7681. https://doi.org/10.1039/C0JM03563G doi: 10.1039/C0JM03563G
![]() |
[69] |
Pichon A, Lazuen-Garay A, James SL (2006) Solvent-free synthesis of a microporous metal-organic framework. CrystEngComm 8: 211–214. https://doi.org/10.1039/B513750K doi: 10.1039/B513750K
![]() |
[70] |
Carson CG, Brown AJ, Sholl DS, et al. (2022) Sonochemical synthesis and characterization of submicrometer crystals of the metal-organic framework Cu[(hfipbb)(H2hfipbb)0.5]. J Mater Chem 19: 18. https://doi.org/10.1021/cg200728b doi: 10.1021/cg200728b
![]() |
[71] |
Seyedin S, Zhang J, Usman KAS, et al. (2019) Facile solution processing of stable MXene dispersions towards conductive composite fibers. Glob Chall 3: 1900037. https://doi.org/10.1002/gch2.201900037 doi: 10.1002/gch2.201900037
![]() |
[72] |
Venna SR, Carreon MA (2010) Highly permeable zeolite imidazolate framework-8 membranes for CO2/CH4 separation. J Am Chem Soc 132: 76–78. https://doi.org/10.1021/ja909263x doi: 10.1021/ja909263x
![]() |
[73] |
Son WJ, Choi JS, Ahn WS (2008) Adsorptive removal of carbon dioxide using polyethyleneimine-loaded mesoporous silica materials. Microporous Mesoporous Mater 113: 31–40. https://doi.org/10.1016/j.micromeso.2007.10.049 doi: 10.1016/j.micromeso.2007.10.049
![]() |
[74] |
Klimakow M, Klobes P, Thünemann AF, et al. (2010) Mechanochemical synthesis of metal-organic frameworks: a fast and facile approach toward quantitative yields and high specific surface areas. Chem Mater 22: 5216–5221. https://doi.org/10.1021/cm1012119 doi: 10.1021/cm1012119
![]() |
[75] |
Yue Y, Qiao ZA, Li X, et al. (2013) Nanostructured zeolitic imidazolate frameworks derived from nanosized zinc oxide precursors. Cryst Growth Des 13: 1002–1005. https://doi.org/10.1021/cg4002362 doi: 10.1021/cg4002362
![]() |
[76] |
Usman KAS, Maina JW, Seyedin S, et al. (2020) Downsizing metal–organic frameworks by bottom-up and top-down methods. NPG Asia Mater 12: 58. https://doi.org/10.1038/s41427-020-00240-5 doi: 10.1038/s41427-020-00240-5
![]() |
[77] |
Ogura M, Nakata SI, Kikuchi E, et al. (2001) Effect of NH4+ exchange on hydrophobicity and catalytic properties of Al-free Ti–Si–beta zeolite. J Catal 199: 41–47. https://doi.org/10.1006/jcat.2000.3156 doi: 10.1006/jcat.2000.3156
![]() |
[78] |
Gökpinar S, Diment T, Janiak C (2017) Environmentally benign dry-gel conversions of Zr-based UiO metal–organic frameworks with high yield and the possibility of solvent re-use. Dalton Trans 46: 9895. https://doi.org/10.1039/C7DT01717K doi: 10.1039/C7DT01717K
![]() |
[79] |
Awadallah-F A, Hillman F, Al-Muhtaseb SA, et al. (2019) Nano-gate opening pressures for the adsorption of isobutane, n-butane, propane, and propylene gases on bimetallic Co–Zn based zeolitic imidazolate frameworks. Microporous Mesoporous Mater 48: 4685. https://doi.org/10.1039/C9DT00222G doi: 10.1039/C9DT00222G
![]() |
[80] |
Tannert N, Gökpinar S, Hastürk E, et al. (2018) Microwave-assisted dry-gel conversion—A new sustainable route for the rapid synthesis of metal-organic frameworks with solvent re-use. Dalton Trans 47: 9850–9860. https://doi.org/10.1039/C8DT02029A doi: 10.1039/C8DT02029A
![]() |
[81] |
Ahmed I, Jeon J, Khan NA, et al. (2012) Synthesis of a metal−organic framework, iron-benezenetricarboxylate, from dry gels in the absence of acid and salt. Cryst Growth Des. 12: 5878–5881. https://doi.org/10.1021/cg3014317 doi: 10.1021/cg3014317
![]() |
[82] |
Jahn A, Reiner JE, Vreeland WN, et al. (2008) Preparation of nanoparticles by continuous-flow microfluidics. J Nanopart Res 10: 925–934. https://doi.org/10.1007/s11051-007-9340-5 doi: 10.1007/s11051-007-9340-5
![]() |
[83] |
Lee YR, Jang MS, Cho HY, et al. (2015) ZIF-8: A comparison of synthesis methods. Chem Eng J 271: 276–280. https://doi.org/10.1016/j.cej.2015.02.094 doi: 10.1016/j.cej.2015.02.094
![]() |
[84] |
Kolmykov O, Commenge JM, Alem H, et al. (2017) Microfluidic reactors for the size-controlled synthesis of ZIF-8 crystals in aqueous phase. Mater Des 122: 31–41. https://doi.org/10.1016/j.matdes.2017.03.002 doi: 10.1016/j.matdes.2017.03.002
![]() |
[85] | Schejn A, Frégnaux M, Commenge JM, et al. (2014) Size-controlled synthesis of ZnO quantum dots in microreactors. Nanotechnology 25. https://iopscience.iop.org/article/10.1088/0957-4484/25/14/145606/data |
[86] |
Gross AF, Sherman E, Vajo JJ (2012) Aqueous room temperature synthesis of cobalt and zinc sodalite zeolitic imidizolate frameworks. Dalton Trans 41: 5458–5460. https://doi.org/10.1039/C2DT30174A doi: 10.1039/C2DT30174A
![]() |
[87] |
Faustini M, Kim J, Jeong GY, et al. (2013) Microfluidic approach toward continuous and ultrafast synthesis of metal−organic framework crystals and hetero structures in confined microdroplets. J Am Chem Soc 135: 14619–14626. https://doi.org/10.1021/ja4039642 doi: 10.1021/ja4039642
![]() |
[88] |
Yamamoto D, Maki T, Watanabe S, et al. (2013) Synthesis and adsorption properties of ZIF-8 nanoparticles using a micromixer. Chem Eng J 227: 145–150. https://doi.org/10.1016/j.cej.2012.08.065 doi: 10.1016/j.cej.2012.08.065
![]() |
[89] | Butova VV, Soldatov MA, Guda AA, et al. (2016) Metal-organic frameworks: structure, properties, methods of synthesis and characterization. Russ Chem Rev 85: 280–307. https://iopscience.iop.org/article/10.1070/RCR4554 |
[90] |
Butova VV, Budnyk AP, Bulanova EA, et al. (2017) Hydrothermal synthesis of high surface area ZIF-8 with minimal use of TEA. Solid State Sci 69: 13–21. https://doi.org/10.1016/j.solidstatesciences.2017.05.002 doi: 10.1016/j.solidstatesciences.2017.05.002
![]() |
[91] |
Riley BJ, Vienna JD, Strachan DM, et al. (2016) Materials and processes for the effective capture and immobilization of radioiodine: A review. J Nucl Mater 470: 307–326. https://doi.org/10.1016/j.jnucmat.2015.11.038 doi: 10.1016/j.jnucmat.2015.11.038
![]() |
[92] |
Saini K, Yadav S, Jain M, et al. (2021) Recent advances and challenges in selective environmental applications of metal-organic frameworks. ACS Symp Ser 1394: 223–245. http://dx.doi.org/10.1021/bk-2021-1394.ch009 doi: 10.1021/bk-2021-1394.ch009
![]() |
[93] | Lestari G (2012) Hydrothermal synthesis of zeolitic imidazolate frameworks-8 (ZIF-8) crystals with controllable size and morphology. MS Thesis, King Abdullah University of Science and Technology. https://doi.org/10.25781/KAUST-461G0 |
[94] |
Pan Y, Liu Y, Zeng G, et al. (2011) Rapid synthesis of zeolitic imidazolate framework-8 (ZIF-8) nanocrystals in an aqueous system. Chem Commun 47: 2071–2073. https://doi.org/10.1039/C0CC05002D doi: 10.1039/C0CC05002D
![]() |
[95] |
Huang XC, Lin YY, Zhang JP, et al. (2006) Ligand-directed strategy for zeolite-type metal-organic frameworks: Zinc(ii) imidazolates with unusual zeolitic topologies. Angew Chem Int Ed 45: 1557–1559. https://doi.org/10.1002/anie.200503778 doi: 10.1002/anie.200503778
![]() |
[96] |
Chiang YC, Chin WT, Huang CC (2021) The application of hollow carbon nanofibers prepared by electrospinning to carbon dioxide capture. J Mater Sci 56: 2490–2502. https://doi.org/10.3390/polym13193275 doi: 10.3390/polym13193275
![]() |
[97] |
Malekmohammadi M, Fatemi S, Razavian M, et al. (2019) A comparative study on ZIF-8 synthesis in aqueous and methanolic solutions: Effect of temperature and ligand content. Solid State Sci 91: 108–112. https://doi.org/10.1016/j.solidstatesciences.2019.03.022 doi: 10.1016/j.solidstatesciences.2019.03.022
![]() |
[98] |
Xian S, Xu F, Ma C, et al. (2015) Vapor-enhanced CO2 adsorption mechanism of composite PEI@ZIF-8 modified by polyethyleneimine for CO2/N2 separation. Chem Eng J 280: 363–369. https://doi.org/10.1016/j.cej.2015.06.042 doi: 10.1016/j.cej.2015.06.042
![]() |
[99] |
Hao F, Yan XP (2022) Nano-sized zeolite-like metal-organic frameworks induced hematological effects on red blood cell. J Hazard Mater 424: 127353. https://doi.org/10.1016/j.jhazmat.2021.127353 doi: 10.1016/j.jhazmat.2021.127353
![]() |
[100] |
Zheng W, Ding R, Yang K, et al. (2019) ZIF-8 nanoparticles with tunable size for enhanced CO2 capture of Pebax based MMMs. Sep Purif Technol 214: 111–119. https://doi.org/10.1016/j.seppur.2018.04.010 doi: 10.1016/j.seppur.2018.04.010
![]() |
[101] |
Jiang HL, Liu B, Akita T, et al. (2009) Au@ZIF-8: CO oxidation over gold nanoparticles deposited to metal-organic framework. J Am Chem Soc 131: 11302–11303. https://doi.org/10.1021/ja9047653 doi: 10.1021/ja9047653
![]() |
[102] |
Kong X, Yu Y, Ma S, et al. (2018) Adsorption mechanism of H2O molecule on the Li4SiO4 (010) surface from first principles. Chem Phys Lett 691: 1–7. https://doi.org/10.1016/j.cplett.2017.10.054 doi: 10.1016/j.cplett.2017.10.054
![]() |
[103] |
Chen B, Yang Z, Zhu Y, et al. (2014) Zeolitic imidazolate framework materials: recent progress in synthesis and applications J Mater Chem A 2: 16811–16831. https://doi.org/10.1039/C4TA02984D doi: 10.1039/C4TA02984D
![]() |
[104] |
Chiang YC, Chin WT (2022) Preparation of zeolitic imidazolate framework-8-based nanofiber composites for carbon dioxide adsorption. Nanomaterials 12: 1492. https://doi.org/10.3390/nano12091492 doi: 10.3390/nano12091492
![]() |
[105] |
Aulia W, Ahnaf A, Irianto MY, et al. (2020) Synthesis and characterization of zeolitic imidazolate framework-8 (ZIF-8)/Al2O3 composite. IPTEK 31: 18–24. https://doi.org/10.12962/j20882033.v31i1.5511 doi: 10.12962/j20882033.v31i1.5511
![]() |
[106] |
Su Z, Zhang M, Lu Z, et al. (2018) Functionalization of cellulose fiber by in situ growth of zeolitic imidazolate framework-8 (ZIF-8) nanocrystals for preparing a cellulose-based air filter with gas adsorption ability. Cellulose 25: 1997–2008. https://doi.org/10.1007/s10570-018-1696-4 doi: 10.1007/s10570-018-1696-4
![]() |
[107] |
Payra S, Challagulla S, Indukuru RR, et al (2018) The structural and surface modification of zeolitic imidazolate frameworks towards reduction of encapsulated CO2. New J Chem 42: 19205–19213. https://doi.org/10.1039/C8NJ04247K doi: 10.1039/C8NJ04247K
![]() |
[108] |
Chen R, Yao J, Gu Q, et al. (2013) A two-dimensional zeolitic imidazolate framework with a cushion-shaped cavity for CO2 adsorption. Chem Commun 49: 9500. https://doi.org/10.1039/C3CC44342F doi: 10.1039/C3CC44342F
![]() |
[109] |
Awadallah-F A, Hillman F, Al-Muhtaseb SA, et al. (2019) On the nanogate-opening pressures of copper-doped zeolitic imidazolate framework ZIF-8 for the adsorption of propane, propylene, isobutane, and n-butane. J Mater Sci 54: 5513–5527. https://doi.org/10.1007/s10853-018-03249-y doi: 10.1007/s10853-018-03249-y
![]() |
[110] |
Cho HY, Kim J, Kim SN, et al. (2013) High yield 1-L scale synthesis of ZIF-8 via a sonochemical route. Micropor Mesopor Mat 169: 180–184. https://doi.org/10.1016/j.micromeso.2012.11.012 doi: 10.1016/j.micromeso.2012.11.012
![]() |
[111] |
Fu F, Zheng B, Xie LH, et al. (2018) Size-controllable synthesis of zeolitic imidazolate framework/carbon nanotube composites. Crystals 8: 367. https://doi.org/10.3390/cryst8100367 doi: 10.3390/cryst8100367
![]() |
[112] |
Du PD, Hieu NT, Thien TV (2021) Ultrasound-assisted rapid ZIF-8 synthesis, porous ZnO preparation by heating ZIF-8, and their photocatalytic activity. J Nanomater 2021: 9988998. https://doi.org/10.1155/2021/9988998 doi: 10.1155/2021/9988998
![]() |
[113] |
Modi A, Jiang Z, Kasher R (2022) Hydrostable ZIF-8 layer on polyacrylonitrile membrane for efficient treatment of oilfield produced water. Chem Eng J 434: 133513. https://doi.org/10.1016/j.cej.2021.133513 doi: 10.1016/j.cej.2021.133513
![]() |
[114] |
Mphuthi LE, Erasmus E, Langner EHG (2021) Metal exchange of ZIF-8 and ZIF-67 nanoparticles with Fe(Ⅱ) for enhanced photocatalytic performance. ACS Omega 6: 31632–31645. https://doi.org/10.1021/acsomega.1c04142 doi: 10.1021/acsomega.1c04142
![]() |
[115] |
Wu T, Dong J, De France K, et al. (2020) Porous carbon frameworks with high CO2 capture capacity derived from hierarchical polyimide/zeolitic imidazolate frameworks composite aerogels. Chem Eng J 395: 124927. https://doi.org/10.1016/j.cej.2020.124927 doi: 10.1016/j.cej.2020.124927
![]() |
[116] |
Aceituno Melgar VM, Ahn H, Kim J, et al. (2015) Zeolitic imidazolate framework membranes for gas separation: A review of synthesis methods and gas separation performance. J Ind Eng Chem 28: 1–15. https://doi.org/10.1016/j.jiec.2015.03.006 doi: 10.1016/j.jiec.2015.03.006
![]() |
[117] |
Drobek M, Bechelany M, Vallicari C, et al. (2015) An innovative approach for the preparation of confined ZIF-8 membranes by conversion of ZnO ALD layers. J Membr Sci 475: 39–46. https://doi.org/10.1016/j.memsci.2014.10.011 doi: 10.1016/j.memsci.2014.10.011
![]() |
[118] |
Kenyotha K, Chanapattharapol KC, McCloskey S (2020) Water based synthesis of ZIF-8 assisted by hydrogen bond acceptors and enhancement of CO2 uptake by solvent assisted ligand exchange. Crystals 10: 599. https://doi.org/10.3390/cryst10070599 doi: 10.3390/cryst10070599
![]() |
[119] |
Ding B, Wang X, Xu Y, et al. (2018) Hydrothermal preparation of hierarchical ZIF-L nanostructures for enhanced CO2 capture. J Colloid Interface Sci 519: 38–43. https://doi.org/10.1016/j.jcis.2018.02.047 doi: 10.1016/j.jcis.2018.02.047
![]() |
[120] |
Chen Y, Wang B, Zhao L, et al. (2015) New Pebax®/zeolite Y composite membranes for CO2 capture from flue gas. J Membr Sci 495: 415–423. https://doi.org/10.1016/j.memsci.2015.08.045 doi: 10.1016/j.memsci.2015.08.045
![]() |
[121] |
McEwen J, Hayman JD, Ozgur Yazaydin A, et al. (2013) A comparative study of CO2, CH4 and N2 adsorption in ZIF-8, Zeolite-13X and BPL activated carbon. Chem Phys 412: 72–76. https://doi.org/10.1016/j.chemphys.2012.12.012 doi: 10.1016/j.chemphys.2012.12.012
![]() |
[122] |
Papchenko K, Risaliti G, Ferroni M, et al. (2021) An analysis of the effect of ZIF-8 addition on the separation properties of polysulfone at various temperatures. Membranes 11: 427. https://doi.org/10.3390/membranes11060427 doi: 10.3390/membranes11060427
![]() |
[123] |
Ma H, Wang Z, Zhang XF, et al. (2021) In situ growth of amino-functionalized ZIF-8 on bacterial cellulose foams for enhanced CO2 adsorption. Carbohydr Polym 270: 118376. https://doi.org/10.1016/j.carbpol.2021.118376 doi: 10.1016/j.carbpol.2021.118376
![]() |
[124] |
Korelskiy D, Ye P, Fouladvand S, et al. (2015) Efficient ceramic zeolite membranes for CO2/H2 separation. J Mater Chem A 3: 12500–12506. https://doi.org/10.1039/C5TA02152A doi: 10.1039/C5TA02152A
![]() |
[125] |
Ban Y, Li Y, Peng Y, et al. (2014) Metal-substituted zeolitic imidazolate framework ZIF-108: Gas-sorption and membrane-separation properties. Chem Eur J 20: 11402–11409. https://doi.org/10.1002/chem.201402287 doi: 10.1002/chem.201402287
![]() |
[126] |
Al Abdulla S, Sabouni R, Ghommem M, et al. (2023) Synthesis and performance analysis of zeolitic imidazolate frameworks for CO2 sensing applications. Heliyon 9: e21349. https://doi.org/10.1016/j.heliyon.2023.e21349 doi: 10.1016/j.heliyon.2023.e21349
![]() |
[127] |
Wang B, Côté AP, Furukawa H, et al. (2008) Colossal cages in zeolitic imidazolate frameworks as selective carbon dioxide reservoirs. Nature 453: 207–211. https://doi.org/10.1038/nature06900 doi: 10.1038/nature06900
![]() |
[128] |
Banerjee R, Phan A, Wang B, et al. (2008) High-throughput synthesis of zeolitic imidazolate frameworks and application to CO2 capture. Science 319: 939–943. https://doi.org/10.1126/science.1152516 doi: 10.1126/science.1152516
![]() |
[129] |
Chen C, Kim J, Yang DA, et al. (2011) Carbon dioxide adsorption over zeolite-like metal-organic frameworks (ZMOFs) having a sod topology: Structure and ion-exchange effect. Chem Eng J 168: 1134–1139. https://doi.org/10.1016/j.cej.2011.01.096 doi: 10.1016/j.cej.2011.01.096
![]() |
[130] |
Shieh FK, Wang SC, Leo SY, et al. (2013) Water-based synthesis of zeolitic imidazolate framework-90 (ZIF-90) with a controllable particle size. Chem Eng J 19: 11139–11142 https://doi.org/10.1002/chem.201301560 doi: 10.1002/chem.201301560
![]() |
[131] |
Tien-Binh N, Rodrigue D, Kaliaguine S (2018) In-situ cross interface linking of PIM-1 polymer and UiO-66-NH2 for outstanding gas separation and physical aging control. J Membr Sci 548: 429–438. https://doi.org/10.1016/j.memsci.2017.11.054 doi: 10.1016/j.memsci.2017.11.054
![]() |
[132] |
Vedrtnam A, Kalauni K, Dubey S, et al. (2020) A comprehensive study on structure, properties, synthesis, and characterization of ferrites. AIMS Mater Sci 7: 800–835. https://doi.org/10.3934/matersci.2020.6.800 doi: 10.3934/matersci.2020.6.800
![]() |
[133] |
Vendite AC, Soares TA, Coutinho K (2022) The effect of surface composition on the selective capture of atmospheric CO2 by ZIF nanoparticles: The case of ZIF-8. J Chem Inf Model 62: 6530–6543. https://doi.org/10.1021/acs.jcim.2c00579 doi: 10.1021/acs.jcim.2c00579
![]() |
[134] |
Railey P, Song Y, Liu T, et al. (2017) Metal-organic frameworks with immobilized nanoparticles: Synthesis and applications in photocatalytic hydrogen generation and energy storage. Mater Res Bull 96: 385–394. https://doi.org/10.1016/j.materresbull.2017.04.020 doi: 10.1016/j.materresbull.2017.04.020
![]() |
[135] |
Keskin S, van Heest TM, Sholl DS (2010) Can metal-organic framework materials play a useful role in large-scale carbon dioxide separations? ChemSusChem 3: 879–891. https://doi.org/10.1002/cssc.201000114 doi: 10.1002/cssc.201000114
![]() |
[136] |
Abraha YW, Tsai CW, Niemantsverdriet JWH, et al. (2021) Optimized CO2 capture of the zeolitic imidazolate framework ZIF-8 modified by solvent-assisted ligand exchange. ACS Omega 6: 21850–21860. https://doi.org/10.1021/acsomega.1c01130 doi: 10.1021/acsomega.1c01130
![]() |
[137] |
Karagiaridi O, Lalonde MB, Bury W, et al. (2012) Opening ZIF-8: A catalytically active zeolitic imidazolate framework of sodalite topology with unsubstituted linkers. J Am Chem Soc 134: 18790–18796. https://doi.org/10.1021/ja308786r doi: 10.1021/ja308786r
![]() |
[138] |
Jayachandrababu KC, Sholl DS, Nair S (2017) Structural and mechanistic differences in mixed-linker zeolitic imidazolate framework synthesis by solvent-assisted linker exchange and de novo routes. J Am Chem Soc 139: 5906–5915. https://doi.org/10.1021/jacs.7b01660 doi: 10.1021/jacs.7b01660
![]() |
[139] |
Wang P, Liu J, Liu C, et al. (2016) Electrochemical synthesis and catalytic properties of encapsulated metal clusters within zeolitic imidazolate frameworks. Chem Eur J 22: 16613–16620. https://doi.org/10.1002/chem.201602924 doi: 10.1002/chem.201602924
![]() |
[140] |
Phan A, Doonan CJ, Uribe-Romo FJ, et al. (2010) Synthesis, structure, and carbon dioxide capture properties of zeolitic imidazolate frameworks. Acc Chem Res 43: 58–67. https://doi.org/10.1021/ar900116g doi: 10.1021/ar900116g
![]() |
[141] |
Marti AM, Wickramanayake W, Dahe G, et al. (2017) Continuous flow processing of ZIF-8 membranes on polymeric porous hollow fiber supports for CO2 capture. ACS Appl Mater Interfaces 9: 5678–5682. https://doi.org/10.1021/acsami.6b15619 doi: 10.1021/acsami.6b15619
![]() |
[142] |
Chen B, Bai F, Zhu Y, et al. (2014) A cost-effective method for the synthesis of zeolitic imidazolate framework-8 materials from stoichiometric precursors via aqueous ammonia modulation at room temperature. Microporous Mesoporous Mater 193: 7–14. https://doi.org/10.1016/j.micromeso.2014.03.006 doi: 10.1016/j.micromeso.2014.03.006
![]() |
[143] |
Lai LS, Yeong YF, Lau KK, et al. (2016) Effect of synthesis parameters on the formation of ZIF-8 under microwave-assisted solvothermal conditions. Procedia Eng 148: 35–42. https://doi.org/10.1016/j.proeng.2016.06.481 doi: 10.1016/j.proeng.2016.06.481
![]() |
[144] |
Jiang S, Liu J, Guan J, et al. (2023) Enhancing CO2 adsorption capacity of ZIF-8 by synergetic effect of high pressure and temperature. Sci Rep 13: 1–10. https://doi.org/10.1038/s41598-023-44960-4 doi: 10.1038/s41598-023-44960-4
![]() |
[145] |
Pouramini Z, Mousavi SM, Babapoor A, et al. (2023) Effect of metal atom in zeolitic imidazolate frameworks. Catalysts 13: 155. https://doi.org/10.3390/catal13010155 doi: 10.3390/catal13010155
![]() |
[146] |
Latrach Z, Moumen E, Kounbach S, et al. (2022) Mixed-ligand strategy for the creation of hierarchical porous ZIF-8 for enhanced adsorption of copper ions. ACS Omega 7: 12345–12354. https://doi.org/10.1021/acsomega.2c00980 doi: 10.1021/acsomega.2c00980
![]() |
[147] |
Hu J, Liu Y, Liu J, et al. (2017) Effects of water vapor and trace gas impurities in flue gas on CO2 capture in zeolitic imidazolate frameworks: The significant role of functional groups. Fuel 200: 244–251. https://doi.org/10.1016/j.fuel.2017.03.079 doi: 10.1016/j.fuel.2017.03.079
![]() |
[148] |
Liu Y, Kasik A, Linneen N, et al. (2014) Adsorption and diffusion of carbon dioxide on ZIF-68. Chem Eng Sci 118: 32–40. https://doi.org/10.1016/j.ces.2014.07.030 doi: 10.1016/j.ces.2014.07.030
![]() |
[149] |
Liu Y, Liu J, Chang M, et al. (2012) Theoretical studies of CO2 adsorption mechanism on linkers of metal–organic frameworks. Fuel 95: 521–527. https://doi.org/10.1016/j.fuel.2011.09.057 doi: 10.1016/j.fuel.2011.09.057
![]() |
[150] |
Gu C, Liu Y, Wang W, et al. (2021) Effects of functional groups for CO2 capture using metal-organic frameworks. Front Chem Sci Eng 15: 437–449 https://doi.org/10.1007/s11705-020-1961-6 doi: 10.1007/s11705-020-1961-6
![]() |
[151] |
Yang F, Ge T, Zhu X, et al. (2022) Study on CO2 capture in humid flue gas using amine-modified ZIF-8. Sep Purif Technol 287: 120535. https://doi.org/10.1016/j.seppur.2022.120535 doi: 10.1016/j.seppur.2022.120535
![]() |
[152] |
Ji Y, Liu X, Li H, et al. (2023) Hydrophobic ZIF-8 covered active carbon for CO2 capture from humid gas. J Ind Eng Chem 121: 331–337. https://doi.org/10.1016/j.jiec.2023.01.036 doi: 10.1016/j.jiec.2023.01.036
![]() |
[153] |
Xu H, Cheng W, Jin X, et al. (2013) Effect of the particle size of quartz powder on the synthesis and CO2 absorption properties of Li4SiO4 at high temperature. Ind Eng Chem Res 52: 1886–1891. https://doi.org/10.1021/ie301178p doi: 10.1021/ie301178p
![]() |
[154] |
Li Z, Cao Z, Grande C, et al. (2021) A phase conversion method to anchor ZIF-8 onto a PAN nanofiber surface for CO2 capture. RSC Adv 12: 664–670. https://doi.org/10.1039/D1RA06480K doi: 10.1039/D1RA06480K
![]() |
[155] |
Åhlén M, Jaworski A, Strømme M, et al. (2021) Selective adsorption of CO2 and SF6 on mixed-linker ZIF-7–8s: The effect of linker substitution on uptake capacity and kinetics. Chem Eng J 422: 130117. https://doi.org/10.1016/j.cej.2021.130117 doi: 10.1016/j.cej.2021.130117
![]() |
[156] |
Asadi E, Ghadimi A, Hosseini SS, et al. (2022) Surfactant-mediated and wet-impregnation approaches for modification of ZIF-8 nanocrystals: Mixed matrix membranes for CO2/CH4 separation. Microporous Mesoporous Mater 329: 111539. https://doi.org/10.1016/j.micromeso.2021.111539 doi: 10.1016/j.micromeso.2021.111539
![]() |
[157] |
Zhang H, Duan C, Li F, et al. (2018) Green and rapid synthesis of hierarchical porous zeolitic imidazolate frameworks for enhanced CO2 capture. Inorg Chim Acta 482: 358–363. https://doi.org/10.1016/j.ica.2018.06.034 doi: 10.1016/j.ica.2018.06.034
![]() |
[158] |
Martinez Joaristi A, Juan-Alcañiz J, Serra-Crespo P, et al. (2012) Electrochemical synthesis of some archetypical Zn2+, Cu2+, and Al3+ metal-organic frameworks. Cryst Growth Des 12: 3489–3498. https://doi.org/10.1021/cg300552w doi: 10.1021/cg300552w
![]() |
[159] |
Zhang X, Yuan N, Chen T, et al. (2022) Fabrication of hydrangea-shaped Bi2WO6/ZIF-8 visible-light responsive photocatalysts for degradation of methylene blue. Chemosphere 307: 135678. https://doi.org/10.1016/j.chemosphere.2022.135949 doi: 10.1016/j.chemosphere.2022.135949
![]() |
[160] |
Liu X, Gao F, Xu J, et al. (2016) Zeolite@mesoporous silica-supported-amine hybrids for the capture of CO2 in the presence of water. Microporous Mesoporous Mater 222: 113–119. https://doi.org/10.1016/j.micromeso.2015.10.006 doi: 10.1016/j.micromeso.2015.10.006
![]() |
[161] |
Sebastián V, Kumakiri I, Bredesen R, et al. (2007) Zeolite membrane for CO2 removal: Operating at high pressure. J Membr Sci 292: 92–97. https://doi.org/10.1016/j.memsci.2007.01.017 doi: 10.1016/j.memsci.2007.01.017
![]() |
[162] |
Erkartal M, Incekara K, Sen U (2022) Synthesis of benzotriazole functionalized ZIF-8 by postsynthetic modification for enhanced CH4 and CO2 uptakes. Inorg Chem Commun 142: 109696. https://doi.org/10.1016/j.inoche.2022.109696 doi: 10.1016/j.inoche.2022.109696
![]() |
[163] |
Bolotov VA, Kovalenko KA, Samsonenko DG, et al. (2018) Enhancement of CO2 uptake and selectivity in a metal–organic framework by the incorporation of thiophene functionality. Inorg Chem 57: 5074–5082. https://doi.org/10.1021/acs.inorgchem.8b00138 doi: 10.1021/acs.inorgchem.8b00138
![]() |
[164] |
Shi GM, Yang T, Chung TS (2012) Polybenzimidazole (PBI)/zeolitic imidazolate frameworks (ZIF-8) mixed matrix membranes for pervaporation dehydration of alcohols. J Membr Sci 415–416: 577–586. https://doi.org/10.1016/j.memsci.2012.05.052 doi: 10.1016/j.memsci.2012.05.052
![]() |
[165] |
Yang T, Chung TS (2013) High performance ZIF-8/PBI nano-composite membranes for high temperature hydrogen separation consisting of carbon monoxide and water vapor. Int J Hydrogen Energy 38: 229–239. https://doi.org/10.1016/j.ijhydene.2012.10.045 doi: 10.1016/j.ijhydene.2012.10.045
![]() |
[166] |
Abdelhamid HN, Mathew AP (2021) In-situ growth of zeolitic imidazolate frameworks into a cellulosic filter paper for the reduction of 4-nitrophenol. Carbohydr Polym 274: 118657. https://doi.org/10.1016/j.carbpol.2021.118657 doi: 10.1016/j.carbpol.2021.118657
![]() |
[167] |
Ding M, Flaig RW, Jiang HL, et al. (2019) Carbon capture and conversion using metal–organic frameworks and MOF-based materials. Chem Soc Rev 48: 2783–2828. https://doi.org/10.1039/C8CS00829A doi: 10.1039/C8CS00829A
![]() |
[168] |
Liu D, Ma X, Xi H, et al. (2014) Gas transport properties and propylene/propane separation characteristics of ZIF-8 membranes. J Membr Sci 451: 85–93. https://doi.org/10.1016/j.memsci.2013.09.029 doi: 10.1016/j.memsci.2013.09.029
![]() |
[169] |
Reza Abbasi A, Moshtkob A, Shahabadi N, et al. (2019) Synthesis of nano zinc-based metal–organic frameworks under ultrasound irradiation in comparison with solvent-assisted linker exchange: Increased storage of N2 and CO2. Ultrason Sonochem 59: 104729. https://doi.org/10.1016/j.ultsonch.2019.104729 doi: 10.1016/j.ultsonch.2019.104729
![]() |
[170] |
Hu Z, Zhang H, Zhang XF, et al. (2022) Polyethylenimine grafted ZIF-8@cellulose acetate membrane for enhanced gas separation. J Membr Sci 662: 120996. https://doi.org/10.1016/j.memsci.2022.120996 doi: 10.1016/j.memsci.2022.120996
![]() |
1. | Eugene B. Postnikov, Anant Pratap Singh, Alexander V. Sychev, Anastasia I. Lavrova, Vineet Kumar Singh, A stochastic model for the bacterial growth exhibiting staged growth, desynchronization, saturation and persistence, 2024, 378, 00255564, 109322, 10.1016/j.mbs.2024.109322 | |
2. | Rushna Wazahat, Strategic diagnosis- Unraveling Tuberculosis- A comprehensive approach, 2024, 00195707, 10.1016/j.ijtb.2024.08.004 | |
3. | Nathan Mugenyi, Nelson Ssewante, Joseph Baruch Baluku, Felix Bongomin, Mutuku Mukenya Irene, Alfred Andama, Pauline Byakika-Kibwika, Innovative laboratory methods for improved tuberculosis diagnosis and drug-susceptibility testing, 2024, 1, 2813-7868, 10.3389/ftubr.2023.1295979 | |
4. | Arifatun Nasicha, Ni Made Mertaniasih, Eko Budi Koendhori, Pepy Dwi Endraswari, Ariani Permatasari, , Xpert® MTB/RIF Ultra Accuracy in Pulmonary TB Diagnosis: Comparative Analysis with Mycobacterial Growth Indicator Tube 960 System, 2025, 19, 09737510, 1134, 10.22207/JPAM.19.2.16 |