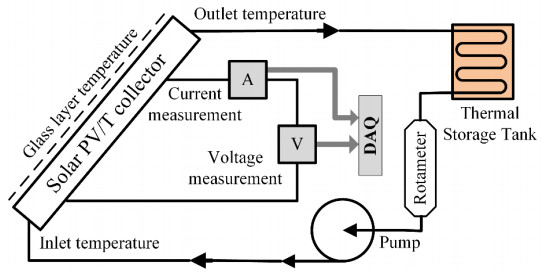
Citation: Ibraheem Ahmad Qeays, Syed Mohd. Yahya, M. Saad Bin Arif, Azhar Jamil. Nanofluids application in hybrid Photovoltaic Thermal System for Performance Enhancement: A review[J]. AIMS Energy, 2020, 8(3): 365-393. doi: 10.3934/energy.2020.3.365
[1] | Kaovinath Appalasamy, R Mamat, Sudhakar Kumarasamy . Smart thermal management of photovoltaic systems: Innovative strategies. AIMS Energy, 2025, 13(2): 309-353. doi: 10.3934/energy.2025013 |
[2] | Wei An, Yifan Zhang, Bo Pang, Jun Wu . Synergistic design of an integrated pv/distillation solar system based on nanofluid spectral splitting technique. AIMS Energy, 2021, 9(3): 534-557. doi: 10.3934/energy.2021026 |
[3] | Fadhil Khadoum Alhousni, Firas Basim Ismail, Paul C. Okonkwo, Hassan Mohamed, Bright O. Okonkwo, Omar A. Al-Shahri . A review of PV solar energy system operations and applications in Dhofar Oman. AIMS Energy, 2022, 10(4): 858-884. doi: 10.3934/energy.2022039 |
[4] | Nadwan Majeed Ali, Handri Ammari . Design of a hybrid wind-solar street lighting system to power LED lights on highway poles. AIMS Energy, 2022, 10(2): 177-190. doi: 10.3934/energy.2022010 |
[5] | Alemayehu T. Eneyaw, Demiss A. Amibe . Annual performance of photovoltaic-thermal system under actual operating condition of Dire Dawa in Ethiopia. AIMS Energy, 2019, 7(5): 539-556. doi: 10.3934/energy.2019.5.539 |
[6] | Ruaa Al Mezrakchi . Investigation of various hybrid nanofluids to enhance the performance of a shell and tube heat exchanger. AIMS Energy, 2024, 12(1): 235-255. doi: 10.3934/energy.2024011 |
[7] | Virendra Sharma, Lata Gidwani . Recognition of disturbances in hybrid power system interfaced with battery energy storage system using combined features of Stockwell transform and Hilbert transform. AIMS Energy, 2019, 7(5): 671-687. doi: 10.3934/energy.2019.5.671 |
[8] | Zeid Al Qaisi, Qais Alsafasfeh, Ahmad Harb . Stability impact of integrated small scale hybrid (PV/Wind) system with electric distribution network. AIMS Energy, 2018, 6(5): 832-845. doi: 10.3934/energy.2018.5.832 |
[9] | Aaron St. Leger . Demand response impacts on off-grid hybrid photovoltaic-diesel generator microgrids. AIMS Energy, 2015, 3(3): 360-376. doi: 10.3934/energy.2015.3.360 |
[10] | Virendra Sharma, Lata Gidwani . Optimistic use of battery energy storage system to mitigate grid disturbances in the hybrid power system. AIMS Energy, 2019, 7(6): 688-709. doi: 10.3934/energy.2019.6.688 |
The escalation of world's population has led to an increased demand of resources including water, electricity, and housing [1]. Consumption of fossil fuels has also increased in order to meet the human demands resulting in environmental degradation [2,3]. Scientists and researchers are putting their efforts to look after some alternate eco-friendly/renewable sources of energy which are economical and less polluting. One such clean source of energy, i.e., olar energy can be harnessed in two possible ways. Thermal energy coming from the sun is harnessed by constructing solar collectors with heat absorbers like water or any other fluid. Light energy can be harnessed by semiconductor photovoltaic panels which convert light energy directly to DC current. Solar radiation is an energy source with tremendous potential for developing countries, particularly the ones located in temperate and tropical zones [4]. PV cells have a wide range of application and are clean and eco-friendly source for power production [5]. Photovoltaic systems have various advantages such as independent operation, reliable long life, no fuel and fumes, easy installation low maintenance, and low recurrent costs as compared to other sources [6]. However the PV systems tend to get heated due to non-direct absorption with continuous operation which obstructs the efficiency of PV system. This absorbed heat results in increase of the PV cell temperature [7,8]. A non-inflammable fluid is generally chosen which flows beneath the PV panel and acts as a heat exchanger. It absorbs the solar thermal energy directly, reducing the heat loss and hence enhancing the thermal efficiency. The system formed in this way is called a photovoltaic thermal (PV/T) system, which can supply electrical and thermal energy simultaneously [9,10,11]. The conventional fluids like air and water which are used in hybrid PV/T system are not much effective due to low thermal conductivity and low heat carrying capacity [12,13,14,15,16]. Thus efforts are being made to synthesize an ideal heat exchange fluid for these systems. Different compositions and types of nanofluids are also being employed in these systems due to their high thermal conductivity. Some researchers have used PCM based cooling of PVT system however, due to lower heat removal capability nano-enhanced PCM is used to improve the performance of PVT system.
The aim of the present review paper is to present an extensive literature survey on the applications on nanofluids and PCM in a PV/T system. The authors have also tried to configure in detail the effects of properties of nanofluids and PCM on the thermal, electrical and overall efficiencies associated with PV/T system. Furthermore efforts were made to summarise the nanofluid as a coolant an optical filter alongwith nanoflim, their merits and demerits. We have also proposed some possible modifications which can be done for hybrid Photovoltaic system to achieve maximum electrical efficiency of PV panel and thermal efficiency of the Nano fluids and PCM.
Use of conventional fluids like air and water in PV/T systems limits the amount of heat that can be transferred from the panel. Researchers have tried to eliminate this shortcoming by using nanofluids and nano-PCM as a heat transfer carrier with higher thermal conductivities [17,18,19]. Nanoparticles due to their smaller size can pass through pumps and plumbing without causing any wear and erosion in contrast to the micron-sized particles [20,21,22,23,24,25].
An extensive literature survey showed that the impending roles in which nanofluids are used as a coolant or as an optical filter in the hybrid PV/T systems. These primary classifications are further divided into sub categories based on the simulative work and experimental work and the summary is stated in the tabular form.
Majority of researchers have focused on lowering the PV cell temperature and utilizing the excess heat by employing nanofluid as a coolant on the back side of the PV panel. Al-Shamani et al. [26] used different types of nanofluids (SiO2, TiO2 and SiC) in the PV/T system. The experimental results showed that the PV/T collector with SiC nanofluid has the highest PV/T electrical efficiency of 13.52% and combined photovoltaic thermal (PV/T) efficiency of 81.73% was recorded at a mass flow rate of 0.170 kg/s and insolation of 1000 W/m2. Yun and Qunzhi [27] prepared MgO-water nanofluid has been and applied over the silicon PV panel in order to reduce the heat of solar cells. The transmittance of light, temperature of solar cells, optical properties and temperature dropped from inlet to outlet and output power of solar cells had been compared for different mass concentration of fluid produced. The particle size of the nanofluid used was approximately 10 nm and the concentrations taken were 0.02 t%, 0.06 t% and 0.1 t%. The designed PV/T system having a 2 mm thick liquid layer had efficiency above 60%. Also, there was no gain in electrical efficiency if compared to that of traditional PV system but overall efficiency achieved was enhanced. Michael and Iniyan [28] used CuO/water nanofluid in hybrid PV/T system. They observed that 0.05% volume fraction of CuO/water nanofluid made a remarkable improvement in the thermal efficiency up to 45.76% compared to water.
Rejeb et al. [29] studied the performance of a nanofluid based photovoltaic thermal (PV/T) system. Different types of nanoparticles (Al2O3 and Cu) dispersed in different based fluids (pure water and ethylene glycol) with varying concentration were used. The best thermal and electrical efficiency was attained by using Cu/water in the place of Cu/ethylene glycol, and same as for Al2O3/water in comparison with Al2O3/ethylene glycol. Sardarabadi et al. [30] used silica/water nanofluid as a coolant and studied its effect on the thermal and electrical efficiencies of a photovoltaic thermal system. Silica nanoparticles of 11–14 nm diameter were mixed with water in two concentrations, i.e., 1% and 3% by weight. A thin copper tube was attached to the solar cells in which the coolant was circulated at constant mass flow rates. The experiment results exhibited that the thermal efficiency of the system was increased considerably when nanofluid was used as a coolant. There was an increase of 7.6% in thermal efficiency for nanofluid with 1% wt and for 3% wt the thermal efficiency was increased by 12.8%. It was also observed that the overall efficiency was also upgraded with the use of nanofluid, for 1% wt the increase was 3.6% whereas it goes to 7.9% in case of 3% wt.
Al-Waeli et al. [31] observed that by adding the 3% wt of SiC nanofluids with water increases the fluid density and viscosity by 0.0082% and 1.8% respectively. The electrical efficiency was increased up-to 24.1%.The overall efficiency of the PV/T system which was compared to the PV system was about 88.9% by using 3% wt of SiC as a nanofluid. Jing et al. [32] used Silica/water nanofluids to study the optimal operational condition for PV/T system. The particle sizes of nanofluids were 5 nm, 10 nm, 25 nm and 50 nm. When concentration level was 40 and at flow rate of 0.015 m/sec, the enhancement in the exergetic efficiency of PV/T system was 7.0% as compared to that of DI water. When the concentration was 100 and at optimum flow rate of 0.1 m/sec, exergetic efficiency was enhanced by 9.5%.
Radwan et al. [33] studied novel cooling strategy for low concentrated photovoltaic thermal system by introducing a micro channel heat sink with different nanofluids (Al2O3 and SiC) at different concentration ratio. Then the optimization of the results was made on different concentration levels of the nanofluids and influence of mass flow rate of the cooling fluid was also analysed. Silicon Carbide (SiC) was found more effective than aluminium oxide (Al2O3–water) nanofluid as higher reduction in the temperature was found in the case of the SiC- nanofluid water. The results showed a drop in the temperature of the cell to 38 ℃ and electrical efficiency improved to 19% while using nanofluids. Karami and Rahimi et al. [34] studied the behaviour of the water based nanofluid with small amount of Boehmite in the PV/T system. They made two different configurations, one was straight channel and other was helical channel based.The performance of the water based nanofluid containing boehmite was evaluated and it was found that the nanofluid increased the efficiency of the PV/T system as compared to that of the water based system. The temperature drop was higher in case of nanofluid as compared to water. The electrical efficiencies of both the systems were 20.57% and 37.67% for straight and helical respectively. Thus the helical configuration with Boehmite nanofluid was a better option to increase the performance of the PV/T system.
Hussien et al. [35] investigated the thermal and electrical efficiency of the PV/T system by using nanofluid as the coolant. They constructed a cooling water technique and studied the effects of the nanofluid by using different concentration ratios on the system. The base fluid used in the experiment was water and Al2O3 nanoparticles of size 30 nm were used in five different concentrations ratios as (0.1%, 0.2%, 0.3%, 0.4%, and 0.5%). The results showed that when nanofluid is used as the coolant the thermal and electrical efficiencies were increased considerably. They concluded that for an optimum concentration ratio 0.3% of the nanofluid, the electrical efficiency increased to 12.1% and thermal efficiency increased to 34.4%. Al-Waeli et al. [36] studied the effect of nanofluids in increasing efficiency of PV/T system. The nanofluids used were Al2O3, CuO and SiC each for concentration of 0.5 vol%, 1 vol%, 2 vol%, 3 vol% and 4 vol% using water as base fluid. It was seen that the SiC provided the best thermal efficiency among all of these nanofluids. The diameter of nanoparticles of Al2O3, CuO and SiC were around 30-60 nm, 35–45 nm and 45–65 nm respectively. For 4 vol% for each case, the thermal efficiency enhanced by 1.96%, 3.42% and 4.8% by using nanofluid Al2O3, CuO and SiC respectively.
Sardarabadi et al. [37] investigated the effect of Al2O3, TiO2 and ZnO nanofluids with water as base fluid in PV/T system. The concentration taken for each nanofluid is 0.2 wt%. The diameter of nanoparticle Al2O3 was 20 nm. The particle size for that of TiO2was about 10–30 nm and for ZnO it was 10–25 nm. The enhancement achieved in electrical efficiency for deionized water, TiO2, ZnO and Al2O3 was about 5.48%, 6.54%, 6.46% and 6.36% respectively. Bellos and Tzivanidis [38] examined the impact of nanofluid on the efficiencies of a hybrid PV/T system. The nanofluid examined was Cu/water and the results were compared to that those with the water based system. They examined the hybrid PV/T system with different storage tanks, the reason for using the storage tanks was the effect of storage on thermal output. The result of the experiment showed that for 150 L storage tank when Cu/water nanofluid was used the electrical efficiency enhanced by 1.49% and the thermal efficiency was increased by 4.35%. They also found that the mean yearly thermal efficiency for Cu nanofluid obtained was 43.8% which was 42% in case of water and the mean yearly electrical efficiency increased from 12.4% to 12.5% when the results were compared with water.
Lari et al. [39] experimentally investigated the effects of nanofluids as a coolant on the performance of PV system. The nanoparticle used in the experiment was silver with particle size 10 nm, the concentration of particles taken was 0.5% vol and was mixed into the deionized water forming Ag/water nanofluid. The results of the experiment showed that there was an increase in electrical output by 8.5% and an increase in thermal output by 13% when the performance was compared to the watercooled system. Sardarbadi et al. [40] investigated the impact of nanofluid as a coolant on the PV thermal system and its effect on the efficiencies associated with the system. In the experiment ZnO nanoparticles of size 35–45 nm were suspended in deionized water, the concentration of the nanofluid taken was 0.2% wt. The results showed an increase of 13% in the electrical efficiency and an increase of 5% in thermal efficiency as compared to the conventional PV module. Al-Shamani et al. [41] experimentally observed the performance of a PV thermal system by using the nanofluid as a heat exchanging medium. The thermo-physical properties of the nanofluid such as thermal conductivity, viscosity and density etc. were determined and their effects on the PV thermal systems were observed.The nanofluid used in the experiment was SiC. The experiment concluded that the electrical efficiency of the system was enhanced from 8.77% to 13.52% and the overall efficiency of the system was increased from 8.4% to 13.1% when nanofluid was used in place of the conventional fluids.
Hasan et al. [42] performed an experiment that studied the effects of the nanofluid on the electrical, thermal and overall efficiency of the system. The setup consists of 36 nozzles and 4 parallel tubes. The nozzles inject the nanofluid on the back of PV system. Three nanofluids were used in the experiment (SiC, TiO2 and SiO2) and the base fluid used was water. The results depicted that the SiC/water nanofluid showed the highest efficiency. The electrical, thermal and overall efficiency of the system in case of SiC nanoparticle was documented 12.75%, 85% and 97.75% respectively. Gangadevi et al. [43] investigated experimentally the effect of Al2O3/water nanofluid of 1% wt and 2% wt by circulating in a PV/T system on the thermal, electrical and overall efficiencies with the flow rate of 40 L/H. The results showed that by using 2% wt of Al2O3 with water caused an enhancement in the electrical efficiency and thermal efficiency by 13% and 45% and the overall efficiency was up to 58% as compared to water based PV/T system. Chandrasekar et al. [44] compared the electrical and thermal efficiency of the PV/T system using water, Al2O3/water nanofluid and CuO/water and also compared their efficiency when no cooling fluid was used. The maximum temperature reached was dropped by 11% and 17% when cooled by CuO nanofluid and Al2O3 nanofluid respectively when compared to that when without cooling fluid. The maximum electrical efficiency when no cooling was provided was 9% and which was increased to 9.4% and 9.8% when cooling fluid CuO nanofluid and Al2O3 nanofluid respectively was used.
Many types of NPs from different materials (metallic, oxide, etc.) with various structures (homogeneous, core–shell, etc.), sizes, shapes, plasmonic and thermo-optical properties were investigated for absorption of solar radiation. Nanomaterials in the form of either nanofluids (nanoparticle suspended fluids), nanofilms and nanowires have also been widely investigated and applied to emerging spectral-splitting technologies owing to their unique optical properties. An optical filter absorbed the infrared and ultraviolet components of the visible light spectrum and hence increases the temperature of the nanofluid. An et al. [45] developed a concentrating PV/T collector with fluid based spectral splitting filter. The oleyl amine solution of Cu9S5 nanoparticle was implemented in the filter to produce the moderate-temperature heat in the outdoor condition and was also used to investigate the performance of the collector. The results showed that the PV efficiency of Si cell itself improved when the Cu9S5 nano fluid was employed as the optical filter. The experimental maximum overall efficiency of the PV/T collector was about 34.2%, which was 17.9% more than the PV collector having no filter. The schematics of their setup are shown below. Xu and Kleinstreuer [46] studied use of SiO2 nanoparticle in a CPV/T system. They made a new design of dual concentration photovoltaic thermal system (CPV/T) via optical techniques that increased both thermal and electrical efficiencies of the system and reduction in the solar cell material usage was also achieved. They made a new thermal conductivity model for nanofluids and then efficiencies of the system were evaluated at different climatic conditions. The results obtained showed increased efficiency of the system when temperature of the solar cell was 62 ℃ the overall efficiency of the system reached 70% and electrical efficiency was found to be 11% whereas the thermal efficiency was 59% while using nanofluids in the CPV/T system.
Hjerrild et al. [47] designed a system which was composed of suspended core–shell Ag–SiO2 nano discs and carbon nanotubes (CNTs) in water. By using Ag-SiO2 as a nanofluid in the PV/T system caused an enhancement in the electrical efficiency of 18% compared to the water cooled PV/T System and the maximum enhancement in the thermal efficiency with compared to the water cooled is 59%. The increase in overall efficiency was about 30% on using the Ag-SiO2 as a nanofluid with a concentration of 0.026 wt%. Otanicar et al. [48] investigated that for a PV/thermal system using mild thermal connection could lead to increase in the efficiency. In this design, a fluid filter absorbed energy directly in the fluid below the band gap. By using the above system, it was found that the combined efficiency had increased up to 38%. Taylor et al. [49] used different PV cells such as InGaP, CdTe, InGaAs, Si, and Ge and provide an optimization on nanofluid based PV/T system by using water and therminol VP-1 as a base fluid with SiO2. For InGaP, CdTe and InGaAs water was used as a base fluid and for Si and Ge therminol VP-1 was used as a base fluid. By using water and therminol VP-1 as a base fluid with SiO2 caused an 4%–8% enhancement in the efficiency of the PV/T system as compared to the water based PV/T system. Hassani et al. [50] simulated the thermal and electrical efficiencies of different collector configurations, working fluids and solar concentrations. Their results showed that PV-T collectors with a nanofluid filter can delivered higher-grade heat and a shorter payback time compared to conventional PV-T collector designs.
Nanofilm-based filters are generally used to provide a selectively reflective effect in which only a part of the solar spectrum can pass through the filter, while the rest is reflected. Otanicar et al. [51] developed hybrid PV/T collectors. They investigated the theoretical performance of the optical filter in the form of thin film of nanofluid in hybrid PV/T system. The TiO2/SiO2 nanofilm filter was also investigated by Dorodnyy et al. [52], who designed a 60-layer irregular TiO2/SiO2 structure positioned over Si solar cells. The optimized thickness of the different layers was, again, in the range from 10 to 110 nm. The nanofilm-based selectively reflective thin-film layer can also been applied to multi-junction solar cells without a thermal absorber. For example, a significantly higher electrical efficiency of the multi-junction solar cells was achieved with the help of a selectively reflective thin-film layer in Refs. [53]. Nevertheless, a few investigators have reported on the long-term performance of nanofilm-based filters, which needs further investigation in future work. The nanoparticle based fluid filters system has 4% higher thermal efficiency than conventional thin film-based optical fluid filters.
The cost of spectral-splitting filters is also an important selection criterion in commercial applications. Solid filters are usually proposed for CPV-T collectors to save material and the associated costs. Fluid filters are more common in the literature and cheaper than solid filters. Water is the most common and affordable liquid filter which has a high absorptivity in the IR. Nanofluids are the most expensive liquid filters, followed by synthetic oils and glycols. A complete economic analysis is necessary in spectral-splitting filter screenings and downstream selection and optimization for PV-T applications. the economic analysis conducted by Liang et al. [54] reported a payback time of about 22 years for a PV-T system using SiO2/TiO2 interference thin films. Some researchers suggest that nanofluids can be inexpensive (<$1/L) [55], however, in general the cost of nanofluids depends highly on the material, size and concentration. As large-scale implementation of nanomaterials has not yet been realized in PV-T applications, there is insufficient data and information available for evaluating the cost of nanomaterials. In either case, further work is required in this space for further cost reduction, prototype demonstration and to understand the full cost implications of deployment.
Khanjari et al. [56] considered pure water and Alumina-water nanofluid as coolant fluids for CFD simulation. The proposed model considered conduction and convection heat transfer mechanisms by including a water riser tube and an absorber plate. The result showed that by increasing the volume fraction of nanoparticles the efficiency and heat transfer coefficient was enhanced. The maximum increase percentage of heat transfer coefficient for alumina-water was 12%. For alumina-water nanofluid, increasing the heat transfer coefficient with respect to the inlet fluid velocity at volume fraction 5% was in the range of 8–10% as comparison to pure water.
Xu and Kleinstreuer [57] performed computational study to scrutinize the thermal heat transfer carried out by Al2O3—water as cooling fluid in the PV cell that are closely packed. They altered various specifications such as volume fraction of nanofluid, inlet temperature, inlet Reynolds number and different channel heights. The system efficiency achieved was 20%. However, the efficiency can further be optimized by lowering the inlet temperature of nanofluid. Al Waeli et al. [58] performed technical assessment of nanofluids in a GCPV/T system. The system was installed and data was evaluated for power and efficiency. They used MATLAB software for the simulations and evaluated the electrical performance of the system. The results of the experiment showed that by using nanofluids the efficiency of the GCPV/T system was improved to 9.1%.
Hassani et al. [50] studied the effects of nanofluids as a coolant and as an optical filter on the PV/T system. In the experiment, they used two designs GaAs and Si fabricated PV cells at different concentration ratios from 0.001% to 1.5%.They obtained the results that by using nanofluid as coolant the electrical efficiency for GaAs cells and Si cells was increased by 9% and 5.6% respectively and the overall efficiency of the system with GaAs cells and Si cells was improved from 24.2% to 30% and from 19.8% to 24.4% respectively. Saroha et al. [59] proposed a model in which nanofluid act as heat transfer carrier as well as optical filter. In this paper, they designed a novel PV/T hybrid collector by using gold and silver nanoparticles on a silicon PV cell. By performing this experiment they analysed that nanoparticles represent feasible and viable multifunctional media in PV/T solar systems. Zhao et al. [60] designed a PV/T system in which the infrared radiation was absorbed by working fluid and remaining visible light was transferred to the PV system. The quantity of infrared absorbed by thermal unit was about 89% and the conversion of visible light into electricity was about 84%. The results showed that infrared radiation caused an enhancement in the electrical efficiency about 9.6%, thermal efficiency about 40% and the exergetic efficiency of the system was increased up to 22%.
From the above discussion, it is clear that almost all the authors either experimentally or numerically have concentrated their research on limited types of nanoparticles such as SiC, Al2O3, TiO2, MgO, Cu, Au, Ag, ZnO, SiO2 and Ag-SiO2. Nevertheless, carbon-based nanoparticles such as multiwalled carbon nanotubes (MWCNTs), Hybrid nanofluid, graphene and MXenes are yet to be investigated. These novel nanofluids can further improve the PVT performance and create avenues for future research.
Phase change material which stores energy in form of latent heat during which it changes its phase from solid to liquid is a well-known passive technique. To store large amount of energy, PCM must have high latent heat [61]. PCMs are classified into inorganic salt hydrates, organic fatty acids and eutectics. These are mostly used for cooling, heating and energy storage purposes due to availability of various phase transition temperatures and high latent heat [62]. Zondag et al. [63] used PCM for thermal energy storage in lab scale prototype for industries at different temperatures and flow rates. It was concluded that temperature difference had a strong impact on the performance of PCM compared to flow rates while considering natural convection. Wang et al. [64] presented inorganic salts and metal alloy in a novel dual PCM thermal energy storage system. Metal PCM was encapsulated over tubes which were inserted in inorganic salts to increase its charging and discharging time. Simulation resulted in quick transmission of heat from tubes to inorganic salts. However, it was concluded that metal PCM is better than solid PCM in terms of heat storage capacity and reinforcement.
Raul et al. [65] employed encapsulated PCM for latent heat thermal energy storage in solar energy applications. It was observed as temperature of heat transfer fluid increases, energy stored in PCM rises. When temperature of fluid decreases, heat is transferred from PCM to fluid thus, resulting in higher thermal efficiency. Maximum efficiency of 75.7% was obtained at 8.2 lpm flow rate with 180 and 120 ℃ inlet heat transfer fluid temperatures during charging and discharging respectively. Mousavi et al. [66] explained key parameters affecting PV-PCM system using Paraffin C18, Paraffin C22, Pal/Cap acid, salt and Paraffin C15. Best performance was showed by Paraffin C22 with 83% thermal efficiency; however, electrical efficiency was enhanced with all PCMs. Su et al. [67] stated that increase in thickness of PCM layer results in higher thermal capacity but gives lower heat transfer coefficient between PCM layer and PV panel. For a PCM of 40 ℃ melting temperature, optimal layer thickness should be 3.4 cm to obtain maximum overall energy.
Enhancement in electrical and thermal efficiency was further achieved by merging both active and passive cooling in a single module. Zhou et al. [68] performed simulation on PV/T with PCM system with water as working fluid. It was concluded that with the increase in mass flow rate and decrease in inlet fluid temperature results in improved electrical efficiency. Maximum reduction in temperature was from 70 ℃ to 30 ℃ at 0.25 kg/s flow rate. Yang et al. [69] compared performance of PV/T system with and without PCM experimentally. Thermal efficiency of PV/T and PV/T with PCM system was 63.93% and 76.87% respectively. Thus, addition of PCM to PV/T system resulted in better overall performance and minimum surface temperature.
In this section mathematical modelling adopted by various researchers are summarised in Table 1, with type of system and parameters evaluated for performance indication. Table 1, includes experimental study as well as study which resulted from combined numerical and experimental performance evaluation. Some authors focussed on increasing the overall efficiency as a combined parameter, some emphasis on electrical efficiency improvement and few were involved in increasing thermal efficiency. All the studies mentioned in Table 1, includes the uncertainty and error analysis and for they have used different approaches. However as far as electrical and thermal efficiency is concerned all the studies seems to follow the same trend. Some have considered packing factor some of them neglect it as it did not affect efficiency at larger scale. Fewer studies are available that includes friction factor through collector tubes. It was well established that moderate concentration of nanofluid will not affect the friction and hence pumping power to much extent. Therefore researchers neglect these parameters during study. In Table 2, only numerical studies related to PVT and CPVT system were summarised to give a better taste to audience for further development in this area. All the researchers followed the same methodology with little changes in symbols and presentation. However some studies discussed the exergetic efficiency also along with energy analysis. Few authors performed economic analysis also which is further help in development of prototype and cost optimisation. Apart from these All have discussed the thermal, electrical and overall efficiency of PVT and CPVT system.
Authors | Type of Study and System | Mathematical Modelling of the system | Parameters Evaluated |
Al-Shamani et al. [26] | ■ Experimental. ■ PV/T system having stainless steel tube absorber |
ηel=ηr(1−β(Tpm−Tr)) where, ηel is the electrical efficiency, ηr is the reference efficiency of the PV module, β is the temperature coefficient, Tpm is the temperature of the solar cells and Tr is the reference temperature. WR={(∂R∂x1w1)2+(∂R∂x2w2)2+…+(∂R∂xnwn)2}1/2 where, R is uncertainty - function of the independent variables x1, x2, xn and w1, w2, wn represents the uncertainties associated with independent variables. |
Electrical efficiency |
Yun and Qunzhi [27] | ■ Experimental ■ PV/T system |
ηpvt=ηth+ηe=Qu+PIs×At,Qu=˙mCP(To−Ti) ˙m is the mass flow rate, Is is the solar intensity, Ac is the collector area, Cp is the specific heat, To and Ti are outlet and inlet fluid temperatures respectively |
Overall efficiency |
Michael and Iniyan [28] | ■ Experimental and Numerical ■ Single rectangular absorber PV/T |
ηth=QuIs×At where, ηth is the thermal efficiency [(Uηlηl)2]thεrm=(U˙m˙m)2+(UGG)2+(UTT)2 where, U—denotes uncertainty, ˙m—mass flow rate of water, T—temperature difference between the outlet and inlet fluid and G—incident global solar radiation |
Thermal Efficiency |
Rejeb et al. [29] | ■ Experimental and Numerical ■ Sheet and tube PV/T |
Heat transfer modeling of collector was developed in FORTRAN language, based on the finite volume method. ηth=QuIs×At and ηel=ηo(1−β(Tpv−298)) [(Uηlηl)2]thεrm=(U˙m˙m)2+(UGG)2+(UTT)2 [(Uηiηl)2]elec=(UvV)2+(Ull)2+(UGG)2 where, V is voltage and I is current |
Thermal Efficiency Electrical Efficiency |
Sardarabadi et al. [30] | ■ Experimental study ■ Phase change material ■ Comparative Analysis |
ηpvt, is the overall efficiency which is the sum of the thermal and electrical efficiencies (ηth and ηel, respectively): ηpvt≅Eth+EelEin=>ηpvt=∫t2t1(AcE″th+ApvE″el)dtAc∫t2t1(Geff)dt=ηth+r.ηel where Ac—area of the collector, Apv—area of PV cells and r—packing factor. δR=√(δRδv1δv1)2+(δRδv2δv2)2+⋯+(δRδvnδvn)2 where δR is the uncertainty of function R. |
Thermal efficiency |
Al-Waeli et al. [31] | ■ Experimental study | The total efficiency (ηr)=the thermal efliciency+the electrical efficiency ηt=ηthermal+ηelectrical=Qu+PIs×At where, At the total area of the panel and collector. Change in the operations by: eR=[(∂R∂V1e1)2+(∂R∂V2e2)2+…+(∂R∂Vnen)2]0.5 where, eR: uncertainty in the results, R: a given function of the independent variables y1,y2,…,yΠ or R=R(V1,V2,…,Vn), ei: uncertainty interval in the nth variable. |
Electrical Thermal efficiency |
Radwan et al. [33] | ■ Experimental study | The solar cell generated electrical energy per unit area, thermal efficiency, friction factor and net produced power are calculated as follows: Psc=ηscβscτgG(t).CR, ηth=PthG.CR.Asc and Pfriction=ΔP.mρnf |
Thermal efficiency |
Karami and Rahimi et al. [34] | ■ Experimental study | The values of friction factor ω were calculated using the following equation: f=ΔpchDh2ρf.u2m.Lch where, Dch—hydraulic diameter. |
Electrical efficiency. |
Hussien et al. [35] | ■ Hybrid PVT systems. ■ Experimental study |
Hybrid PVT system overall efficiency is give as: ηtotal=ηth+ηele=mCp∫(To−Ti)dt+∫VIdtAo∫(t)dt |
Overall efficiency. |
Al-Waeli et al. [36] | ■ Photovoltaic thermal PV/T collectors | The total efficiency (ηr)=the thermal efliciency+the electrical efficiency ηt=ηthermal+ηelectrical=Qu+PIs×At where At—the total area of the panel and collector. eR=[(∂R∂V1e1)2+(∂R∂V2e2)2+…+(∂R∂Vnen)2]0.5 where, eR: uncertainty in the results, R: a given function of the independent variables y1,y2,…,yΠ or R=R(V1,V2,…,Vn) and ei: uncertainty interval in the nth variable. |
Overall system efficiency |
Sardarabadi et al. [37] | ■ Experimental and Numerical study | Electrical efficiency of PVT system can be estimated as ηclcc=Ee1ecEin=Voc×Jsc×FFGeffective where V—open circuit voltage and Isc—short circuit current and FF—Fill factor eR=[(∂R∂V1e1)2+(∂R∂V2e2)2+…+(∂R∂Vnen)2]0.5 where, eR: uncertainty in the results, R: a given function of the independent variables y1,y2,…,yΠ or R=R(V1,V2,…,Vn), ei: uncertainty interval in the nth variable. |
Electrical efficiency |
Bellos et al. [38] | ■ Experimental study ■ Performance Analysis |
The total exergy based on daily solar irradiation (Zs) is evaluated using following model: Zs=Ac.HT.(1−43.(TamTsun)+13.(TamTsun)4) |
Thermal efficiency Electrical efficiency |
Sardarbadi et al. [40] | ■ Experimental study ■ Phase change material. ■ Comparative Analysis |
Summation of the thermal and electrical efficiencies constitutes overall efficiency given by: ηpvt≅Eth+EelEin=>ηpvt=∫t2t1(AcE″th+ApvE″el)dtAc∫t2t1(G″eff)dt=ηth+r.ηel where, Ac—area of the collector, Apv—area of PV cells, r—packing factor defined as Apv/Ac. δR=√(δRδv1δv1)2+(δRδv2δv2)2+⋯+(δRδvnδvn)2 where δR—uncertainty associated with R, dvi —uncertainty associated with vi |
Thermal efficiency Electrical efficiency |
Shamani et al. [41] | ■ Experimental Study ■ Grid connected PVT systems |
The PV module efficiency is determined using: ηPV=(EDCHr∗Ac)∗100 (%) The system efficiency is given as: ηsys=(EACHr∗Ac)∗100 (%) The inverter efficiency is given as: ηinv=(EACEDC)∗100 (%) |
Overall efficiency Electrical efficiency |
Hasan et al. [42] | ■ Experimental study ■ Jet array nanofluids impingement on PVT collectors |
Overall thermal efficiency of the PVT system is given by: ηthι=FR(τα)−FRUL(Ti−TaGT) The electrical efficiency of the PV module can be determined using the following equation: ηele=ηΓ(1−γ(Tc−Tr)) |
Electrical, thermal and combined Photovoltaic efficiency. |
Gangadevi et al. [43] | ■ Experimental Work ■ Hybrid PV/Spiral flow thermal collector system |
ηpvt≅Eth+EelEin=>ηpvt=∫t2t1(AcE″th+ApvE″el)dtAc∫t2t1(G″eff)dt=ηth+r.ηel where Ac—area of the collector, Apv—area of PV cells and r—packing factor given as Apv/Ac |
Electrical, thermal and overall PVT efficiency. |
Chandrasekar et al. [44] | ■ Experimental Work | The efficiency of the PV module (η) is calculated by : η=PAI(t) Probable errors in the variables of the problem are calculated which is further used to estimate the uncertainties associated with experimental results as: ΔPP=[(ΔV)V)2+(ΔII)2]0.5 |
Electrical Efficiency |
An et al. [45] | ■ Experimental ■ Concentrating PV/T collector |
η=(ˉPpv+ˉPth)/ˉGCA where, η is overall efficiency, ˉPpv is average electrical power, ˉPth is average thermal power, ˉG is Average solar irradiance, C is concentration ratio and A is Area of Cell unit. δx=Max(xi−ˉx)ˉx where, δx is Relative error, xi is testing value and x is average value |
Overall efficiency |
Xu and Kleinstreuer [46] | ■ CPV/T system ■ Experimntal and numerical study |
The electrical efficiency of the CPV/T system is defined as the ηel=Gec.ηopt.ηcell.ηinv−WsysGrec where ηopf is the optical efficiency, ηce// is the cell efficiency, ηjnv. Is the electrical inverter efficiency, and Wsys is the electrical power required for running the CPV/T system |
Electrical efficiency Overall efficiency |
Hjerrild et al. [47] | ■ Experimental Study ■ Hybrid Ag-SiO2 /Carbon nanofluids |
The value of w(worthfactor) is derived from the relative value of electricity and thermal energy and the fact that thermal power plants typically convert three units of heat into one unit of electricity MF=w∗PPV+PThw∗PPV,nofilteer |
PV efficiency |
Taylor et al. [49] | ■ Experimental Study ■ Optical Fibres in PVT systems |
Nanofluid is used as a bandpass filter, overall efficiency is given by η=∫longλshortλEλTλdλ)∫longλshortλEλdλ)−∫shortλ0EλTλdλ)∫shortλ0Eλdλ)−∫4μmlongλEλTλdλ)∫4μmlongλEλdλ) where Eλ is the is the amount of solar irradiance per unit wavelength. A perfectly transparent (T=1) filter between the short and long edges which is also perfectly absorbing (T=0) outside of that range, will achieve an efficiency of 1. |
Overall efficiency |
Xu and Kleinstreuer [57] | ■ Experimental study ■ Numerical Study |
Sgen(themal)=kT2[(∂T∂X)2+(∂T∂y)2] Sgen (frictional) =Sgen(frictiona1)+Sgen(frictiona1)′=μT(∂ˉu∂y)2+μTΦ′ |
Overall system efficiency |
Authors | Type of Study and System | Mathematical Modelling of the system | Parameters Evaluated |
Jing et al. [32] | ● CPVT system. ● Numerical study |
PV/T system overall exergetic efficiency is defined as, Exoverall=Eth+EeleEsun=ηth(1−TenTout)+ExelEsun |
Exergetic efficiency |
Al-Waeli et al. [36] | ● Photovoltaic thermal PV/T collectors | The total efficiency (ηr)=the thermal efliciency + the electrical efficiency ηt=ηthermal+ηelectrical=Qu+PIs×At where At—the total area of the panel and collector. eR=[(∂R∂V1e1)2+(∂R∂V2e2)2+…+(∂R∂Vnen)2]0.5 where, eR: uncertainty in the results, R: a given function of the independent variables y1,y2,…,yΠ or R=R(V1,V2,…,Vn) and ei: uncertainty interval in the nth variable. |
Overall system efficiency |
Lari et al. [39] | ● Numerical simulations using ANSYS 15.0 ● Mesh generation using GAMBIT |
Computational Fluid Dynamics on PVT panels followed by extensive economic analysis was carried out in this study. | Thermal and Electrical efficiency Economic Analysis |
Khanjari et al. [56] | ● Numerical ● Sheet and tube PV/T system |
Computational fluid dynamics (CFD) employing conjugate heat transfer model is used for estimating the efficiencies. | Heat transfer coefficient |
Hassani et al. [50] | ● Physical modelling ● Electrical Modelling |
PVT system overall efficiency is estimated as: ηov=ηel+J(2∑i=1(1−T0Tn,i)ηth,i where i represents the channel number, (1−T0/Tn,i) is the Carnot efficiency, K - usefull fraction of thermal energy taken as 0.5, Thermal exergy efficiency—KΣ2i=1(1−T0Tn,i)ηth,i |
Electrical output Thermal Exergy output |
Saroha et al. [59] | ● Theoretical & Numerical Modelling ● Indoor hybrid PV/T system |
In this study, a nanofluid based PV/T hybrid collector is proposed and numerically modelled. | Thermal and Overall efficiency |
Table 3 summarizes the maximum percentage of enhancement of electrical, thermal and overall efficiency of the PV/T systems by using nanofluid as the coolant instead of base fluid, obtained from the below mentioned papers. The greatest enhancement in electrical efficiency of 24.1% was observed for SiC/water nanofluid with 3%wt. It is clear that SiC/water nanofluid is best among the various coolant used in the studies mentioned below in Table 3. With the decrease in concentration SiC nanoparticles plays the same role with lesser electrical efficiency attainment. SiC nanofluid with 1% wt. concentration shown 12.75% electrical efficiency wich further increases to 13.52% for 2% wt. SiC is also used with Parrafin wax in nano-enhanced phase change material which improves the electrical efficiency from 8.7% to 13.52% and the overall efficiency of the system was increased from 8.4% to 13.1%. The thermal efficiency enhanced by 1.96%, 3.42% and 4.8% by using nanofluid Al2O3, CuO and SiC respectively. So SiC has also shown better thermal performance as compared to Al2O3 and CuO. However the highest thermal efficiency of 45% is observed by Al2O3 for 1% wt. which is also cost promising among the various nanofluid type. Ag and Au also shown increment in electrical and thermal efficiency but these values are still lesser than other cheaper nanofluid. Ag/water with 0.5% vol. concentration produced 8.5% enhancement in electrical efficiency while 13% in thermal efficiency. In between Ag and Au with water as a base fluid Ag shown better performance both in electrical and thermal output. When nanofluid is used as optical filter than lesser concentration is sufficient to overcome the heating problem of PV panel and hence can improve performance with lesser cost input. In Xu and Kleinstreuer [46] SiO2 nanofluid is used as optical filter which improves the performance to a larger extent. At 62 ℃ the overall efficiency of the system reached 70% and electrical efficiency was found to be 11% whereas the thermal efficiency was observed to be 59%. After silica–water nanofluid Ag shown second largest enhancement in overall efficiency of about 30% then followed by Cu9S5 to 17%. A single study using hybrid nanofluid (Ag-SiO2)/water as an optical is also observed in the literature however adding silica to silver nanoparticles does not add to enhancement rather the value dips to 30% for overall efficiency in comparison to solo application of silica which gives highest overall efficiency when used as an optical filter.
S.No. | Authors | Year | Location | Nano particles | Base fluid | Concentration and size | Nanofluid usage | Results |
1. | Shamani et al. [26] | 2016 | Malaysia | SiO2, TiO2 and SiC | Water | 0.5, 1, 2 wt.% | Coolant | SiC nanofluid had the highest PV/T electrical efficiency of 13.52% followed by TiO2 and SiO2 nanofluids. |
2. | Yun and Qunzhi [27] | 2012 | China | MgO | Water | 0.02%wt. 0.06% wt. 0.10% wt. | Coolant | The designed PV/T system having a 2 mm thick liquid layer had efficiency above 60%. |
3. | Michael and Iniyan [28] | 2015 | India | CuO | Water | 0.05 vol%, 75 nm | Coolant | Improvement in the thermal efficiency upto 45.76% compared to water. |
4. | Rejeb et al. [29] | 2015 | TunisiaIran France | Al2O3 and Cu | Water & Ethylene glycol | 0.001–1.5%, 10 nm | Coolant | The best thermal and electrical efficiency was attained by using Cu/water in the place of Cu/ethylene glycol and same as for Al2O3/water in comparison with Al2O3/ethylene glycol. |
5. | Sardarabadi et al. [30] | 2014 | Iran | SiO2 | Water | 1, 3 wt %, 11–14 nm | Coolant | Increase of 7.6% in thermal efficiency for nanofluid with 1% wt and for 3% wt the thermal efficiency was increased by 12.8%. The overall efficiency was also upgraded with the use of nanofluid, for 1% wt the increase was 3.6% whereas it goes to 7.9% in case of 3% wt. |
6. | Al-Waeli et al. [31] | 2016 | Malaysia | SiC | Water | 3 wt.% | Coolant | The electrical efficiency was increased up-to 24.1%. The overall efficiency of the PV/T system was about 88.9%. |
7. | Jing et al. [32] | 2015 | China | SiO2 | Water | 0.5, 1, 2 vol%, 5, 10, 25, 50 nm | Coolant | Exergetic efficiency was enhanced by 9.5%. |
8. | Radwan et al. [33] | 2016 | Egypt | Al2O3 and SiC | Water | 0–4vol.%, 20 nm | Coolant | SiC was found to be more effective than aluminium oxide Al2O3 nanofluid. |
9. | Karami and Rahimi et al.[34] | 2013 | Iran | Boehmite (AlOOH.xH2O) | Water | 0.01, 0.1, 0.3 wt%, 5–10 nm | Coolant | The electrical efficiencies for straight and helical systems were 20.57% and 37.67% respectively. |
10. | Hussien et al. [35] | 2015 | Iraq | Al2O3 | Water | 0.1–0.5% 30 nm | Coolant | The electrical efficiency increased to 12.1% and thermal efficiency increased to 34.4%. |
11. | Al-Waeli et al. [36] | 2017 | Malaysia | Al2O3, CuO and SiC | Water | 0.5, 1, 2, 3, and 4% by vol Al2O3:30–60 nm CuO: 35–45 nm SiC: 45–65 nm | Coolant | The thermal efficiency enhanced by 1.96%, 3.42% and 4.8% by using nanofluid Al2O3, CuO and SiC respectively. |
12. | Sardarabadi et al. [37] | 2016 | Iran | Al2O3, TiO2 and ZnO | Water | Al2O3: 2 wt.%, 20nm TiO2: 2 wt.%, 10-30 nm ZnO: 2wt.%, 10–25 nm | Coolant | The electrical efficiency for deionized water, TiO2, ZnO and Al2O3 was enhanced by 5.48%, 6.54%, 6.46% and 6.36% respectively. |
13. | Bellos et al. [38] | 2017 | Greece | Cu | Water | 0%–4% | Coolant | Electrical efficiency enhanced by 1.49% and the thermal efficiency was increased by 4.35%. |
14. | Lari et al. [39] | 2017 | Saudi Arabia | Ag | Water | 0.5 vol%, 10 nm | Coolant | There was an increase in electrical output by 8.5% and an increase in thermal output by 13%. |
15. | Sardarbadi et al. [40] | 2017 | Iran | ZnO | Water | 0.2% wt. 50 nm | Coolant | The results showed an increase of 13% in the electrical efficiency and 5% in thermal efficiency as compared to the conventional PV module. |
16. | Shamani et al. [41] | 2016 | Malaysia | SiC | Water | Phase change material | The electrical efficiency of the system was enhanced from 8.77% to 13.52% and the overall efficiency of the system was increased from 8.4% to 13.1%. | |
17. | Hasan et al. [42] | 2016 | Malaysia | SiC, TiO2 and SiO2 | Water | 1 wt.% | Coolant | The electrical, thermal and overall efficiency of the system in case of SiC nanoparticle was documented 12.75%, 85% and 97.75% respectively. |
18. | Gangadevi et al. [43] | 2017 | India | Al2O3 | Water | 1% wt and 2% wt. 50 nm | Coolant | Electrical, thermal and overall efficiency enhanced by 13%, 45% and 58% respectively. |
19. | Chandrasekar et al. [44] | 2012 | India | Al2O3 and CuO | Water | 0.1 vol.% | Coolant | The maximum electrical efficiency when no cooling was provided was 9% and which was increased to 9.4% and 9.8% when cooling fluid CuO nanofluid and Al2O3 nanofluid respectively was used. |
20. | An et al. [45] | 2016 | China | Cu9S5 | Water | 22.3, 44.6, 89.2 ppm, 60.2 nm | Spectral filter | The overall efficiency of the PV/T system increased upto 17%. |
21. | Xu and Kleinstreuer [46] | 2014 | U.S | SiO2 | Water | Optical filter | At 62 ℃ the overall efficiency of the system reached 70% and electrical efficiency was found to be 11% whereas the thermal efficiency was 59%. | |
22. | Hjerrild et al. [47] | 2016 | Australia | Ag-SiO2 | Water | Ag-SiO2: 0.001, 0.003, 0.006, 0.026 wt.% 17.5 nm | Spectral filter | The increase in overall efficiency was about 30% on using the Ag-SiO2. |
23. | Taylor et al. [49] | 2012 | Australia | SiO2 | Water and Therminol VP-1 | Therminol VP-1: 0–0.01 wt.% 20–50 nm | Spectral filter | An enhancement of 4%–8% in the efficiency of the PV/T system as compared to the water based PV/T system. |
24. | Khanjari et al. [56] | 2016 | Iraq | Al2O3 & Ag | Water | 0–12%, 50 nm | Coolant | For Alumina-water heat transfer coefficient increased by 12%. For Ag-water heat transfer coefficient increased by 43%. |
25. | Xu and Kleinstreuer [57] | 2014 | U.S | Al2O3 | Water | 5 vol.%, 38.4 nm | Coolant | The system efficiency achieved was 20%. |
26. | Hassani et al. [50] | 2016 | Malaysia | Ag | Water and Therminol VP-1 | 0.001–1.5 vol.%, 10 nm | Spectral filter & coolant | Electrical efficiency was increased by 9% and 5.6% respectively and the overall efficiency of the system was improved to 30% and 24.4% respectively for GaAs and Si cells. |
27. | Saroha et al. [59] | 2015 | India | Au and Ag | Water | Au: 0.00019, 0.0093 vol.% 5, 10 nm Ag: 0.00025, 0.00193 vol.%, 5, 10 nm | Spectral filter and coolant | Highest thermal and overall efficiency is achived using Ag-water nanofluid. |
Hassani et al. [50] carried out the study for Ag/water-therminol as an optical as well as coolant. It was observed Electrical efficiency was increased by 9% and 5.6% respectively and the overall efficiency of the system was improved to 30% and 24.4% respectively for Ga-As and Si cells. Figure 8 & 9 depicts the Performance comparison of various parameters of hybrid PVT systems using different nanofluids (Figure 8 showing efficiency achieved by the system and Figure 9 showing increment in efficiency). Here y-axis represents the reference number from the bibliography while x-axis represents the efficiency and the colour trend tells about the thermal, electrical and overall efficiency. It is clear from figure 8, that the highest overall efficiency of around 96% is observed in the study of [42] which incorporated the SiC/water nanofluid in PVT system. While the maximum electrical efficiency is achieved by Boehmite based PV/T system, which reported 39% electrical efficiency [34]. However the highest thermal efficiency is achieved in the study of [42] of about 82%. On the other side the lowest value of electrical, thermal and overall efficiency is achieved by [41] of about 10%, [35] of about 37% and [45] of about 18% respectively. Maximum and minimum percentage enhancement in the value of overall efficiency is observed in the study of [43] and [30] respectively. Similarly maximum percentage enhancement in the electrical efficiency is observed in [40] of about 16%. There exist studies in the literature which compare the role of nanofluid as a coolant and also as an optical filter to filter out unwanted part of solar spectrum. Figure 10 & 11 both demonstrate the capabilities of nanofluid as a coolant and optical filter respectively. Reference [42] of the bibliography gives best performance of nanofluid as a coolant in terms of overall efficiency. The overall efficiency achieved is 97% which is too high. However least overall efficiency of about 16% is observed in the study of [41]. Similarly best optical filter as shown in Figure 11, corresponds to reference [46] which improves the overall efficiency to maximum value of 70% and thermal efficiency to about 60%. Reference [45] corresponds to study in which least overall efficiency is observed when nanofluid is used as an optical filter.
In PVT system due to high temperature rise, inevitable heating is faced by PV panels which can be further processed to useful thermal energy. A variety of approaches have appeared as promising avenues to alleviate this problem and deliver high electrical efficiency and a better thermal delivery. A comprehensive summary of nanofluid performance enhancement of Hybrid PV/T system is carried out to ascertain the role of nanofluid as a coolant and as an optical filter their benefits and improvement associated. Furthermore PCM capabilities were discussed and ways of efficiency improvements were focussed in this critical review to lower the PV panel temperature. Spectral filtering provision is also reviewed for the past decade in context with nanofluid and nanofilm. This has included an up-to-date review of recent publications covering the development of relevant approaches and of suitable existing and emerging nanomaterials as applied to spectral splitting, along with a discussion of the arising challenges and opportunities in different approaches and applications.
Looking over the different papers in wide perspective we can say that nanofluid based hybrid PV/T systems can meet the world's energy demands. All the nanofluids are compared on different parameters to make a biased comparison. According to the results of different authors worked in this field, following conclusions were drawn:
● Effectiveness of nanofluid as a coolant is more remarkable for low flow rate as it get enough interaction for heat transfer from wall to ambient.
● Effect of nanoparticle size over the efficiency of PVT system is negligible.
● The effect of the base fluid is found to be very well pronounced in the PVT systems.
● Performance of water is found to be better than EG-water and therminol-water as a base fluid.
● Nanomaterials have emerged as a particularly promising solution for achieving specific, desired spectral-splitting characteristics and performance.
● Current nanofilm filters still suffer from considerable optical losses.
● The nanoparticle based fluid filters system has 4% higher thermal efficiency than conventional thin film-based optical fluid filters.
After conducting an exhaustive study of so many papers written in the last 20 years on PV/T systems employing nanofluid cooling (active/passive) techniques, followings points can be anticipated for further work in this field:
1) Today there are various methods by which nanofluid colloids can be prepared and further work can be carried out in this way to explore new methods by which nanofluids can be prepared to make them more and more stable.
2) Various heat transfer methods and different parameters can be overlooked employing various CFD simulations in Hybrid PV/T systems.
3) Experimental and Numerical investigation can be carried out on deployment of various base fluids except water like ethylene glycol etc. both ways as coolant and optical filter.
4) Various methods of nanofluid manufacturing are yet to be overlooked in which the increase of thermal conductivity is more than the increase of its viscosity.
5) Performance parameters of Hybrid PV/T system can be found by using different combinations of nanofluids. Different nanofluids with different thermal conductivity.
6) Some newly developed particles like MXene and grapheme can be employed with different base fluid to get better performance.
7) Optimisation techniques can be employed to get optimal parametrs level for hybrid PVT system. Machine learning approach can be employed for prediction of efficiency of PVT system.
8) Nano-enhanced Phase Change Materials or other emerging techniques such as thermoelectric devices can be employed for the cooling purpose of PV cells. This field is almost vacant and needs to work extensively to explore more of its capabilities.
The authors declare that there is no conflict of interest in publishing this paper.
[1] |
Kazem HA, Al-Waeli AH, Chaichan MT, et al. (2017) Design, measurement and evaluation of photovoltaic pumping system for rural areas in Oman. Environ, Dev Sustainability 19: 1041-1053. doi: 10.1007/s10668-016-9773-z
![]() |
[2] |
Zhang S, Zhuang Z, Hu Y, et al. (2016) Applicability study on a hybrid renewable energy system for Net-Zero energy house in Shanghai. Energy Procedia 88: 768-774. doi: 10.1016/j.egypro.2016.06.108
![]() |
[3] | Tan H (2015) The development and applicability of renewable energy for buildings. Build Sci 8: 34-42. |
[4] | Firth SK (2006) Raising efficiency in photovoltaic systems: High resolution monitoring and performance analysis. |
[5] | Kazem HA, Al-Badi HA, Al Busaidi AS, et al. (2016) Optimum design and evaluation of hybrid solar/wind/diesel power system for Masirah Island. Environ, Dev Sustainability 19: 1761-1778. |
[6] | Oi A (2005) Design and simulation of photovoltaic water pumping system. California Polytechnic State University. |
[7] |
Chow TT (2010) A review on photovoltaic/thermal hybrid solar technology. Appl Energy 87: 365-379. doi: 10.1016/j.apenergy.2009.06.037
![]() |
[8] |
Kalogirou SA, Tripanagnostopoulos Y (2006) Hybrid PV/T solar systems for domestic hot water and electricity production. Energy Convers Manage 47: 3368-3382. doi: 10.1016/j.enconman.2006.01.012
![]() |
[9] |
Li M, Ji X, Li G, et al. (2011) Performance study of solar cell arrays based on a trough concentrating photovoltaic/thermal system. Appl Energy 88: 3218-3227. doi: 10.1016/j.apenergy.2011.03.030
![]() |
[10] |
Shan F, Tang F, Cao L, et al. (2014) Performance evaluations and applications of photovoltaic-thermal collectors and systems. Renewable Sustainable Energy Rev 33:467-483. doi: 10.1016/j.rser.2014.02.018
![]() |
[11] |
Tyagi VV, Kaushik SC, Tyagi SK (2012) Advancement in solar photovoltaic/thermal (PV/T) hybrid collector technology. Renewable Sustainable Energy Rev 16: 1383-1398. doi: 10.1016/j.rser.2011.12.013
![]() |
[12] |
Tripanagnostopoulos Y (2007) Aspects and improvements of hybrid photovoltaic/thermal solar energy systems. Sol Energy 81: 1117-1131. doi: 10.1016/j.solener.2007.04.002
![]() |
[13] |
Tiwari GN, Mishra RK, Solanki SC (2011) Photovoltaic modules and their applications: A review on thermal modelling. Appl Energy 88: 2287-2304. doi: 10.1016/j.apenergy.2011.01.005
![]() |
[14] |
Reddy SR, Ebadian MA, Lin CX (2015) A review of PV-T systems: thermal management and efficiency with single phase cooling. Int J Heat Mass Trans 91: 861-871. doi: 10.1016/j.ijheatmasstransfer.2015.07.134
![]() |
[15] |
Charalambous PG, Maidment GG, Kalogirou SA, et al. (2007) Photovoltaic thermal (PV/T) collectors: A review. Appl Therm Eng 27: 275-286. doi: 10.1016/j.applthermaleng.2006.06.007
![]() |
[16] |
Besheer AH, Smyth M, Zacharopoulos A, et al. (2016) Review on recent approaches for hybrid PV/T solar technology. Int J Energy Res 40: 2038-2053. doi: 10.1002/er.3567
![]() |
[17] |
Kim HJ, Lee SH, Lee JH, et al. (2015) Effect of particle shape on suspension stability and thermal conductivities of water-based bohemite alumina nanofluids. Energy 90: 1290-1297. doi: 10.1016/j.energy.2015.06.084
![]() |
[18] |
Ali HM, Ali H, Liaquat H, et al. (2015) Experimental investigation of convective heat transfer augmentation for car radiator using ZnO-water nanofluids. Energy 84: 317-324. doi: 10.1016/j.energy.2015.02.103
![]() |
[19] |
Manikandan S, Rajan KS (2015) MgO-Therminol 55 nanofluids for efficient energy management: analysis of transient heat transfer performance. Energy 88: 408-416. doi: 10.1016/j.energy.2015.05.061
![]() |
[20] |
Sun B, Lei W, Yang D (2015) Flow and convective heat transfer characteristics of Fe2O3-water nanofluids inside copper tubes. Int Commun Heat Mass Tran 64: 21-28. doi: 10.1016/j.icheatmasstransfer.2015.01.008
![]() |
[21] |
Setti D, Sinha MK, Ghosh S, et al. (2015) Performance evaluation of Ti-6Al-4V grinding using chip formation and coefficient of friction under the influence of nanofluids. Int J Mach Tools and Manuf 88: 237-248. doi: 10.1016/j.ijmachtools.2014.10.005
![]() |
[22] |
Zhang Y, Li C, Jia D, et al. (2015) Experimental evaluation of the lubrication performance of MoS2/CNT nanofluid for minimal quantity lubrication in Ni-based alloy grinding. Int J Mach Tools Manuf 99: 19-33. doi: 10.1016/j.ijmachtools.2015.09.003
![]() |
[23] |
Hong J, Kim D (2012) Effects of aggregation on the thermal conductivity of alumina/water nanofluids. Thermochim Acta 542: 28-32. doi: 10.1016/j.tca.2011.12.019
![]() |
[24] |
Meng ZQ, Li XB, Xion YJ, et al. (2012) Preparation and tribological performances of Ni-P-multi-walled carbon nanotubes composite coatings. Trans Nonferrous Met Soc China 22: 2719-2725. doi: 10.1016/S1003-6326(11)61523-9
![]() |
[25] |
Abdolzadeh M, Ameri M (2009) Improving the effectiveness of a photovoltaic water pumping system by spraying water over the front of photovoltaic cells. Renewable Energy 34: 91-96. doi: 10.1016/j.renene.2008.03.024
![]() |
[26] |
Al-Shamani AN, Sopian K, Mat S, et al. (2016) Experimental studies of rectangular tube absorber photovoltaic thermal collector with various types of nanofluids under the tropical climate conditions. Energy Convers Manage 124: 528-542. doi: 10.1016/j.enconman.2016.07.052
![]() |
[27] | Cui Y, Zhu Q (2012) Study of photovoltaic/thermal systems with MgO-water nanofluids flowing over silicon solar cells. Asia-Pacific Power and Energy Engineering Conference (APPEEC), 1-4. |
[28] |
Michael JJ, Iniyan S (2015) Performance analysis of a copper sheet laminated photovoltaic thermal collector using copper oxide-water nanofluid. Sol Energy 119: 439-451. doi: 10.1016/j.solener.2015.06.028
![]() |
[29] | Rejeb O, Sardarabadi M, Ménézo C, et al. (2016) Numerical and model validation of uncovered nanofluid sheet and tube type photovoltaic thermal solar system. Energy Convers Manage 110: 367-377. |
[30] |
Sardarabadi M, Passandideh-Fard M, Heris SZ, (2014) Experimental investigation of the effects of silica/water nanofluid on PV/T (photovoltaic thermal units). Energy 66: 264-272. doi: 10.1016/j.energy.2014.01.102
![]() |
[31] | Al-Waeli AH, Sopian K, Chaichan M, et al. (2017) An experimental investigation of SiC nanofluid as a base-fluid for a photovoltaic thermal PV/T system. Energy Convers Manage 142: 547-558. |
[32] |
Jing D, Hu Y, Liu M, et al. (2015) Preparation of highly dispersed nanofluid and CFD study of its utilization in a concentrating PV/T system. Sol Energy 112: 30-40. doi: 10.1016/j.solener.2014.11.008
![]() |
[33] | Radwan A, Ahmed M, Ookawara S (2016) Performance enhancement of concentrated photovoltaic systems using a microchannel heat sink with nanofluids. Energy Convers Manage 119: 289-303. |
[34] |
Karami N, Rahimi M (2014) Heat transfer enhancement in a PV cell using Boehmite nanofluid. Energy Convers Manage 86: 275-285. doi: 10.1016/j.enconman.2014.05.037
![]() |
[35] | Hussien HA, Noman AH, Abdulmunem AR (2015) Indoor investigation for improving the hybrid photovoltaic/thermal system performance using nanofluid (Al2O3-water). Eng Tech J 33: 889-901. |
[36] | Al-Waeli AH, Chaichan MT, Kazem HA, et al. (2017) Comparative study to use nano-(Al2O3, CuO, and SiC) with water to enhance photovoltaic thermal PV/T collectors. Energy Convers Manage 148: 963-973. |
[37] |
Sardarabadi M, Passandideh-Fard M (2016) Experimental and numerical study of metal-oxides/water nanofluids as coolant in photovoltaic thermal systems (PV/T). Sol Energy Mater Sol Cells 157: 533-542. doi: 10.1016/j.solmat.2016.07.008
![]() |
[38] |
Bellos E, Tzivanidis C (2017) Yearly performance of a hybrid PV operating with nanofluid. Renewable Energy 113: 867-884. doi: 10.1016/j.renene.2017.06.060
![]() |
[39] |
Lari MO, Sahin AZ (2017) Design, performance and economic analysis of a nanofluid-based photovoltaic/thermal system for residential applications. Energy Convers Manage 149: 467-484 doi: 10.1016/j.enconman.2017.07.045
![]() |
[40] |
Sardarabadi M, Passandideh-Fard M, Maghrebi MJ, et al. (2017) Experimental study of using both ZnO/water nanofluid and phase change material (PCM) in photovoltaic thermal systems. Sol Energy Mater Sol Cells 161: 62-69. doi: 10.1016/j.solmat.2016.11.032
![]() |
[41] |
Al-Shamani AN, Sopian K, Mat S, et al. (2017) Performance enhancement of photovoltaic grid-connected system using PV/T panels with nanofluid. Sol Energy 150: 38-48. doi: 10.1016/j.solener.2017.04.005
![]() |
[42] |
Hasan HA, Sopian K, Jaaz AH, et al. (2017) Experimental investigation of jet array nanofluids impingement in photovoltaic/thermal collector. Sol Energy 144: 321-334. doi: 10.1016/j.solener.2017.01.036
![]() |
[43] | Gangadevi R, Vinayagam BK, Senthilraja S (2017) Experimental investigations of hybrid PV/Spiral flow thermal collector system performance using Al2O3/water nanofluid. IOP Conference Series: Materials and Science Engineering.197: 012041. |
[44] |
Chandrasekar M, Suresh S, Senthilkumar T (2013) Passive cooling of standalone flat PV module with cotton wick structures. Energy Convers Manage 71: 43-50. doi: 10.1016/j.enconman.2013.03.012
![]() |
[45] |
An W, Wu J, Zhu T, et al. (2016) Experimental investigation of a concentrating PV/T collector with Cu9S5 nanofluid spectral splitting filter. Appl Energy 184: 197-206. doi: 10.1016/j.apenergy.2016.10.004
![]() |
[46] |
Xu Z, Kleinstreuer C (2014) Concentration photovoltaic-thermal energy co-generation system using nanofluids for cooling and heating. Energy Convers Manage 87: 504-512. doi: 10.1016/j.enconman.2014.07.047
![]() |
[47] |
Hjerrild NE, Mesgari S, Crisostomo F, et al. (2016) Hybrid PV/T enhancement using selectively absorbing Ag-SiO2/carbon nanofluids. Sol Energy Mater Sol Cells 147: 281-287. doi: 10.1016/j.solmat.2015.12.010
![]() |
[48] |
Otanicar TP, Chowdhury I, Prasher R, et al. (2011) Band-gap tuned direct absorption for a hybrid concentrating solar photovoltaic/thermal system. J Sol Energy Eng 133: 041014. doi: 10.1115/1.4004708
![]() |
[49] | Taylor RA, Otanicar T, Rosengarten G (2012) Nanofluid-based optical filter optimization for PV/T systems. Light: Sci Appl 1: 1-7. |
[50] |
Hassani S, Saidur R, Mekhilef S, et al. (2016) Environmental and exergy benefit of nanofluid based hybrid PV/T systems. Energy Convers Manage 123: 431-444. doi: 10.1016/j.enconman.2016.06.061
![]() |
[51] |
Otanicar TP, Taylor RA, Telang C (2013) Photovoltaic/thermal system performance utilizing thin film and nanoparticle dispersion based optical filters. J Renewable Sustainable Energy 5: 033124. doi: 10.1063/1.4811095
![]() |
[52] |
Dorodnyy A, Shklover A, Braginsky L, et al. (2015) High-efficiency spectrum splitting for solar photovoltaics. Sol Energy Mater Sol Cells 136: 120-126. doi: 10.1016/j.solmat.2015.01.005
![]() |
[53] | Eisler CA, Flowers CA, Warmann EC, et al. (2018) The polyhedral specular reflector: A spectrum-splitting multijunction design to achieve ultrahigh (>50%) solar module efficiencies. IEEE J Photovolt 9: 174-182. |
[54] |
Liang H, Cheng Z, Wang H, et al. (2019) Investigation on optical properties and solar energy conversion efficiency of spectral splitting PV/T system. Energy Procedia 158: 15-20. doi: 10.1016/j.egypro.2019.01.025
![]() |
[55] |
Hjerrild NE, Mesgari S, Crisostomo F, et al. (2016) Hybrid PV/T enhancement using selectively absorbing Ag-SiO2/carbon nanofluids. Sol Energy Mater Sol Cell 147: 281-287. doi: 10.1016/j.solmat.2015.12.010
![]() |
[56] |
Khanjari Y, Pourfayaz F, Kasaeian AB (2016) Numerical investigation on using of nanofluid in a water-cooled photovoltaic thermal system. Energy Convers Manage 122: 263-278. doi: 10.1016/j.enconman.2016.05.083
![]() |
[57] |
Xu Z, Kleinstreuer C (2014) Computational analysis of nanofluid cooling of high concentration photovoltaic cells. J Therm Sci Eng Appl 6: 031009. doi: 10.1115/1.4026355
![]() |
[58] | Al-Waeli AH, Kazem HA, Sopian K, et al. (2017) Techno-economical assessment of grid connected PV/T using nanoparticles and water as base-fluid systems in Malaysia. Inter J Sustainable Energy 37: 558-575. |
[59] | Saroha S, Mittal T, Modi PJ, et al. (2015) Theoretical analysis and testing of nanofluids-based solar photovoltaic/thermal hybrid collector. J Heat Trans 137: 091015. |
[60] |
Zhao J, Song Y, Lam WH, et al. (2011) Solar radiation transfer and performance analysis of an optimum photovoltaic/thermal system. Energy Convers Manage 52: 1343-1353. doi: 10.1016/j.enconman.2010.09.032
![]() |
[61] | No€el JA, et al. (2016) Phase change materials. Storing Energy, Elsevier, Oxford, 249-272. |
[62] | Parameshwaran R, Sarı A, Jalaiah N, et al. (2018) Applications of thermal analysis to the study of phase-change materials. Handb Therm Anal Calorim 6: 519-572. |
[63] |
Zondag HA, de Boer R, Smeding SF, et al. (2018) Performance analysis of industrial PCM heat storage lab prototype. J Energy Storage 18: 402-413. doi: 10.1016/j.est.2018.05.007
![]() |
[64] |
Wang G, Xu C, Wei G, et al. (2019) Numerical study of a novel dual-PCM thermal energy storage structure filled with inorganic salts and metal alloy as the PCMs. Energy Procedia 158: 4423-4428. doi: 10.1016/j.egypro.2019.01.774
![]() |
[65] |
Raul A, Jain M, Gaikwad S, et al. (2018) Modelling and experimental study of latent heat thermal energy storage with encapsulated PCMs for solar thermal applications. Appl Therm Eng 143: 415-428. doi: 10.1016/j.applthermaleng.2018.07.123
![]() |
[66] |
Mousavi S, Kasaeian A, Shafii MB, et al. (2018) Numerical investigation of the effects of a copper foam filled with phase change materials in a water-cooled photovoltaic/thermal system. Energy Convers Manage 163: 187-195. doi: 10.1016/j.enconman.2018.02.039
![]() |
[67] |
Su D, Jia Y, Lin Y, et al. (2017) Maximizing the energy output of a photovoltaic thermal solar collector incorporating phase change materials. Energy Build 153: 382-391. doi: 10.1016/j.enbuild.2017.08.027
![]() |
[68] |
Zhou Y, Liu X, Zhang G (2017) Performance of buildings integrated with a photovoltaic thermal collector and phase change materials. Procedia Eng 205: 1337-1343. doi: 10.1016/j.proeng.2017.10.109
![]() |
[69] |
Yang X, Sun L, Yuan Y, et al. (2018) Experimental investigation on performance comparison of PV/T-PCM system and PV/T system. Renewable Energy 119: 152-159. doi: 10.1016/j.renene.2017.11.094
![]() |
1. | Uvais Mustafa, Ibraheem Ahmed Qeays, M Saad BinArif, Syed Mohd Yahya, Shahrin Bin Md.Ayob, Efficiency improvement of the solar PV-system using nanofluid and developed inverter topology, 2020, 1556-7036, 1, 10.1080/15567036.2020.1808119 | |
2. | Huaxu Liang, Fuqiang Wang, Luwei Yang, Ziming Cheng, Yong Shuai, Heping Tan, Progress in full spectrum solar energy utilization by spectral beam splitting hybrid PV/T system, 2021, 141, 13640321, 110785, 10.1016/j.rser.2021.110785 | |
3. | Naman Parashar, Navid Aslfattahi, Syed Mohd Yahya, R. Saidur, ANN Modeling of Thermal Conductivity and Viscosity of MXene-Based Aqueous IoNanofluid, 2021, 42, 0195-928X, 10.1007/s10765-020-02779-5 | |
4. | Junaid Khan, Rashid Ali, Muzammil Zubair, Syed Mohd Yahya, Energy and Life Cycle Assessment of Zinc/Water Nanofluid Based Photovoltaic Thermal System, 2022, 119, 0199-8595, 827, 10.32604/ee.2022.016344 | |
5. | Farzad Hossain, Md. Rezwanul Karim, Arafat A. Bhuiyan, A review on recent advancements of the usage of nano fluid in hybrid photovoltaic/thermal (PV/T) solar systems, 2022, 188, 09601481, 114, 10.1016/j.renene.2022.01.116 | |
6. | Mohammad Hemmat Esfe, Mehdi Hajian, Davood Toghraie, Mohamad Khaje khabaz, Alireza Rahmanian, Mostafa Pirmoradian, Hossein Rostamian, Prediction the dynamic viscosity of MWCNT-Al2O3 (30:70)/ Oil 5W50 hybrid nano-lubricant using Principal Component Analysis (PCA) with Artificial Neural Network (ANN), 2022, 23, 11108665, 427, 10.1016/j.eij.2022.03.004 | |
7. | Warga Chegeno Meraje, Chang‐chiun Huang, Masaki Ujihara, Chung‐Feng Jeffrey Kuo, Performance enhancement study of ag@ SiO 2 core‐shell nanoplate plasmonic hybrid spectral splitting nanofluid based photovoltaic thermal system in a temperate region , 2022, 46, 0363-907X, 15008, 10.1002/er.8202 | |
8. | Shahriar Ahmed, KH. Nazmul Ahshan, Md. Nur Alam Mondal, Shorab Hossain, Application of metal oxides-based nanofluids in PV/T systems: a review, 2022, 16, 2095-1701, 397, 10.1007/s11708-021-0758-8 | |
9. | J. Prakash Arul Jose, Anurag Shrivastava, Prem Kumar Soni, N. Hemalatha, Saad Alshahrani, C. Ahamed Saleel, Abhishek Sharma, Saboor Shaik, Ibrahim M. Alarifi, Mohammad Rahimi-Gorji, An Analysis of the Effects of Nanofluid-Based Serpentine Tube Cooling Enhancement in Solar Photovoltaic Cells for Green Cities, 2023, 2023, 1687-4129, 1, 10.1155/2023/3456536 | |
10. | Samer Ali, Charbel Habchi, Hassan Zaytoun, Mahmoud Khaled, Talib Dbouk, Surrogate-based optimization of the attack and inclination angles of a delta winglet pair vortex generator in turbulent channel flow, 2023, 20, 26662027, 100473, 10.1016/j.ijft.2023.100473 | |
11. | M. Saad Bin Arif, Mohammad Sarfraz, Faroghul Imam, Brahim Mohamed Mrabet, Muhammad Mazin Al Maathidi, 2024, Chapter 45, 978-981-97-6348-1, 643, 10.1007/978-981-97-6349-8_45 |
Authors | Type of Study and System | Mathematical Modelling of the system | Parameters Evaluated |
Al-Shamani et al. [26] | ■ Experimental. ■ PV/T system having stainless steel tube absorber |
ηel=ηr(1−β(Tpm−Tr)) where, ηel is the electrical efficiency, ηr is the reference efficiency of the PV module, β is the temperature coefficient, Tpm is the temperature of the solar cells and Tr is the reference temperature. WR={(∂R∂x1w1)2+(∂R∂x2w2)2+…+(∂R∂xnwn)2}1/2 where, R is uncertainty - function of the independent variables x1, x2, xn and w1, w2, wn represents the uncertainties associated with independent variables. |
Electrical efficiency |
Yun and Qunzhi [27] | ■ Experimental ■ PV/T system |
ηpvt=ηth+ηe=Qu+PIs×At,Qu=˙mCP(To−Ti) ˙m is the mass flow rate, Is is the solar intensity, Ac is the collector area, Cp is the specific heat, To and Ti are outlet and inlet fluid temperatures respectively |
Overall efficiency |
Michael and Iniyan [28] | ■ Experimental and Numerical ■ Single rectangular absorber PV/T |
ηth=QuIs×At where, ηth is the thermal efficiency [(Uηlηl)2]thεrm=(U˙m˙m)2+(UGG)2+(UTT)2 where, U—denotes uncertainty, ˙m—mass flow rate of water, T—temperature difference between the outlet and inlet fluid and G—incident global solar radiation |
Thermal Efficiency |
Rejeb et al. [29] | ■ Experimental and Numerical ■ Sheet and tube PV/T |
Heat transfer modeling of collector was developed in FORTRAN language, based on the finite volume method. ηth=QuIs×At and ηel=ηo(1−β(Tpv−298)) [(Uηlηl)2]thεrm=(U˙m˙m)2+(UGG)2+(UTT)2 [(Uηiηl)2]elec=(UvV)2+(Ull)2+(UGG)2 where, V is voltage and I is current |
Thermal Efficiency Electrical Efficiency |
Sardarabadi et al. [30] | ■ Experimental study ■ Phase change material ■ Comparative Analysis |
ηpvt, is the overall efficiency which is the sum of the thermal and electrical efficiencies (ηth and ηel, respectively): ηpvt≅Eth+EelEin=>ηpvt=∫t2t1(AcE″th+ApvE″el)dtAc∫t2t1(Geff)dt=ηth+r.ηel where Ac—area of the collector, Apv—area of PV cells and r—packing factor. δR=√(δRδv1δv1)2+(δRδv2δv2)2+⋯+(δRδvnδvn)2 where δR is the uncertainty of function R. |
Thermal efficiency |
Al-Waeli et al. [31] | ■ Experimental study | The total efficiency (ηr)=the thermal efliciency+the electrical efficiency ηt=ηthermal+ηelectrical=Qu+PIs×At where, At the total area of the panel and collector. Change in the operations by: eR=[(∂R∂V1e1)2+(∂R∂V2e2)2+…+(∂R∂Vnen)2]0.5 where, eR: uncertainty in the results, R: a given function of the independent variables y1,y2,…,yΠ or R=R(V1,V2,…,Vn), ei: uncertainty interval in the nth variable. |
Electrical Thermal efficiency |
Radwan et al. [33] | ■ Experimental study | The solar cell generated electrical energy per unit area, thermal efficiency, friction factor and net produced power are calculated as follows: Psc=ηscβscτgG(t).CR, ηth=PthG.CR.Asc and Pfriction=ΔP.mρnf |
Thermal efficiency |
Karami and Rahimi et al. [34] | ■ Experimental study | The values of friction factor ω were calculated using the following equation: f=ΔpchDh2ρf.u2m.Lch where, Dch—hydraulic diameter. |
Electrical efficiency. |
Hussien et al. [35] | ■ Hybrid PVT systems. ■ Experimental study |
Hybrid PVT system overall efficiency is give as: ηtotal=ηth+ηele=mCp∫(To−Ti)dt+∫VIdtAo∫(t)dt |
Overall efficiency. |
Al-Waeli et al. [36] | ■ Photovoltaic thermal PV/T collectors | The total efficiency (ηr)=the thermal efliciency+the electrical efficiency ηt=ηthermal+ηelectrical=Qu+PIs×At where At—the total area of the panel and collector. eR=[(∂R∂V1e1)2+(∂R∂V2e2)2+…+(∂R∂Vnen)2]0.5 where, eR: uncertainty in the results, R: a given function of the independent variables y1,y2,…,yΠ or R=R(V1,V2,…,Vn) and ei: uncertainty interval in the nth variable. |
Overall system efficiency |
Sardarabadi et al. [37] | ■ Experimental and Numerical study | Electrical efficiency of PVT system can be estimated as ηclcc=Ee1ecEin=Voc×Jsc×FFGeffective where V—open circuit voltage and Isc—short circuit current and FF—Fill factor eR=[(∂R∂V1e1)2+(∂R∂V2e2)2+…+(∂R∂Vnen)2]0.5 where, eR: uncertainty in the results, R: a given function of the independent variables y1,y2,…,yΠ or R=R(V1,V2,…,Vn), ei: uncertainty interval in the nth variable. |
Electrical efficiency |
Bellos et al. [38] | ■ Experimental study ■ Performance Analysis |
The total exergy based on daily solar irradiation (Zs) is evaluated using following model: Zs=Ac.HT.(1−43.(TamTsun)+13.(TamTsun)4) |
Thermal efficiency Electrical efficiency |
Sardarbadi et al. [40] | ■ Experimental study ■ Phase change material. ■ Comparative Analysis |
Summation of the thermal and electrical efficiencies constitutes overall efficiency given by: ηpvt≅Eth+EelEin=>ηpvt=∫t2t1(AcE″th+ApvE″el)dtAc∫t2t1(G″eff)dt=ηth+r.ηel where, Ac—area of the collector, Apv—area of PV cells, r—packing factor defined as Apv/Ac. δR=√(δRδv1δv1)2+(δRδv2δv2)2+⋯+(δRδvnδvn)2 where δR—uncertainty associated with R, dvi —uncertainty associated with vi |
Thermal efficiency Electrical efficiency |
Shamani et al. [41] | ■ Experimental Study ■ Grid connected PVT systems |
The PV module efficiency is determined using: ηPV=(EDCHr∗Ac)∗100 (%) The system efficiency is given as: ηsys=(EACHr∗Ac)∗100 (%) The inverter efficiency is given as: ηinv=(EACEDC)∗100 (%) |
Overall efficiency Electrical efficiency |
Hasan et al. [42] | ■ Experimental study ■ Jet array nanofluids impingement on PVT collectors |
Overall thermal efficiency of the PVT system is given by: ηthι=FR(τα)−FRUL(Ti−TaGT) The electrical efficiency of the PV module can be determined using the following equation: ηele=ηΓ(1−γ(Tc−Tr)) |
Electrical, thermal and combined Photovoltaic efficiency. |
Gangadevi et al. [43] | ■ Experimental Work ■ Hybrid PV/Spiral flow thermal collector system |
ηpvt≅Eth+EelEin=>ηpvt=∫t2t1(AcE″th+ApvE″el)dtAc∫t2t1(G″eff)dt=ηth+r.ηel where Ac—area of the collector, Apv—area of PV cells and r—packing factor given as Apv/Ac |
Electrical, thermal and overall PVT efficiency. |
Chandrasekar et al. [44] | ■ Experimental Work | The efficiency of the PV module (η) is calculated by : η=PAI(t) Probable errors in the variables of the problem are calculated which is further used to estimate the uncertainties associated with experimental results as: ΔPP=[(ΔV)V)2+(ΔII)2]0.5 |
Electrical Efficiency |
An et al. [45] | ■ Experimental ■ Concentrating PV/T collector |
η=(ˉPpv+ˉPth)/ˉGCA where, η is overall efficiency, ˉPpv is average electrical power, ˉPth is average thermal power, ˉG is Average solar irradiance, C is concentration ratio and A is Area of Cell unit. δx=Max(xi−ˉx)ˉx where, δx is Relative error, xi is testing value and x is average value |
Overall efficiency |
Xu and Kleinstreuer [46] | ■ CPV/T system ■ Experimntal and numerical study |
The electrical efficiency of the CPV/T system is defined as the ηel=Gec.ηopt.ηcell.ηinv−WsysGrec where ηopf is the optical efficiency, ηce// is the cell efficiency, ηjnv. Is the electrical inverter efficiency, and Wsys is the electrical power required for running the CPV/T system |
Electrical efficiency Overall efficiency |
Hjerrild et al. [47] | ■ Experimental Study ■ Hybrid Ag-SiO2 /Carbon nanofluids |
The value of w(worthfactor) is derived from the relative value of electricity and thermal energy and the fact that thermal power plants typically convert three units of heat into one unit of electricity MF=w∗PPV+PThw∗PPV,nofilteer |
PV efficiency |
Taylor et al. [49] | ■ Experimental Study ■ Optical Fibres in PVT systems |
Nanofluid is used as a bandpass filter, overall efficiency is given by η=∫longλshortλEλTλdλ)∫longλshortλEλdλ)−∫shortλ0EλTλdλ)∫shortλ0Eλdλ)−∫4μmlongλEλTλdλ)∫4μmlongλEλdλ) where Eλ is the is the amount of solar irradiance per unit wavelength. A perfectly transparent (T=1) filter between the short and long edges which is also perfectly absorbing (T=0) outside of that range, will achieve an efficiency of 1. |
Overall efficiency |
Xu and Kleinstreuer [57] | ■ Experimental study ■ Numerical Study |
Sgen(themal)=kT2[(∂T∂X)2+(∂T∂y)2] Sgen (frictional) =Sgen(frictiona1)+Sgen(frictiona1)′=μT(∂ˉu∂y)2+μTΦ′ |
Overall system efficiency |
Authors | Type of Study and System | Mathematical Modelling of the system | Parameters Evaluated |
Jing et al. [32] | ● CPVT system. ● Numerical study |
PV/T system overall exergetic efficiency is defined as, Exoverall=Eth+EeleEsun=ηth(1−TenTout)+ExelEsun |
Exergetic efficiency |
Al-Waeli et al. [36] | ● Photovoltaic thermal PV/T collectors | The total efficiency (ηr)=the thermal efliciency + the electrical efficiency ηt=ηthermal+ηelectrical=Qu+PIs×At where At—the total area of the panel and collector. eR=[(∂R∂V1e1)2+(∂R∂V2e2)2+…+(∂R∂Vnen)2]0.5 where, eR: uncertainty in the results, R: a given function of the independent variables y1,y2,…,yΠ or R=R(V1,V2,…,Vn) and ei: uncertainty interval in the nth variable. |
Overall system efficiency |
Lari et al. [39] | ● Numerical simulations using ANSYS 15.0 ● Mesh generation using GAMBIT |
Computational Fluid Dynamics on PVT panels followed by extensive economic analysis was carried out in this study. | Thermal and Electrical efficiency Economic Analysis |
Khanjari et al. [56] | ● Numerical ● Sheet and tube PV/T system |
Computational fluid dynamics (CFD) employing conjugate heat transfer model is used for estimating the efficiencies. | Heat transfer coefficient |
Hassani et al. [50] | ● Physical modelling ● Electrical Modelling |
PVT system overall efficiency is estimated as: ηov=ηel+J(2∑i=1(1−T0Tn,i)ηth,i where i represents the channel number, (1−T0/Tn,i) is the Carnot efficiency, K - usefull fraction of thermal energy taken as 0.5, Thermal exergy efficiency—KΣ2i=1(1−T0Tn,i)ηth,i |
Electrical output Thermal Exergy output |
Saroha et al. [59] | ● Theoretical & Numerical Modelling ● Indoor hybrid PV/T system |
In this study, a nanofluid based PV/T hybrid collector is proposed and numerically modelled. | Thermal and Overall efficiency |
S.No. | Authors | Year | Location | Nano particles | Base fluid | Concentration and size | Nanofluid usage | Results |
1. | Shamani et al. [26] | 2016 | Malaysia | SiO2, TiO2 and SiC | Water | 0.5, 1, 2 wt.% | Coolant | SiC nanofluid had the highest PV/T electrical efficiency of 13.52% followed by TiO2 and SiO2 nanofluids. |
2. | Yun and Qunzhi [27] | 2012 | China | MgO | Water | 0.02%wt. 0.06% wt. 0.10% wt. | Coolant | The designed PV/T system having a 2 mm thick liquid layer had efficiency above 60%. |
3. | Michael and Iniyan [28] | 2015 | India | CuO | Water | 0.05 vol%, 75 nm | Coolant | Improvement in the thermal efficiency upto 45.76% compared to water. |
4. | Rejeb et al. [29] | 2015 | TunisiaIran France | Al2O3 and Cu | Water & Ethylene glycol | 0.001–1.5%, 10 nm | Coolant | The best thermal and electrical efficiency was attained by using Cu/water in the place of Cu/ethylene glycol and same as for Al2O3/water in comparison with Al2O3/ethylene glycol. |
5. | Sardarabadi et al. [30] | 2014 | Iran | SiO2 | Water | 1, 3 wt %, 11–14 nm | Coolant | Increase of 7.6% in thermal efficiency for nanofluid with 1% wt and for 3% wt the thermal efficiency was increased by 12.8%. The overall efficiency was also upgraded with the use of nanofluid, for 1% wt the increase was 3.6% whereas it goes to 7.9% in case of 3% wt. |
6. | Al-Waeli et al. [31] | 2016 | Malaysia | SiC | Water | 3 wt.% | Coolant | The electrical efficiency was increased up-to 24.1%. The overall efficiency of the PV/T system was about 88.9%. |
7. | Jing et al. [32] | 2015 | China | SiO2 | Water | 0.5, 1, 2 vol%, 5, 10, 25, 50 nm | Coolant | Exergetic efficiency was enhanced by 9.5%. |
8. | Radwan et al. [33] | 2016 | Egypt | Al2O3 and SiC | Water | 0–4vol.%, 20 nm | Coolant | SiC was found to be more effective than aluminium oxide Al2O3 nanofluid. |
9. | Karami and Rahimi et al.[34] | 2013 | Iran | Boehmite (AlOOH.xH2O) | Water | 0.01, 0.1, 0.3 wt%, 5–10 nm | Coolant | The electrical efficiencies for straight and helical systems were 20.57% and 37.67% respectively. |
10. | Hussien et al. [35] | 2015 | Iraq | Al2O3 | Water | 0.1–0.5% 30 nm | Coolant | The electrical efficiency increased to 12.1% and thermal efficiency increased to 34.4%. |
11. | Al-Waeli et al. [36] | 2017 | Malaysia | Al2O3, CuO and SiC | Water | 0.5, 1, 2, 3, and 4% by vol Al2O3:30–60 nm CuO: 35–45 nm SiC: 45–65 nm | Coolant | The thermal efficiency enhanced by 1.96%, 3.42% and 4.8% by using nanofluid Al2O3, CuO and SiC respectively. |
12. | Sardarabadi et al. [37] | 2016 | Iran | Al2O3, TiO2 and ZnO | Water | Al2O3: 2 wt.%, 20nm TiO2: 2 wt.%, 10-30 nm ZnO: 2wt.%, 10–25 nm | Coolant | The electrical efficiency for deionized water, TiO2, ZnO and Al2O3 was enhanced by 5.48%, 6.54%, 6.46% and 6.36% respectively. |
13. | Bellos et al. [38] | 2017 | Greece | Cu | Water | 0%–4% | Coolant | Electrical efficiency enhanced by 1.49% and the thermal efficiency was increased by 4.35%. |
14. | Lari et al. [39] | 2017 | Saudi Arabia | Ag | Water | 0.5 vol%, 10 nm | Coolant | There was an increase in electrical output by 8.5% and an increase in thermal output by 13%. |
15. | Sardarbadi et al. [40] | 2017 | Iran | ZnO | Water | 0.2% wt. 50 nm | Coolant | The results showed an increase of 13% in the electrical efficiency and 5% in thermal efficiency as compared to the conventional PV module. |
16. | Shamani et al. [41] | 2016 | Malaysia | SiC | Water | Phase change material | The electrical efficiency of the system was enhanced from 8.77% to 13.52% and the overall efficiency of the system was increased from 8.4% to 13.1%. | |
17. | Hasan et al. [42] | 2016 | Malaysia | SiC, TiO2 and SiO2 | Water | 1 wt.% | Coolant | The electrical, thermal and overall efficiency of the system in case of SiC nanoparticle was documented 12.75%, 85% and 97.75% respectively. |
18. | Gangadevi et al. [43] | 2017 | India | Al2O3 | Water | 1% wt and 2% wt. 50 nm | Coolant | Electrical, thermal and overall efficiency enhanced by 13%, 45% and 58% respectively. |
19. | Chandrasekar et al. [44] | 2012 | India | Al2O3 and CuO | Water | 0.1 vol.% | Coolant | The maximum electrical efficiency when no cooling was provided was 9% and which was increased to 9.4% and 9.8% when cooling fluid CuO nanofluid and Al2O3 nanofluid respectively was used. |
20. | An et al. [45] | 2016 | China | Cu9S5 | Water | 22.3, 44.6, 89.2 ppm, 60.2 nm | Spectral filter | The overall efficiency of the PV/T system increased upto 17%. |
21. | Xu and Kleinstreuer [46] | 2014 | U.S | SiO2 | Water | Optical filter | At 62 ℃ the overall efficiency of the system reached 70% and electrical efficiency was found to be 11% whereas the thermal efficiency was 59%. | |
22. | Hjerrild et al. [47] | 2016 | Australia | Ag-SiO2 | Water | Ag-SiO2: 0.001, 0.003, 0.006, 0.026 wt.% 17.5 nm | Spectral filter | The increase in overall efficiency was about 30% on using the Ag-SiO2. |
23. | Taylor et al. [49] | 2012 | Australia | SiO2 | Water and Therminol VP-1 | Therminol VP-1: 0–0.01 wt.% 20–50 nm | Spectral filter | An enhancement of 4%–8% in the efficiency of the PV/T system as compared to the water based PV/T system. |
24. | Khanjari et al. [56] | 2016 | Iraq | Al2O3 & Ag | Water | 0–12%, 50 nm | Coolant | For Alumina-water heat transfer coefficient increased by 12%. For Ag-water heat transfer coefficient increased by 43%. |
25. | Xu and Kleinstreuer [57] | 2014 | U.S | Al2O3 | Water | 5 vol.%, 38.4 nm | Coolant | The system efficiency achieved was 20%. |
26. | Hassani et al. [50] | 2016 | Malaysia | Ag | Water and Therminol VP-1 | 0.001–1.5 vol.%, 10 nm | Spectral filter & coolant | Electrical efficiency was increased by 9% and 5.6% respectively and the overall efficiency of the system was improved to 30% and 24.4% respectively for GaAs and Si cells. |
27. | Saroha et al. [59] | 2015 | India | Au and Ag | Water | Au: 0.00019, 0.0093 vol.% 5, 10 nm Ag: 0.00025, 0.00193 vol.%, 5, 10 nm | Spectral filter and coolant | Highest thermal and overall efficiency is achived using Ag-water nanofluid. |
Authors | Type of Study and System | Mathematical Modelling of the system | Parameters Evaluated |
Al-Shamani et al. [26] | ■ Experimental. ■ PV/T system having stainless steel tube absorber |
ηel=ηr(1−β(Tpm−Tr)) where, ηel is the electrical efficiency, ηr is the reference efficiency of the PV module, β is the temperature coefficient, Tpm is the temperature of the solar cells and Tr is the reference temperature. WR={(∂R∂x1w1)2+(∂R∂x2w2)2+…+(∂R∂xnwn)2}1/2 where, R is uncertainty - function of the independent variables x1, x2, xn and w1, w2, wn represents the uncertainties associated with independent variables. |
Electrical efficiency |
Yun and Qunzhi [27] | ■ Experimental ■ PV/T system |
ηpvt=ηth+ηe=Qu+PIs×At,Qu=˙mCP(To−Ti) ˙m is the mass flow rate, Is is the solar intensity, Ac is the collector area, Cp is the specific heat, To and Ti are outlet and inlet fluid temperatures respectively |
Overall efficiency |
Michael and Iniyan [28] | ■ Experimental and Numerical ■ Single rectangular absorber PV/T |
ηth=QuIs×At where, ηth is the thermal efficiency [(Uηlηl)2]thεrm=(U˙m˙m)2+(UGG)2+(UTT)2 where, U—denotes uncertainty, ˙m—mass flow rate of water, T—temperature difference between the outlet and inlet fluid and G—incident global solar radiation |
Thermal Efficiency |
Rejeb et al. [29] | ■ Experimental and Numerical ■ Sheet and tube PV/T |
Heat transfer modeling of collector was developed in FORTRAN language, based on the finite volume method. ηth=QuIs×At and ηel=ηo(1−β(Tpv−298)) [(Uηlηl)2]thεrm=(U˙m˙m)2+(UGG)2+(UTT)2 [(Uηiηl)2]elec=(UvV)2+(Ull)2+(UGG)2 where, V is voltage and I is current |
Thermal Efficiency Electrical Efficiency |
Sardarabadi et al. [30] | ■ Experimental study ■ Phase change material ■ Comparative Analysis |
ηpvt, is the overall efficiency which is the sum of the thermal and electrical efficiencies (ηth and ηel, respectively): ηpvt≅Eth+EelEin=>ηpvt=∫t2t1(AcE″th+ApvE″el)dtAc∫t2t1(Geff)dt=ηth+r.ηel where Ac—area of the collector, Apv—area of PV cells and r—packing factor. δR=√(δRδv1δv1)2+(δRδv2δv2)2+⋯+(δRδvnδvn)2 where δR is the uncertainty of function R. |
Thermal efficiency |
Al-Waeli et al. [31] | ■ Experimental study | The total efficiency (ηr)=the thermal efliciency+the electrical efficiency ηt=ηthermal+ηelectrical=Qu+PIs×At where, At the total area of the panel and collector. Change in the operations by: eR=[(∂R∂V1e1)2+(∂R∂V2e2)2+…+(∂R∂Vnen)2]0.5 where, eR: uncertainty in the results, R: a given function of the independent variables y1,y2,…,yΠ or R=R(V1,V2,…,Vn), ei: uncertainty interval in the nth variable. |
Electrical Thermal efficiency |
Radwan et al. [33] | ■ Experimental study | The solar cell generated electrical energy per unit area, thermal efficiency, friction factor and net produced power are calculated as follows: Psc=ηscβscτgG(t).CR, ηth=PthG.CR.Asc and Pfriction=ΔP.mρnf |
Thermal efficiency |
Karami and Rahimi et al. [34] | ■ Experimental study | The values of friction factor ω were calculated using the following equation: f=ΔpchDh2ρf.u2m.Lch where, Dch—hydraulic diameter. |
Electrical efficiency. |
Hussien et al. [35] | ■ Hybrid PVT systems. ■ Experimental study |
Hybrid PVT system overall efficiency is give as: ηtotal=ηth+ηele=mCp∫(To−Ti)dt+∫VIdtAo∫(t)dt |
Overall efficiency. |
Al-Waeli et al. [36] | ■ Photovoltaic thermal PV/T collectors | The total efficiency (ηr)=the thermal efliciency+the electrical efficiency ηt=ηthermal+ηelectrical=Qu+PIs×At where At—the total area of the panel and collector. eR=[(∂R∂V1e1)2+(∂R∂V2e2)2+…+(∂R∂Vnen)2]0.5 where, eR: uncertainty in the results, R: a given function of the independent variables y1,y2,…,yΠ or R=R(V1,V2,…,Vn) and ei: uncertainty interval in the nth variable. |
Overall system efficiency |
Sardarabadi et al. [37] | ■ Experimental and Numerical study | Electrical efficiency of PVT system can be estimated as ηclcc=Ee1ecEin=Voc×Jsc×FFGeffective where V—open circuit voltage and Isc—short circuit current and FF—Fill factor eR=[(∂R∂V1e1)2+(∂R∂V2e2)2+…+(∂R∂Vnen)2]0.5 where, eR: uncertainty in the results, R: a given function of the independent variables y1,y2,…,yΠ or R=R(V1,V2,…,Vn), ei: uncertainty interval in the nth variable. |
Electrical efficiency |
Bellos et al. [38] | ■ Experimental study ■ Performance Analysis |
The total exergy based on daily solar irradiation (Zs) is evaluated using following model: Zs=Ac.HT.(1−43.(TamTsun)+13.(TamTsun)4) |
Thermal efficiency Electrical efficiency |
Sardarbadi et al. [40] | ■ Experimental study ■ Phase change material. ■ Comparative Analysis |
Summation of the thermal and electrical efficiencies constitutes overall efficiency given by: ηpvt≅Eth+EelEin=>ηpvt=∫t2t1(AcE″th+ApvE″el)dtAc∫t2t1(G″eff)dt=ηth+r.ηel where, Ac—area of the collector, Apv—area of PV cells, r—packing factor defined as Apv/Ac. δR=√(δRδv1δv1)2+(δRδv2δv2)2+⋯+(δRδvnδvn)2 where δR—uncertainty associated with R, dvi —uncertainty associated with vi |
Thermal efficiency Electrical efficiency |
Shamani et al. [41] | ■ Experimental Study ■ Grid connected PVT systems |
The PV module efficiency is determined using: ηPV=(EDCHr∗Ac)∗100 (%) The system efficiency is given as: ηsys=(EACHr∗Ac)∗100 (%) The inverter efficiency is given as: ηinv=(EACEDC)∗100 (%) |
Overall efficiency Electrical efficiency |
Hasan et al. [42] | ■ Experimental study ■ Jet array nanofluids impingement on PVT collectors |
Overall thermal efficiency of the PVT system is given by: ηthι=FR(τα)−FRUL(Ti−TaGT) The electrical efficiency of the PV module can be determined using the following equation: ηele=ηΓ(1−γ(Tc−Tr)) |
Electrical, thermal and combined Photovoltaic efficiency. |
Gangadevi et al. [43] | ■ Experimental Work ■ Hybrid PV/Spiral flow thermal collector system |
ηpvt≅Eth+EelEin=>ηpvt=∫t2t1(AcE″th+ApvE″el)dtAc∫t2t1(G″eff)dt=ηth+r.ηel where Ac—area of the collector, Apv—area of PV cells and r—packing factor given as Apv/Ac |
Electrical, thermal and overall PVT efficiency. |
Chandrasekar et al. [44] | ■ Experimental Work | The efficiency of the PV module (η) is calculated by : η=PAI(t) Probable errors in the variables of the problem are calculated which is further used to estimate the uncertainties associated with experimental results as: ΔPP=[(ΔV)V)2+(ΔII)2]0.5 |
Electrical Efficiency |
An et al. [45] | ■ Experimental ■ Concentrating PV/T collector |
η=(ˉPpv+ˉPth)/ˉGCA where, η is overall efficiency, ˉPpv is average electrical power, ˉPth is average thermal power, ˉG is Average solar irradiance, C is concentration ratio and A is Area of Cell unit. δx=Max(xi−ˉx)ˉx where, δx is Relative error, xi is testing value and x is average value |
Overall efficiency |
Xu and Kleinstreuer [46] | ■ CPV/T system ■ Experimntal and numerical study |
The electrical efficiency of the CPV/T system is defined as the ηel=Gec.ηopt.ηcell.ηinv−WsysGrec where ηopf is the optical efficiency, ηce// is the cell efficiency, ηjnv. Is the electrical inverter efficiency, and Wsys is the electrical power required for running the CPV/T system |
Electrical efficiency Overall efficiency |
Hjerrild et al. [47] | ■ Experimental Study ■ Hybrid Ag-SiO2 /Carbon nanofluids |
The value of w(worthfactor) is derived from the relative value of electricity and thermal energy and the fact that thermal power plants typically convert three units of heat into one unit of electricity MF=w∗PPV+PThw∗PPV,nofilteer |
PV efficiency |
Taylor et al. [49] | ■ Experimental Study ■ Optical Fibres in PVT systems |
Nanofluid is used as a bandpass filter, overall efficiency is given by η=∫longλshortλEλTλdλ)∫longλshortλEλdλ)−∫shortλ0EλTλdλ)∫shortλ0Eλdλ)−∫4μmlongλEλTλdλ)∫4μmlongλEλdλ) where Eλ is the is the amount of solar irradiance per unit wavelength. A perfectly transparent (T=1) filter between the short and long edges which is also perfectly absorbing (T=0) outside of that range, will achieve an efficiency of 1. |
Overall efficiency |
Xu and Kleinstreuer [57] | ■ Experimental study ■ Numerical Study |
Sgen(themal)=kT2[(∂T∂X)2+(∂T∂y)2] Sgen (frictional) =Sgen(frictiona1)+Sgen(frictiona1)′=μT(∂ˉu∂y)2+μTΦ′ |
Overall system efficiency |
Authors | Type of Study and System | Mathematical Modelling of the system | Parameters Evaluated |
Jing et al. [32] | ● CPVT system. ● Numerical study |
PV/T system overall exergetic efficiency is defined as, Exoverall=Eth+EeleEsun=ηth(1−TenTout)+ExelEsun |
Exergetic efficiency |
Al-Waeli et al. [36] | ● Photovoltaic thermal PV/T collectors | The total efficiency (ηr)=the thermal efliciency + the electrical efficiency ηt=ηthermal+ηelectrical=Qu+PIs×At where At—the total area of the panel and collector. eR=[(∂R∂V1e1)2+(∂R∂V2e2)2+…+(∂R∂Vnen)2]0.5 where, eR: uncertainty in the results, R: a given function of the independent variables y1,y2,…,yΠ or R=R(V1,V2,…,Vn) and ei: uncertainty interval in the nth variable. |
Overall system efficiency |
Lari et al. [39] | ● Numerical simulations using ANSYS 15.0 ● Mesh generation using GAMBIT |
Computational Fluid Dynamics on PVT panels followed by extensive economic analysis was carried out in this study. | Thermal and Electrical efficiency Economic Analysis |
Khanjari et al. [56] | ● Numerical ● Sheet and tube PV/T system |
Computational fluid dynamics (CFD) employing conjugate heat transfer model is used for estimating the efficiencies. | Heat transfer coefficient |
Hassani et al. [50] | ● Physical modelling ● Electrical Modelling |
PVT system overall efficiency is estimated as: ηov=ηel+J(2∑i=1(1−T0Tn,i)ηth,i where i represents the channel number, (1−T0/Tn,i) is the Carnot efficiency, K - usefull fraction of thermal energy taken as 0.5, Thermal exergy efficiency—KΣ2i=1(1−T0Tn,i)ηth,i |
Electrical output Thermal Exergy output |
Saroha et al. [59] | ● Theoretical & Numerical Modelling ● Indoor hybrid PV/T system |
In this study, a nanofluid based PV/T hybrid collector is proposed and numerically modelled. | Thermal and Overall efficiency |
S.No. | Authors | Year | Location | Nano particles | Base fluid | Concentration and size | Nanofluid usage | Results |
1. | Shamani et al. [26] | 2016 | Malaysia | SiO2, TiO2 and SiC | Water | 0.5, 1, 2 wt.% | Coolant | SiC nanofluid had the highest PV/T electrical efficiency of 13.52% followed by TiO2 and SiO2 nanofluids. |
2. | Yun and Qunzhi [27] | 2012 | China | MgO | Water | 0.02%wt. 0.06% wt. 0.10% wt. | Coolant | The designed PV/T system having a 2 mm thick liquid layer had efficiency above 60%. |
3. | Michael and Iniyan [28] | 2015 | India | CuO | Water | 0.05 vol%, 75 nm | Coolant | Improvement in the thermal efficiency upto 45.76% compared to water. |
4. | Rejeb et al. [29] | 2015 | TunisiaIran France | Al2O3 and Cu | Water & Ethylene glycol | 0.001–1.5%, 10 nm | Coolant | The best thermal and electrical efficiency was attained by using Cu/water in the place of Cu/ethylene glycol and same as for Al2O3/water in comparison with Al2O3/ethylene glycol. |
5. | Sardarabadi et al. [30] | 2014 | Iran | SiO2 | Water | 1, 3 wt %, 11–14 nm | Coolant | Increase of 7.6% in thermal efficiency for nanofluid with 1% wt and for 3% wt the thermal efficiency was increased by 12.8%. The overall efficiency was also upgraded with the use of nanofluid, for 1% wt the increase was 3.6% whereas it goes to 7.9% in case of 3% wt. |
6. | Al-Waeli et al. [31] | 2016 | Malaysia | SiC | Water | 3 wt.% | Coolant | The electrical efficiency was increased up-to 24.1%. The overall efficiency of the PV/T system was about 88.9%. |
7. | Jing et al. [32] | 2015 | China | SiO2 | Water | 0.5, 1, 2 vol%, 5, 10, 25, 50 nm | Coolant | Exergetic efficiency was enhanced by 9.5%. |
8. | Radwan et al. [33] | 2016 | Egypt | Al2O3 and SiC | Water | 0–4vol.%, 20 nm | Coolant | SiC was found to be more effective than aluminium oxide Al2O3 nanofluid. |
9. | Karami and Rahimi et al.[34] | 2013 | Iran | Boehmite (AlOOH.xH2O) | Water | 0.01, 0.1, 0.3 wt%, 5–10 nm | Coolant | The electrical efficiencies for straight and helical systems were 20.57% and 37.67% respectively. |
10. | Hussien et al. [35] | 2015 | Iraq | Al2O3 | Water | 0.1–0.5% 30 nm | Coolant | The electrical efficiency increased to 12.1% and thermal efficiency increased to 34.4%. |
11. | Al-Waeli et al. [36] | 2017 | Malaysia | Al2O3, CuO and SiC | Water | 0.5, 1, 2, 3, and 4% by vol Al2O3:30–60 nm CuO: 35–45 nm SiC: 45–65 nm | Coolant | The thermal efficiency enhanced by 1.96%, 3.42% and 4.8% by using nanofluid Al2O3, CuO and SiC respectively. |
12. | Sardarabadi et al. [37] | 2016 | Iran | Al2O3, TiO2 and ZnO | Water | Al2O3: 2 wt.%, 20nm TiO2: 2 wt.%, 10-30 nm ZnO: 2wt.%, 10–25 nm | Coolant | The electrical efficiency for deionized water, TiO2, ZnO and Al2O3 was enhanced by 5.48%, 6.54%, 6.46% and 6.36% respectively. |
13. | Bellos et al. [38] | 2017 | Greece | Cu | Water | 0%–4% | Coolant | Electrical efficiency enhanced by 1.49% and the thermal efficiency was increased by 4.35%. |
14. | Lari et al. [39] | 2017 | Saudi Arabia | Ag | Water | 0.5 vol%, 10 nm | Coolant | There was an increase in electrical output by 8.5% and an increase in thermal output by 13%. |
15. | Sardarbadi et al. [40] | 2017 | Iran | ZnO | Water | 0.2% wt. 50 nm | Coolant | The results showed an increase of 13% in the electrical efficiency and 5% in thermal efficiency as compared to the conventional PV module. |
16. | Shamani et al. [41] | 2016 | Malaysia | SiC | Water | Phase change material | The electrical efficiency of the system was enhanced from 8.77% to 13.52% and the overall efficiency of the system was increased from 8.4% to 13.1%. | |
17. | Hasan et al. [42] | 2016 | Malaysia | SiC, TiO2 and SiO2 | Water | 1 wt.% | Coolant | The electrical, thermal and overall efficiency of the system in case of SiC nanoparticle was documented 12.75%, 85% and 97.75% respectively. |
18. | Gangadevi et al. [43] | 2017 | India | Al2O3 | Water | 1% wt and 2% wt. 50 nm | Coolant | Electrical, thermal and overall efficiency enhanced by 13%, 45% and 58% respectively. |
19. | Chandrasekar et al. [44] | 2012 | India | Al2O3 and CuO | Water | 0.1 vol.% | Coolant | The maximum electrical efficiency when no cooling was provided was 9% and which was increased to 9.4% and 9.8% when cooling fluid CuO nanofluid and Al2O3 nanofluid respectively was used. |
20. | An et al. [45] | 2016 | China | Cu9S5 | Water | 22.3, 44.6, 89.2 ppm, 60.2 nm | Spectral filter | The overall efficiency of the PV/T system increased upto 17%. |
21. | Xu and Kleinstreuer [46] | 2014 | U.S | SiO2 | Water | Optical filter | At 62 ℃ the overall efficiency of the system reached 70% and electrical efficiency was found to be 11% whereas the thermal efficiency was 59%. | |
22. | Hjerrild et al. [47] | 2016 | Australia | Ag-SiO2 | Water | Ag-SiO2: 0.001, 0.003, 0.006, 0.026 wt.% 17.5 nm | Spectral filter | The increase in overall efficiency was about 30% on using the Ag-SiO2. |
23. | Taylor et al. [49] | 2012 | Australia | SiO2 | Water and Therminol VP-1 | Therminol VP-1: 0–0.01 wt.% 20–50 nm | Spectral filter | An enhancement of 4%–8% in the efficiency of the PV/T system as compared to the water based PV/T system. |
24. | Khanjari et al. [56] | 2016 | Iraq | Al2O3 & Ag | Water | 0–12%, 50 nm | Coolant | For Alumina-water heat transfer coefficient increased by 12%. For Ag-water heat transfer coefficient increased by 43%. |
25. | Xu and Kleinstreuer [57] | 2014 | U.S | Al2O3 | Water | 5 vol.%, 38.4 nm | Coolant | The system efficiency achieved was 20%. |
26. | Hassani et al. [50] | 2016 | Malaysia | Ag | Water and Therminol VP-1 | 0.001–1.5 vol.%, 10 nm | Spectral filter & coolant | Electrical efficiency was increased by 9% and 5.6% respectively and the overall efficiency of the system was improved to 30% and 24.4% respectively for GaAs and Si cells. |
27. | Saroha et al. [59] | 2015 | India | Au and Ag | Water | Au: 0.00019, 0.0093 vol.% 5, 10 nm Ag: 0.00025, 0.00193 vol.%, 5, 10 nm | Spectral filter and coolant | Highest thermal and overall efficiency is achived using Ag-water nanofluid. |