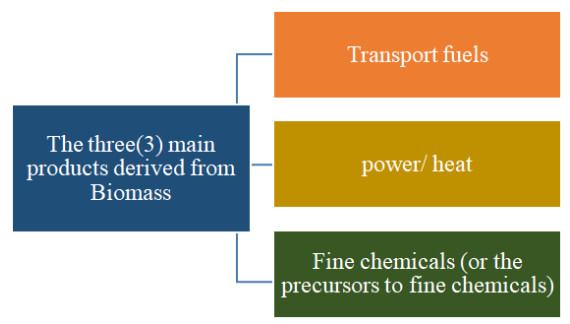
Citation: Aderemi T. Adeleye, Hitler Louis, Ozioma U. Akakuru, Innocent Joseph, Obieze C. Enudi, Dass P. Michael. A Review on the conversion of levulinic acid and its esters to various useful chemicals[J]. AIMS Energy, 2019, 7(2): 165-185. doi: 10.3934/energy.2019.2.165
[1] | Douglas Queiroz Santos, Ana Paula de Lima, Maíra Martins Franco, David Maikel Fernandes, Waldomiro Borges Neto, José Domingos Fabris . Evaluation and Characterization of Biodiesels Obtained Through Ethylic or Methylic Transesterification of Tryacylglicerides in Corn Oil. AIMS Energy, 2014, 2(2): 183-192. doi: 10.3934/energy.2014.2.183 |
[2] | Lifita N. Tande, Valerie Dupont . Autothermal reforming of palm empty fruit bunch bio-oil: thermodynamic modelling. AIMS Energy, 2016, 4(1): 68-92. doi: 10.3934/energy.2016.1.68 |
[3] | Augustine O. Ayeni, Michael O. Daramola, Oluranti Agboola, Ayodeji A. Ayoola, Rasheed Babalola, Babalola A. Oni, Julius O. Omodara, Deinma T. Dick . A comparative evaluation of fermentable sugars production from oxidative, alkaline, alkaline peroxide oxidation, dilute acid, and molten hydrate salt pretreatments of corn cob biomass. AIMS Energy, 2021, 9(1): 15-28. doi: 10.3934/energy.2021002 |
[4] | Fenglei Qi, Mark Mba Wright . A novel optimization approach to estimating kinetic parameters of the enzymatic hydrolysis of corn stover. AIMS Energy, 2016, 4(1): 52-67. doi: 10.3934/energy.2016.1.52 |
[5] | Bárbara Gonçalves Rocha, Alice Lopes Macedo, Bárbara Rodrigues Freitas, Priscylla Caires de Almeida, Vany P. Ferraz, Luis Carlos Duarte Cavalcante, José Domingos Fabris, José Domingos Ardisson . Magnetic fraction from phosphate mining tailings as heterogeneous catalyst for biodiesel production through transesterification reaction of triacylglycerols in bio-oil. AIMS Energy, 2017, 5(5): 864-872. doi: 10.3934/energy.2017.5.864 |
[6] | Hasanudin Hasanudin, Wan Ryan Asri, Utari Permatahati, Widia Purwaningrum, Fitri Hadiah, Roni Maryana, Muhammad Al Muttaqii, Muhammad Hendri . Conversion of crude palm oil to biofuels via catalytic hydrocracking over NiN-supported natural bentonite. AIMS Energy, 2023, 11(2): 197-212. doi: 10.3934/energy.2023011 |
[7] | Maria del Pilar Rodriguez, Ryszard Brzezinski, Nathalie Faucheux, Michèle Heitz . Enzymatic transesterification of lipids from microalgae into biodiesel: a review. AIMS Energy, 2016, 4(6): 817-855. doi: 10.3934/energy.2016.6.817 |
[8] | Conrad Omonhinmin, Enameguono Olomukoro, Ayodeji Ayoola, Evans Egwim . Utilization of Moringa oleifera oil for biodiesel production: A systematic review. AIMS Energy, 2020, 8(1): 102-121. doi: 10.3934/energy.2020.1.102 |
[9] | Angelo Minotti . Hybrid energy converter based on swirling combustion chambers: the hydrocarbon feeding analysis. AIMS Energy, 2017, 5(3): 506-516. doi: 10.3934/energy.2017.3.506 |
[10] | Sandra M. Damasceno, Vanny Ferraz, David L. Nelson, José D. Fabris . Selective adsorption of fatty acid methyl esters onto a commercial molecular sieve or activated charcoal prepared from the Acrocomia aculeata cake remaining from press-extracting the fruit kernel oil. AIMS Energy, 2018, 6(5): 801-809. doi: 10.3934/energy.2018.5.801 |
Abbreviations: Acac: Acetylacetonate; LA: Levulinic acid; LE: Levulinic esters; MTHF: Methyltetrahydrofuran; HMF: Hydroxymethylfurfural; 2-BO: 2-Butanol; 2-PO: 2-Pentanol; GVL: γ- valerolactone; BTU: British thermal unit (Btu); PDO: 1, 4-pentadiol; TPPMS: Monosulfonated Triphenylphosphine; TPPTS: Trisodium 3, 3', 3-phosphinetriyltribenzenesulfonate; BVO: BaeyerVilliger Oxidation
The present energy system is unsustainable as a result of equity issues as well as environmental, economic, increase in global populations and geopolitical concerns that have implications far into the future complemented by the finite and depletion of petroleum based resources on earth. At present, fossil fuels still remain key player contributing greater percent to energy supply of the entire world [1]. Recent reports have it that, greenhouse gas emission that emerges from the use of petroleum-based resources is one of the alarming challenges confronting the human society [2]. The world energy demand is predicted to rise up to 48% by the year 2040, as reported by the U.S. Department of energy, representing a projection from 549 to 815 quadrillion Btu consumption [3]. Petroleum-based resources are undoubtedly the culprit of the world's major energy demand since the mid-20th century; this has further increased in recent years, owing to the meteoric growth in technology. Also, the nonrenewable nature of fossil fuels, and the environmental concern associated with its use have prompt the search for eco-friendly and sustainable alternative energy sources, in order to shift from the sole dependence on fossils resources, and meet the global energy demand alongside, without conflicting the ecosystem. A promising substitute that can reduce the high dependence on fossil fuel is the use of biomass, a renewable organic and abundant resources, to ensure a sustainable future [4]. Lignocellulose, the most abundant form of biomass, is composed of three primary components; cellulose (40–50 wt%), hemicellulose (25–30 wt%), and lignin (15–30 wt%), a phenolic polymer. Woody, herbaceous plants, and other crop residues like sugarcane bagasse, wheat straw, are suitable lignocellulosic feedstocks applied in the biorefinery process, to proffer valuable applications that can be derived from the petroleum-based source. Approximately, 170 billion metric tons of lignocellulosics production are made available yearly, a little amount of this has been used for chemicals and energy syntheses, due to the complexity of lignocellulosic biomass, it is relatively not viable to chemical transformation [5]. But, due to the great advantages associated with the utilization of biomass, serious scientific research attention has been put in this field by modern chemists. There are various available methods, through which biomass can be converted to different viable products. The choice of method is dependent on the nature and application of the end product desired. Power/heat, transport fuels, and fine chemicals (or their precursors) are the three major derivable products from biomass (Figure 1). The utilization of biomass products is possible in other to reduce the alarming consumption of non-renewable based sources, and to combat the deteriorating effects of fossil consumption on the environment. Biomass is considered as a potential substitute, in order to reduce the societal dependence on fossil resources. The use of biomass as a fuel produces an insignificant amount of CO2, which is equivalent to the amount consumed by plants during photosynthesis, a natural process during which plants that make up the biomass reconverts CO2 to carbohydrates. Hence, if biomass is continuously used as the resources for subsequent conversion, there will be relative, no increment in the amount of natural CO2 in the atmosphere, this uncommon attribute of biomass is termed 'carbon neutrality'. Biomass can be seen as a suitable replacement for fossil resources with the benefits of reducing CO2 emission. Hence, research on the utilization of biomass very necessary, and have attracted most researchers around the globe, due to rising environmental concern. In addition, comparison with fossil fuels, which are one-way in CO2 emissions, biomass is applicable for its short span of growth, which makes it crucial in a recycling-based society [3,4].
A wide range of chemicals has their precursor compounds (building blocks) made from biomass. There is great possibility associated with the synthesis of these chemicals using biomass, as compared to those available from petroleum-based resources. Furthermore, the synthesis of high-value chemicals can be achieved through the modification of certain species of plants via biotechnology [6]. The difference between the building blocks derived from petroleum and bio-obtained feedstocks is that bio-compounds are highly oxygenated. This offers an added advantage in the use of biomass, because, many end products of petroleum-based industry are oxygenated, but the economic feasibility of various oxygenation process for hydrocarbon is low. Besides, the reagents required for the few available methods are considered toxic, like lead (Pb), chromium (Cr), etc. which causes serious hazard when disposed into the environment [7]. However, highly functionalized bio-derived feedstock is required to be converted to higher energy density-based molecules, or easily applicable precursors that are obtainable synthetically [8,9,10]. 'Building block chemicals' from biomass are referred to as 'platform molecules', they contain several functional groups that could be processed into various useful molecules/value-added products, which could arrest the market in the nearest future, provided other synthetic chemicals can be derived from it. A suitable platform must have a source that is economically stable in other for its derivatives to be cheap. The processes employed in the synthesis of fuels and chemicals mostly involves the complete cracking process, which entails the breakdown of complex petrochemical molecules before the subsequent build up to desired products, this happens with syngas production during the synthesis of alkanes and alcohols. This same process is employed in the conversion of biomass to syngas. The transformation of biomass into chemical building blocks which retains features (like electrophilic and nucleophilic properties) can follow an alternative and exhaustive productive process; this could be employed in subsequent transformations, such that the desired end products are achieved. Such platform chemicals can be processed through chemical or biological methods. Lignocellulosic biomass consists about 40–50% of cellulose, this value is relatively high, coupled with the fact that cellulose is not edible. Hence, its usage does not compete with food supply. Designing processes that can effectively utilize biomass to produce useful chemicals are of economic importance. Besides all other chemicals that are achievable form biomass transformations, 'levulinic acid' is of great importance, hence, the focus of this review. This review is focused on the synthesis of levulinic acid (LA) from γ-valerolactone (GVL), its derivatives and their applications in the various field, with sole attention on the biorefinery. Much attention will be on derivatives like methyltetranhydrofuran (MTHF), a gasoline addictive, ethyl levulinate as a diesel addictive, succinic acid, 2-Butanol (2-BO) and 2-pentanol (2-PO) which are mainly synthesized from levulinic acid (LA). Also, catalysts and catalytic systems for the process would be treated, then, Baeyer-Villiger (BV) oxidation of levulinates will be briefly considered.
The National Renewable Energy Laboratory of the U.S. Department of Energy revealed 12 chemicals in 2004, which is obtainable through biomass transformation. The list of top value-added chemicals which are practically obtainable during biomass transformation is shown in Scheme 1. Different compounds can be derived through the chemo-catalysis of glucose, the product derived is dependent on the catalyst selected and reaction conditions. Gluconic acid is gotten via oxidation, sorbitol through hydrogenation, fructose through isomerization, and 5-hydroxymethylfurfural via dehydration, this can be further transformed into levulinic acid (LA) and formic acid (FA) by hydrolysis. Glucose can be alternatively subjected to bio-catalysis directly, and be enzymatically transformed into other valuable products, e.g., itaconic acid, succinic acid, and 3-hydroxylproponic acid, etc. Scheme 1 [8]. The chemicals which are potentially suitable precursors for finished products enable biomass to be a crucial resource, which could be applied in the synthesis of chemical products that are currently obtainable from petroleum-based source today. Some years ago, the Biofine Corporation designed a technique for the conversion of cellulose into levulinic acid (4-oxopentanoic acid), a potential 'platform chemical'. Levulinic acid can be derived from the oxidation of simple sugars (glucose or fructose) [9,10].
Levulinic acid (LA) has several important applications. Levulinic esters (LE), which is obtained through esterification of LA is applicable as oxygenated fuel. Hydrogenation of LA gives methyl-tetrahydrofuran (MTHF), which is an additive in gasoline. 2-Methyltetrahydrofuran (2-MTHF), which is an alternative fuel, also finds a great application as the electrolyte for lithium rechargeable batteries, 2-MTHF is a derived from levulinic acid. γ-valerolactone and ethyl levulinate are other valuable chemicals that are derived from levulinic acid [13]. There are five carbon atoms in the molecule structure of levulinic acid (LA). 1 mol of levulinic acid can be produced from 1 mol of hexose stoichiometrically. Cellulose, which is one of the major components of biomass passes through degradation, on the course of transformation to levulinic acid (LA). The degradation pathway from cellulose to LA is illustrated in Scheme 2. Firstly, cellulose is hydrolyzed to glucose, and then glucose undergoes isomerization to give fructose, fructose is then dehydrated to give 5-hydroxymethylfurfural (HMF), an intermediate product. Levulinic acid (LA) is then obtained through hydrolysis of HMF in an acidic condition, with the formation of formic acid alongside. Formic acid is applicable in the synthesis of formalin, plasticizers, pharmaceuticals, textiles, and rubber. Formic acid is currently a low-priced commodity, but there are high potentials of its utilization in fuel cells, and also as a starting material for hydrogen synthesis in future. Scheme 3 illustrates the reaction flow for the conversion of pentose sugar to furfural and levulinic acid (LA). LA is potentially a beneficial platform chemical in the biorefinery today [13].
Levulinic acid (LA) reacts with variety of compounds to give products that are applicable in pharmaceutical, biological active material, corrosion inhibitors, adsorbents, personal care products, batteries, coatings, polymers, anti-freeze agents, electronics, photography, plasticizers, flavoring agents, antifouling compounds, fuels, herbicides and solvents [15,16,17]. Some possible routes, through which LA can be transformed are summarized in Scheme 4. Figure 2 upshots the applications of LA and its derivatives.
Gamma-valerolactone (GVL) has been predicted as a major sustainable liquid which could be useful in the production of renewable hydrocarbon, which is applied to both homogeneous and heterogeneous catalytic systems. GVL, which is a major precursor in a biorefinery, can be obtained from LA through selective transformation. Gamma-valerolactone (GVL) can be synthesized from LA by two main methods. The first involves the hydrogenation of LA or its esters to gamma-hydroxyvaleric acid (or ester), which through intramolecular esterification yields GVL as the product[19,20]. Another route through which GVL can be obtained from LA is the catalytic hydrogenation through 4-hydroxyvaleric acid (4-HVA) as shown in Scheme 5. GVL, which is a platform molecule with sustainable qualities, suitable for the synthesis of fuel, production of solvent, and find useful application as a feedstock in alkenes and transportation fuels production [21,22,23].
The reduction which is carried out in a heterogeneous environment leading to the formation of GVL is usually selective; it occurs in an interesting manner as yield ranges from good to excellent status [24]. A complete conversion of LA with > 97% GVL selectivity, using 5% Ru/SiO2 or Ru/C catalyst has been reported [25]. A ruthenium-based homogeneous catalytic system where formic acid of molecular hydrogen was applied as the hydrogen source have shown a high level of activity [26,27,28]. Joó et al. recorded the first application of water-soluble homogeneous HRuCl(TPPMS)3 for the reduction of the oxo- and keto- acids several years ago before other reports [29]. Though, the turnover frequency obtained (TOF = 13 h-1) at pH = 1, depicts a very low catalytic activity. According to reports, GVL can be obtained from LA with Ru/TPPTS catalyst in an aqueous solution with 95% good yield of GVL [30]. The application of TPPTS modified Ru catalyst in biphasic hydrogenation of LA in CH2Cl2/H2O was reported by Heeres et al., an excellent yield was achieved under a relatively mild condition [31]. The activity of Ruthenium catalyst can be step up by using better electron-donating phosphine ligands such as PBu3. Ru/1, 1, 1-tris-(diphenylphoshinomethyl) ethane (Ru/TriPhos), chlorine free active catalysts have been confirmed for the reduction of LA to give GVL, 1, 4-pentanediol, and methyl-tetrahydrofurans (MTHF) [32,33]. For environmental conservation purpose, it is necessary to note that oxygenates, like 2-Metetrahydrofuran easily lead to the formation of peroxide. The fact that peroxides are threats to the environment should be put into consideration [34]. Despite the beneficial applications of alkylphosphines in transition metal catalysis, in terms of high activity and stability, they have shortcomings in their toxicity, unpleasant smell, and are usually oxygen and water sensitive. Introduction of ionic moieties such as—SO3Na into ligand structure can lower their vapor pressure, and as well accelerate their solubility and stability in polar solutions. Tukacs et al. investigated the electronic and steric characterization of novel electron-donating sulfonated phosphines, and also their application in the Ru catalyzed reduction of LA including its IR and NMR studies. In their work, they claimed that γ-valerolactone could be derived from the levulinic acid gotten from biomass, via reduction, in the presence of a catalyst. It was reported that the catalyst was generated from Ru(Acac)3 and various sulfonated phosphine RnP(C6H4 -m-SO3Na)3-n (n = 1, 2; R = Me, Pr, iPr, nBu, Cp) ligands in a reaction environment devoid of solvent and promoters. The introduction of a sulfonato group into ligand structure does not significantly affect the electronic and steric properties, this was established through the characterization of sulfonated and non-sulfonated ligands. It was confirmed that the activity of electron-donating sulfonated phosphine modified catalysts hugely surpassed the activity of Ru/TPPTS system attaining turnover number up to 6370 and maintained this activity after complete transformation of LA to GVL. They claimed that there was no sign of formation of by-product during the conversion in the IR and NMR studies. The catalyst recovery was successfully demonstrated. The volatile compound was removed at the end of the process through a vacuum, while the catalyst compounds left behind was dissolved in LA, and this showed similar activities like before distillation. A second method is acid-catalyzed dehydration of levulinic acid (LA) to angelica lactone, followed by hydrogenation (Scheme 6), though, GVL yield is lower since acidic media promote coke formation and angelica lactone polymerization [35]. Scheme 7 summarizes the pathways of LA hydrogenation to GVL. (1) hydrogenation followed by dehydration; (2) dehydration followed by hydrogenation.
A recent study by Piskun et al. shows the presence of HPA during the hydrogenation reaction and not angelica lactone, which would also suggest the dominance of pathway 1 [36]. However, there is no absolute consensus, with recent studies claiming that the reaction proceeds via pathway 2 [37,38]. γ-Valerolactone (GVL) is suitable as a promising solvent considering its non-toxic nature and as well find a useful application for the synthesis of a fuel additive or fuel precursor as a result of its functionality or reactivity characteristic feature [39]. Putting its sustainability and renewability to keen consideration, GVL is suitable to provide new avenues where petroleum-based products and fuels could be substituted with biomass-based resources. Alonso DM et al. in their critical review highlighted four major key opportunities for the synthesis of GVL from biomass-derived sources. As described in their work the opportunities we set to derive from production of GVL biomass are as follows: (1) Purification of LA from mineral acids. The issues raised by the use of mineral acids could be addressed by replacing them with heterogeneous catalysts for the production of levulinic acid (LA). This is considered as the most suitable approach means for solving the mineral acids related issues; (2) Choosing stable catalysts in the presence of mineral acids. Recently remarkable development has been recorded as far as this aspect is concerned, using real biomass derived feed is necessary for catalyst stability; (3) Alternative catalysts to noble metal catalysts. The usage of non-precious metal catalysts or solid acid catalysts in the recent work with GVL as a solvent could as well open way for non-precious metals to be used in the hydrogenation process of LA. This would allow the overall system to be more economically feasible; (4) Techno economic analyses of different pathways will be important factor to address the relative challenges attached (e.g., product yields, solvent selection, catalyst metal, etc.) in these GVL processes [39]. Using homogeneous catalyst in the synthesis of levulinic acid poses a major bottleneck of isolation and separation of the product from the aqueous phase containing the mineral acids. In view of this organic solvent extraction is needed for the isolation of LA from the product stream, for instance methyl isobutyl ketone (MIBK) could be a suitable candidate in this regard [40]. The properties of the GVL make it an excellent solvent as it is able to solubilize lignocellulosic biomass and achieve high yields of LA using solid catalysts. Dumesic et al. in their recent works give interesting account of GVL, where novel options to valorize biomass in GVL/water/H2SO4 medium were highlighted as follows:
Synthesis of monomeric carbohydrates using corn stover fractionation in a ternary mixture of GVL, water and another organic solvent, (benzene, toluene, phenol, etc.) [41].
Synthesis of maleic acid via oxidation of furfural synthesized from corncobs fractionation in aqueous GVL solution [42].
High-yield production of furfural by dehydration of xylose obtained from maple wood fractionation in aqueous GVL solution [43].
Recently a lot of researchers have focused their attention on the continuous-flow upgrading of levulinic acid to GVL. Considering the recent research work carried out by Moreno-Marrodan and Barbaro where a sulfonated resin embedded with Ru nanoparticles was developed as a bifunctional heterogeneous catalyst for the hydrogen-mediated reduction of levulinic acid (LA) to GVL. About 90–95% excellent conversion and quantitative selectivity were recorded at mild reaction conditions; 70 ℃ reaction temperature and 5 bar pressure with feedstock of levulinic acid and a stream of H2 to the reactor. The activity of catalyst was intact for the period of 32 h reaction operation time [44]. Palladium and silver nanoparticles supported on a graphitic carbon nitride surface was employed by Tadele et al. in their recent work for the production of GVL from levulinic acid (LA) where formic acid was adopted as the H donor. With the reaction conditions of 50 min and 70 ℃ reaction time and temperature respectively the catalyst in suspension nature was pumped in a feedstock solution of levulinic acid (LA) and formic acid leading to formation of a quantitative yield of GVL in a coil reactor [45]. Gerardy et al. recently reported a cascade process where a single bifunctional Ni-K/HZSM-5 catalyst was applied for the conversion of levulinic acid (LA) directly into a mixture of valeric acid and ethyl valerate. In their work a three-step process for the production of valeric biofuels from levulinic acid was reported including hydrogenation to γ-valerolactone (GVL), hydrogenation of GVL to valeric acid, and a final esterification to give alkyl valerates [46].
2-Butanol and 2-pentanol are monohydric alcohols that can be derived from LA in the pilot scale production. Levulinic acid (LA), an important platform chemical gotten from lignocellulosic biomass, has the potentials of being transformed to other value-added chemicals. 2-BO/2-PO are formed by the removal of the extra oxygen atoms present in the LA molecule. 2-BO/2-PO are tenable to transformation into fuel, fuel additives and solvents [47,48,49]. Scheme 8 shows the overall reaction that leads LA to the production of 2-BO and 2-PO.
Step-a: LA is firstly hydrogenated, then subjected to cyclization to form γ-valerolactone (GVL) [50,51].
Step-b, c: GVL is transformed into 1, 4-pentadiol (PDO) and 2-Methyltetrahydrofuran (MTHF) via a ring opening and dehydration reactions, respectively. PDO and MTHF can be reconverted through dehydration or hydrolysis reactions [52,53].
Step-d, e: A two parallel pathways is unleashed on PDO to cause a C-C bond scission, leading to the formation of 2-BO, and C-O bond scission to produce 2-PO. 2-PO is formed on the hydrogenation of MTHF [54,55]. Due to the multiple role that the catalyst employed in LA transformation ought to play, its highly selective, considering the multiple steps involved in lactone activation, ether, and C-C/C-O bonds. The catalyst directive role on the reaction thus expands the selectivity of PDO, 2-BO, and 2-PO. Cao et al. reported the transformation of GVL to PDO at 200 ℃ and 6 MPa initial pressure, using Cu/ZrO2 as a catalyst, in their work. Kaneda worked with Pt-Mo catalyst, this increased the selectivity of LA hydrogenation with 93% yield of PDO under a milder situation of 130 ℃ and 3 MPa, in comparison with the earlier work by Cao et al. [56]. Palkovits et al. reported the hydrogenation of LA using Ru/C catalyst, it gave a 75% total yield, the best yield so far recorded for 2-BO & 2-PO with such catalyst. Though the process was carried out in a severe condition of 190 ℃ and 10 MPa H2 pressure; hence a general limitation on the process [57]. A yield of 57% of 2-BO & 2-PO was obtained by Arias, using Ru/C catalyst for the transformation of MTHF (an LA hydrogenation intermediate) at a temperature of 250 ℃ and 4 MPa initial pressure [58]. Rh-MoOx/SiO2 catalyst was employed by Li et al., in the hydrogenation of LA at 80 ℃ and 6 MPa (a mild condition), yet the achieved selectivity of 1-pentanol and 2- PO was only 15.8% [59]. We can conclude from the results obtained from previous researches, that elevated temperature and high H2 pressure are indispensable conditions for these supported metal catalysts in other to promote 2-BO & 2-PO yields.
Lircus et al. in their recent work, reported on the use of nanoporous metals for the hydrogenation of LA, nanoporous ruthenium (NP-Ru) displayed good catalytic performance for the synthesis of alcohols. The overall yield of 2-butanol and 2-pentanol was 78.8%, and the yield of 1, 4-pentadiol was recorded to be 74.6%, these were obtained at a reaction temperature of 140 ℃ and 100 ℃ respectively, where water is the solvent [60]. Nanoporous metals are phenomena materials which have high surface-area to volume ratio, and a large proportion of low coordinated surface-active sites. These metals have exhibited excellent catalytic performance in hydrogenation, dehydrogenation and dehydration reactions, even under very mild conditions [61]. They initially studied several Ru-based catalysts using the same conditions for the hydrogenation of LA. 100% conversion of LA was obviously reported using these catalysts, though a different selectivity was observed. γ-valerolactone is the main product derived with powder Ru, Ru/C, and Ru/Al2O3 catalyst; it was still 2-BO & 2-PO. The disparity shows that NP-Ru possess more affinity for the lactone group of GVL, and hence activates better than other Ru catalysts. Several other nanoporous metals (e.g., Ni, Fe, Co, Cu) catalysts were investigated, in order to verify the major desired product(s) formed during the hydrogenation of LA under same conditions, GVL was still the major product obtained, with little amount of 2-BO and 2-PO followed by observation of an accompanied leaching of catalyst [62,63]. Velisoju et al. in their recent work gave account of single step production of pentanoic acid (PA) from levulinic acid (LA) employed Ni-W/SiO2, Ni-W/Al2O3, Ni/HZSM-5 and Ni-W/H-ZSM-5 catalysts at 270 ℃ reaction temperature. Among the investigated catalysts Ni-W/H-ZSM-5 demonstrated high levulinic acid (LA) conversion and pentanoic acid (PA) selectivity compared to other catalysts. This higher PA formation rate over Ni-W/H-ZSM-5 was attributed to high proportion of Brønsted acid sites available on Ni-W/H-ZSM-5 surface compared to other supports. In this report they claimed that the major role of Brønsted acid sites in levulinic acid (LA) conversion to pentanoic acid (PA) was to initiate subsequent conversion of the intermediate GVL [62].
Levulinic acid is one of the important compounds that are easily derived from cellulose; it is useful platform chemical in the chemical industry, a significant progress has been made in its production from cellulose-containing biomass [63,64]. A continuous process that converts biomass into LA has been developed by Biofine Renewables, LLC. This implies that new reaction schemes that will improve the industrial consumption of levulinic acid as raw material should be developed [65,66,67].
The presence of the carbonyl group in the molecular structure of levulinic acid provides different possible reaction pathways to levulinic acid transformation. Several high valued compounds are achievable via Baeyer-Villiger (BV) oxidation of levulinic acid, as shown in Scheme 9. The BV oxidation is generally a two-step reaction, during which a peroxide is added to a C = O group through a nucleophilic attack to form a Criegee intermediate, this is succeeded by the migration of H or alkyl group that is vicinal to the C = O group and the insertion of an oxygen atom into the C-C bond or C-H bond. As displayed in Scheme 9, the insertion of oxygen between the δ-carbon and the γ-carbon is followed by hydrolysis, which gives succinic acid and methanol. In another instance, if the insertion occurs between the γ-carbon and the β-carbon, 3-acetoxypropanoic acid is produced [68]. 3-acetoxypropanoic acid is readily hydrolyzed into acetic acid and 3-hydroxypropanoic acid. The U.S. Department of Energy listed both succinic acid and 3-hydroxypropanoic acid among the top 12 platform molecules, as shown in Scheme 1. Succinic acid is employed in the production of biodegradable polymers, food additives, pharmaceuticals, and detergents, etc. It is also a starting material in the synthesis of 1, 4-butanediol, gamma-butyrolactones, and tetrahydrofuran, etc. Likewise, 3-hydroxypropanoic acid is a potential monomer for the synthesis of biodegradable polymers. Acrylic acid and its derivatives could be derived from the dehydration of 3-hydroxypropanoic acid; this has contributed to its expanded market. It has also been utilized in the synthesis of other useful chemicals, like 1, 3-propanediol [13,69]. But the available technologies applied in their production mainly depend on biological pathways, so, suffer from usual limitations that affect biological production, such as difficult pH control, difficulty in the separation of constituents (e.g., products from fermentation broth), deactivation of enzymes, etc. [70,71,72,73,74]. Hence, the chemocatalytic conversion is a better substitute to achieve these important chemicals. There are two folds of challenges for BV oxidation of levulinic acid; the first is the difficulty in designing an active catalytic system for the oxidation, since LA is a linear ketone with a carboxylic group, and its generally more challenging to convert linear ketones using BV oxidation, this is partly due to the absence of ring strain [75]. Secondly, there is the possibility of insertion of oxygen on either side of the carbonyl (C = O) group, hence, to direct the location of insertion is very necessary and reaction selectivity must be instated. Fermentation is a synthetic pathway from edible biomass (sugar) to succinic acid that has recently been commercialized [76]. Though the use of non-edible lignocellulosic biomass as raw material for valuable chemicals would be better required and preferable, and to secure low cost and sustainability [77,78]. A simple treatment of lignocellulose with Brønsted/Lewis acid catalyst in water or methanol will efficiently result in formation of levulinic acid or its methyl ester in a single step [79,80,81]. Hence, a simple, direct chemical transformation from levulinic acid to succinic acid is needed, because succinic acid is an important four-carbon feedstock applicable for conversions to a number of value-added products, like 1, 4-butanediol, γ-butyrolactone, and 2-pyrrolidone, it also functions as a raw material in the synthesis of bio-based polymers and green, sustainable plastics [82,83,84,85,86]. Hence, a direct and feasible chemical method for the conversion of non-edible lignocellulose to succinic acid through levulinic acid is really desired to achieve a real industrial application. However, the existing chemical methods available for the conversion of levulinic acid to succinic acid involves critical multiple step synthetic route [87,88]. The method also required corrosive reaction conditions and toxic heavy metals in the process and a poor yield is achieved on the course of reaction [89,90,91]. For instance, vanadium catalyst is needed for the gas-phase oxidation of levulinic acid, an appreciable yield of succinic acid is achieved with high temperature (375 ℃) [92]. Silica-coated magnetic nanoparticle-supported Ru(Ⅲ) catalyst when applied in the oxidation at a lower temperature (150 ℃), however, a pressure of 10bar of O2 is demanded. A more convenient method was reported by the use of aq. 30% H2O2 in acidic media, which was based on the application of an unusual terminal Baeyer-Villiger oxidation (BVO) of levulinic acid to produce succinic acid, a net yield of 62% was achieved [93,94,95]. A large amount of acetic acid and 3-hydroxypropionic acid are formed simultaneously through normal BVO (ca. 6:4 selectivity). This shows that the development of a kinetically controlled conversion of levulinic acid to succinic acid under a mild reaction condition is yet needed. Figure 3 shows a recent method reported by Kawasumi et al., it directly converts levulinic to succinic acid at room temperature with high yield. It involved the customization of haloform reaction to enable a one-step, regioselective oxidative demethylation of levulinic acid under a very mild condition. There was a successful investigation of the procedure of the process on the range of methyl ketones and secondary ethanol derivatives for verification [88]. Kong, X. et. al., reported the silicotungstic acid supported on commercially silica-gel sphere supports as catalyst for the esterification of levulinic acid for the first time by modification as alternative inorganic acid for the production of ethyl levulinate (EL). The synthesis was carried out with ethanol and ethyl levulinate (EL) yield obtained was 97% with 20% silicotungstic acid [96].
A numerous number of research activities is currently embarked on globally exploring potential chemical transformations of biomass into value-added chemicals and fuels. Levulinic acid (LA) is a very useful building block for the production of chemicals which find useful applications like in the synthesis of additives, polymer and as a resin precursor. LA is still a promising substrate not only for biocomponents production but also for other chemicals. In the course of searching for new LA sources, wasted parts of raw materials used in food, agricultural, wood industry, etc. should be considered for full exploration in order to alleviate environmental degradation experienced due to the usage of petroleum-based products, and to make the production cost effective. This action would not only allow us to find new LA sources but also could upgrade the value of some waste and could enable new, effective utilization, bringing us more advantages. Increasing energy, fuel, chemicals demand causes the need to turn attention to renewable, unconventional, alternative raw materials, which include biomass. The biorefinery sector, who are currently receiving full support from the European Union is boosting the research strength, as this can be seen in sustainable and bio-based research programs based on the pieces of evidence around the globe. New biomass sources which are cheap, easy in processing and have the ability for rapid renewal at any time are desired to achieve sustainable development. Looking for alternative fuel and energy sources comes down to searching for the new production methods and technological solutions and to the upgrading of existing ones for process efficiency, selectivity and economy increase, with the least possible negative impact on the environment in terms of harmful substances emission. Taking this type of actions is very important because coal, crude oil, and natural gas, are depleting resources and the existence of biomass waste, which lay on landfills and their potential is not used. Further work should be embarked on to improve catalysts and catalytic systems for the synthesis of levulinic acid (LA), to develop environmentally friendly catalysts in line with principles of 'Green Chemistry' in order to salvage our environment from present environmental degradation.
Discussion with Professor Barminas Jeffery of the Department of Chemistry, Modibbo Adama University of Technology is inspiring and challenging which has recently challenged the process through a selfless and corrosive contribution leading to the success of this review paper. It is highly appreciated.
All authors declare no competing conflicts of interest.
[1] | Adeleye AT, Louis H, Temitope HA, et al. (2019). Ionic liquids (ILs): advances in biorefinery for the efficient conversion of lignocellulosic biomass. Asian J Green Chem 3: 391–417. |
[2] |
Tilman D, Hill J, Lehman C (2006). Carbon-Negative biofuels from Low-Input High-Diversity grassland biomass. Science 314: 1598–1600. doi: 10.1126/science.1133306
![]() |
[3] | International Energy Outlook 2016 with Projections to 2040. A report by U.S. Energy Information Administration (EIA), 2016. Available from: https://www.eia.gov/outlooks/ieo/pdf/0484(2016). |
[4] |
Mohanty AK, Misra M, Drzal LT (2002) Sustainable Bio-Composites from renewable resources: opportunities and challenges in the green materials world. J Polym Environ 10: 19–26. doi: 10.1023/A:1021013921916
![]() |
[5] | Belkacemi K, Kemache N, Hamoudi S, et al. (2007) Hydrogenation of sunflower oil over bimetallic supported catalysts on mesostructured silica material. Int J Chem React Eng 5: 1–28. |
[6] |
Makshina EV, Dusselier M, Janssens W, et al. (2014) Review of old chemistry and new catalytic advances in the on-purpose synthesis of butadiene. Chem Soc Rev 43: 7917–7953. doi: 10.1039/C4CS00105B
![]() |
[7] | Bridgwater A (2013) Fast pyrolysis of biomass for the production of liquids for use as fuels and chemicals. Biomass Combust Sci, Technol Eng 7: 130–171. |
[8] | Top value added chemicals from biomass. Volume I-Results of screening for potential candidates from sugars and synthesis gas (2004). Available from: https://www.nrel.gov/docs/fy04osti/35523.pdf. |
[9] |
Jow J, Rorrer GL, Hawley MC, et al. (1987) Dehydration of d-fructose to levulinic acid over LZY zeolite catalyst. Biomass 14: 185–194. doi: 10.1016/0144-4565(87)90046-1
![]() |
[10] |
Serrano-Ruiz JC, West RM, Dumesic JA (2010) Catalytic conversion of renewable biomass resources to fuels and chemicals. Annu Rev Chem Biomol Eng 1: 79–100. doi: 10.1146/annurev-chembioeng-073009-100935
![]() |
[11] |
Andersson-Engels S, Berg R, Svanberg K, et al. (1995) Multi‐colour fluorescence imaging in connection with photodynamic therapy of δ‐amino levulinic acid (ALA) sensitised skin malignancies. Bioimaging 3: 134–143. doi: 10.1002/1361-6374(199509)3:3<134::AID-BIO4>3.3.CO;2-T
![]() |
[12] |
Zhang J, Wu S, Li B, et al. (2012) Advances in the catalytic production of valuable Levulinic Acid derivatives. ChemCatChem 4: 1230–1237. doi: 10.1002/cctc.201200113
![]() |
[13] |
Pasquale G, Vázquez P, Romanelli G, et al. (2012) Catalytic upgrading of levulinic acid to ethyl levulinate using reusable silica-included Wells-Dawson heteropolyacid as catalyst. Catal Commun 18: 115–120. doi: 10.1016/j.catcom.2011.12.004
![]() |
[14] |
Huber GW, Iborra S, Corma A (2006) Synthesis of transportation fuels from biomass: Chemistry, catalysts, and engineering. Chem Rev 106: 4044–4098. doi: 10.1021/cr068360d
![]() |
[15] |
Tiong YW, Yap CL, Gan S, et al. (2018) Conversion of biomass and its derivatives to levulinic acid and levulinate esters via ionic liquids. Ind Eng Chem Res 57: 4749–4766. doi: 10.1021/acs.iecr.8b00273
![]() |
[16] |
Yu HT, Chen BY, Li BY, et al. (2018) Efficient pretreatment of lignocellulosic biomass with high recovery of solid lignin and fermentable sugars using Fenton reaction in a mixed solvent. Biotechnol Biofuels 11: 287. doi: 10.1186/s13068-018-1288-4
![]() |
[17] |
Rackemann DW, Doherty WO (2011) The conversion of lignocellulosics to levulinic acid. Biofuels, Bioprod Biorefin 5: 198–214. doi: 10.1002/bbb.267
![]() |
[18] | Mthembu LD (2016) Production of levulinic acid from sugarcane bagasse. Available from: https://openscholar.dut.ac.za/handle/10321/1713.pdf. |
[19] | Morrison RT, Boyd RN (1983) "Organic Chemistry", 4th Eds., Allyn and Bacon, Inc. Boston, 338. |
[20] |
Chalid M, Heeres HJ, Broekhuis AA (2012) Green polymer precursors from Biomass-Based levulinic acid. Procedia Chem 4: 260–267. doi: 10.1016/j.proche.2012.06.036
![]() |
[21] |
Horváth IT, Mehdi H, Fábos V, et al. (2008) γ-Valerolactone-a sustainable liquid for energy and carbon-based chemicals. Green Chem 10: 238–242. doi: 10.1039/B712863K
![]() |
[22] |
Fegyverneki D, Orha L, Láng G, et al. (2010) Gamma-valerolactone-based solvents. Tetrahedron 66: 1078–1081. doi: 10.1016/j.tet.2009.11.013
![]() |
[23] | Van der Waal JC, de Jong E (2016) Avantium Chemicals: The high potential for the levulinic product tree. Ind Biorenewables 4: 97–120. |
[24] |
Su K, Li Z, Cheng B, et al. (2010) Studies on the carboxymethylation and methylation of bisphenol A with dimethyl carbonate over TiO2/SBA-15. J Mol Catal A: Chem 315: 60–68. doi: 10.1016/j.molcata.2009.08.027
![]() |
[25] |
Yan Z, Lin L, Liu S (2009) Synthesis of γ-Valerolactone by hydrogenation of biomass-derived levulinic acid over Ru/C Catalyst. Energy Fuels 23: 3853–3858. doi: 10.1021/ef900259h
![]() |
[26] |
Tukacs JM, Király D, Strádi A, et al. (2012) Efficient catalytic hydrogenation of levulinic acid: a key step in biomass conversion. Green Chem 14: 2057–2067. doi: 10.1039/c2gc35503e
![]() |
[27] |
Ruppert AM, Jędrzejczyk M, Sneka-Płatek O, et al. (2016) Ru catalysts for levulinic acid hydrogenation with formic acid as a hydrogen source. Green Chem 18: 2014–2028. doi: 10.1039/C5GC02200B
![]() |
[28] |
Jones DR, Iqbal S, Miedziak PJ, et al. (2018) Selective hydrogenation of levulinic acid using Ru/C catalysts prepared by Sol-Immobilisation. Top Catal 61: 833–843. doi: 10.1007/s11244-018-0927-0
![]() |
[29] |
Joó F, Beck MT (1975) Formation and catalytic properties of water-soluble phosphine complexes. React Kinet Catal Lett 2: 257–263. doi: 10.1007/BF02068199
![]() |
[30] |
Mehdi H, Fábos V, Tuba R, et al. (2008) Integration of homogeneous and heterogeneous catalytic processes for a Multi-step conversion of biomass: From sucrose to levulinic acid, γ-Valerolactone, 1,4-Pentanediol, 2-Methyl-tetrahydrofuran, and alkanes. Top Catal 48: 49–54. doi: 10.1007/s11244-008-9047-6
![]() |
[31] |
Mallat T, Baiker A (2004) Oxidation of alcohols with molecular oxygen on solid catalysts. Chem Rev 104: 3037–3058. doi: 10.1021/cr0200116
![]() |
[32] |
Werkmeister S, Junge K, Beller M (2014) Catalytic hydrogenation of carboxylic acid esters, amides, and nitriles with homogeneous catalysts. Org Process Res Dev 18: 289–302. doi: 10.1021/op4003278
![]() |
[33] |
Geilen FMA, Engendahl B, Hölscher M, et al. (2011) Selective homogeneous hydrogenation of biogenic carboxylic acids with [Ru(TriPhos)H]+: A mechanistic study. J Am Chem Soc 133: 14349–14358. doi: 10.1021/ja2034377
![]() |
[34] |
Fábos V, Koczó G, Mehdi H, et al. (2009) Bio-oxygenates and the peroxide number: a safety issue alert. Energy Environ Sci 2: 767–770. doi: 10.1039/b900229b
![]() |
[35] |
Feng J, Gu X, Xue Y, et al. (2018) Production of γ-valerolactone from levulinic acid over a Ru/C catalyst using formic acid as the sole hydrogen source. Sci Total Environ 633: 426–432. doi: 10.1016/j.scitotenv.2018.03.209
![]() |
[36] |
Piskun AS, de Haan JE, Wilbers E, et al. (2016) Hydrogenation of levulinic acid to γ-Valerolactone in water using millimeter sized supported Ru catalysts in a packed bed reactor. ACS Sustainable Chem Eng 4: 2939–2950. doi: 10.1021/acssuschemeng.5b00774
![]() |
[37] | Balla P, Perupogu V, Vanama PK, et al. (2015) Hydrogenation of biomass-derived levulinic acid to γ-valerolactone over copper catalysts supported on ZrO2. J Chem Technol Biotechnol 91: 769–776. |
[38] |
Mohan V, Raghavendra C, Pramod CV, et al. (2014) Ni/H-ZSM-5 as a promising catalyst for vapour phase hydrogenation of levulinic acid at atmospheric pressure. RSC Adv 4: 9660–9669. doi: 10.1039/c3ra46485g
![]() |
[39] |
Alonso DM, Wettstein SG, Dumesic JA, et al. (2013) Gamma-valerolactone, a sustainable platform molecule derived from lignocellulosic biomass. Green Chem 15: 584–595. doi: 10.1039/c3gc37065h
![]() |
[40] | Girisuta B, Heeres HJ (2017) Levulinic acid from biomass: Synthesis and applications. In: Biofuels and Biorefineries, 143–169. |
[41] |
Motagamwala AH, Won W, Dumesic JA, et al. (2016) An engineered solvent system for sugar production from lignocellulosic biomass using biomass derived gammavalerolactone. Green Chem 18: 5756–5763. doi: 10.1039/C6GC02297A
![]() |
[42] |
Rodenas Olaya Y, Mariscal R, Fierro JLG, et al. (2018) Granados, Improving the production of maleic acid from biomass: TS-1 catalysed aqueous phase oxidation of furfural in the presence of γ-valerolactone. Green Chem 20: 2845–2856. doi: 10.1039/C8GC00857D
![]() |
[43] |
Canan S, Hussain MA, Martin AD, et al. (2018) Enhanced furfural yields from xylose dehydration in the γ-Valerolactone/Water solvent system at elevated temperatures. ChemSusChem 11: 2321–2331. doi: 10.1002/cssc.201800730
![]() |
[44] |
Moreno-Marrodan C, Barbaro P (2014) Energy efficient continuous production of γ-valerolactone by bifunctional metal/acid catalysis in one pot. Green Chem 16: 3434–3438. doi: 10.1039/c4gc00298a
![]() |
[45] |
Tadele K, Verma S, Gonzalez MA, et al. (2017) A sustainable approach to empower the bio-based future: upgrading of biomass via process intensification. Green Chem 19: 1624–1627. doi: 10.1039/C6GC03568J
![]() |
[46] | Gerardy R, Morodo R, Estager J, et al. (2018) Sustaining the Transition from a petrobased to a Biobased Chemical Industry with Flow Chemistry. Top Curr Chem 377: 1–35. |
[47] |
Bozell JJ, Petersen GR (2010) Technology development for the production of biobased products from biorefinery carbohydrates-the US Department of Energy's 'Top 10' revisited. Green Chem 12: 539–556. doi: 10.1039/b922014c
![]() |
[48] |
Fortman JL, Chhabra S, Mukhopadhyay A, et al. (2008) Biofuel alternatives to ethanol: pumping the microbial well. Trends Biotechnol 26: 375–381. doi: 10.1016/j.tibtech.2008.03.008
![]() |
[49] |
Serrano-Ruiz JC, Dumesic JA (2009) Catalytic upgrading of lactic acid to fuels and chemicals by dehydration/hydrogenation and C–C coupling reactions. Green Chem 11: 1101–1106. doi: 10.1039/b906869d
![]() |
[50] |
Abdelrahman OA, Heyden A, Bond JQ (2014) Analysis of kinetics and reaction pathways in the Aqueous-Phase hydrogenation of levulinic acid to form γ-Valerolactone over Ru/C. ACS Catal 4: 1171–1181. doi: 10.1021/cs401177p
![]() |
[51] |
Prati L, Jouve A, Villa A (2017) Production and upgrading of γ-Valerolactone with bifunctional catalytic processes. Biofuels Biorefin 8: 221–237. doi: 10.1007/978-981-10-5137-1_7
![]() |
[52] |
Patankar SC, Yadav GD (2015) Cascade engineered synthesis of γ-Valerolactone, 1,4-Pentanediol, and 2-Methyltetrahydrofuran from levulinic acid using Pd–Cu/ZrO2Catalyst in water as solvent. ACS Sustainable Chem Eng 3: 2619–2630. doi: 10.1021/acssuschemeng.5b00763
![]() |
[53] |
Obregón I, Gandarias I, Miletić N, et al. (2015) One-Pot 2-Methyltetrahydrofuran production from levulinic acid in green solvents using Ni-Cu/Al2O3 catalysts. ChemSusChem 8: 3483–3488. doi: 10.1002/cssc.201500671
![]() |
[54] |
Phanopoulos A, White AJP, Long NJ, et al. (2015) Catalytic transformation of levulinic acid to 2-Methyltetrahydrofuran using ruthenium–N-Triphos complexes. ACS Catal 5: 2500–2512. doi: 10.1021/cs502025t
![]() |
[55] |
De Lima AEP, de Oliveira DC (2017) In situ XANES study of cobalt in Co-Ce-Al catalyst applied to steam reforming of ethanol reaction. Catal Today 283: 104–109. doi: 10.1016/j.cattod.2016.02.029
![]() |
[56] |
Du XL, Bi QY, Liu YM, et al. (2012) Tunable copper-catalyzed chemoselective hydrogenolysis of biomass-derived γ-valerolactone into 1,4-pentanediol or 2-methyltetrahydrofuran. Green Chem 14: 935–939. doi: 10.1039/c2gc16599f
![]() |
[57] |
Al-Shaal MG, Dzierbinski A, Palkovits R (2014) Solvent-free γ-valerolactone hydrogenation to 2-methyltetrahydrofuran catalysed by Ru/C: a reaction network analysis. Green Chem 16: 1358–1364. doi: 10.1039/C3GC41803K
![]() |
[58] |
Delidovich I, Palkovits R (2016) Catalytic isomerization of Biomass-Derived aldoses: A Review. ChemSusChem 9: 547–561. doi: 10.1002/cssc.201501577
![]() |
[59] |
Mizugaki T, Nagatsu Y, Togo K, et al. (2015) Selective hydrogenation of levulinic acid to 1,4-pentanediol in water using a hydroxyapatite-supported Pt–Mo bimetallic catalyst. Green Chem 17: 5136–5139. doi: 10.1039/C5GC01878A
![]() |
[60] |
Licursi D, Antonetti C, Fulignati S, et al. (2018) Cascade strategy for the tunable catalytic valorization of levulinic acid and γ-Valerolactone to 2-Methyltetrahydrofuran and alcohols. Catalysts 8: 277–293. doi: 10.3390/catal8070277
![]() |
[61] | Wittstock A, Bäumer M (2013) Catalysis by unsupported skeletal gold catalysts. Acc Chem Res 47: 731–739. |
[62] |
Velisoju VK, Gutta N, Tardio J, et al. (2018) Hydrodeoxygenation activity of W modified Ni/H-ZSM-5 catalyst for single step conversion of levulinic acid to pentanoic acid: An insight on the reaction mechanism and structure activity relationship. Appl Catal A: Gen 550: 142–150. doi: 10.1016/j.apcata.2017.11.008
![]() |
[63] | Isikgor FH, Becer CR (2015) Lignocellulosic biomass: a sustainable platform for the production of bio-based chemicals and polymers. Polym Chem 6: 4497–4559. |
[64] |
Di Mondo D, Ashok D, Waldie F, et al. (2011) Stainless steel as a catalyst for the total deoxygenation of glycerol and levulinic acid in aqueous acidic medium. ACS Catal 1: 355–364. doi: 10.1021/cs200053h
![]() |
[65] |
Lin H, Strull J, Liu Y, et al. (2012) High yield production of levulinic acid by catalytic partial oxidation of cellulose in aqueous media. Energy Environ Sci 5: 9773–9781. doi: 10.1039/c2ee23225a
![]() |
[66] |
Weingarten R, Conner WC, Huber GW (2012) Production of levulinic acid from cellulose by hydrothermal decomposition combined with aqueous phase dehydration with a solid acid catalyst. Energy Environ Sci 5: 7559–7571. doi: 10.1039/c2ee21593d
![]() |
[67] |
Wettstein SG, Alonso DM, Chong Y, et al. (2012) Production of levulinic acid and gamma-valerolactone (GVL) from cellulose using GVL as a solvent in biphasic systems. Energy Environ Sci 5: 8199–8204. doi: 10.1039/c2ee22111j
![]() |
[68] |
Dutta S, Wu L, Mascal M (2015) Efficient, metal-free production of succinic acid by oxidation of biomass-derived levulinic acid with hydrogen peroxide. Green Chem 17: 2335–2338. doi: 10.1039/C5GC00098J
![]() |
[69] |
Zhang D, Hillmyer MA, Tolman WB (2004) A new synthetic route to Poly[3-hydroxypropionic acid] (P[3-HP]): Ring-Opening polymerization of 3-HP macrocyclic esters. Macromolecules 37: 8198–8200. doi: 10.1021/ma048092q
![]() |
[70] |
Song H, Lee SY (2006) Production of succinic acid by bacterial fermentation. Enzyme Microb Technol 39: 352–361. doi: 10.1016/j.enzmictec.2005.11.043
![]() |
[71] |
Beauprez JJ, De Mey M, Soetaert WK, et al. (2010) Microbial succinic acid production: natural versus metabolic engineered producers. Process Biochem 45: 1103–1114. doi: 10.1016/j.procbio.2010.03.035
![]() |
[72] |
Cao Y, Cao Y, Lin X (2011) Metabolicallyengineered escherichia coli forbiotechnological production offour-carbon 1,4-dicarboxylic acids. J Ind Microbiol Biotechnol 38: 649–656. doi: 10.1007/s10295-010-0913-4
![]() |
[73] |
Kumar V, Ashok S, Park S (2013) Recent advances in biological production of 3-hydroxypropionic acid. Biotechnol Adv 31: 945–961.. doi: 10.1016/j.biotechadv.2013.02.008
![]() |
[74] |
Li J, Zheng XY, Fang XJ, et al. (2011) A complete industrial system for economical succinic acid production by Actinobacillus succinogenes. Bioresour Technol 102: 6147–6152. doi: 10.1016/j.biortech.2011.02.093
![]() |
[75] |
Ten Brink GJ, Arends IWCE, Sheldon RA (2004) The Baeyer−Villiger reaction: New developments toward greener procedures. Chem Rev 104: 4105–4124. doi: 10.1021/cr030011l
![]() |
[76] |
Cok B, Tsiropoulos I, Roes AL, et al. (2014) Succinic acid production derived from carbohydrates: An energy and greenhouse gas assessment of a platform chemical toward a bio-based economy. Biofuels, Bioprod Biorefin 8: 16–29. doi: 10.1002/bbb.1427
![]() |
[77] |
Deng W, Zhang Q, Wang Y (2014) Catalytic transformations of cellulose and cellulose derived carbohydrates into organic acids. Catal Today 234: 31–41. doi: 10.1016/j.cattod.2013.12.041
![]() |
[78] |
Kobayashi H, Fukuoka A (2013) Synthesis and utilisation of sugar compounds derived from lignocellulosic biomass. Green Chem 15: 1740–1764.. doi: 10.1039/c3gc00060e
![]() |
[79] | Nemoto K, Tominaga K, Sato K (2015) Facile and efficient transformation of lignocellulose into levulinic acid using an AlCl3.6H2O/H3PO4 hybrid acid catalyst. Bull Chem Soc Jpn 88: 1752–1754. |
[80] |
Nemoto K, Tominaga K, Sato K (2014) Straightforward synthesis of levulinic acid ester from lignocellulosic biomass resources. Chem Lett 43: 1327–1329. doi: 10.1246/cl.140382
![]() |
[81] |
Tominaga K, Mori A, Fukushima Y, et al. (2011) Mixed-acid systems for the catalytic synthesis of methyl levulinate from cellulose. Green Chem 13: 810–813. doi: 10.1039/c0gc00715c
![]() |
[82] |
Galletti AMR, Antonetti C, De Luise V, et al. (2012) A sustainable process for the production of γ-valerolactone by hydrogenation of biomass-derived levulinic acid. Green Chem 14: 688–695. doi: 10.1039/c2gc15872h
![]() |
[83] |
Joshi SS, Zodge AD, Pandare KV, et al. (2014) Efficient conversion of cellulose to levulinic acid by hydrothermal treatment using zirconium dioxide as a recyclable solid acid catalyst. Ind Eng Chem Res 53: 18796–18805. doi: 10.1021/ie5011838
![]() |
[84] |
Tuteja J, Choudhary H, Nishimura S, et al. (2014) Direct synthesis of 1,6-Hexanediol from HMF over a heterogeneous Pd/ZrP catalyst using formic acid as hydrogen source. ChemSusChem 7: 96–100. doi: 10.1002/cssc.201300832
![]() |
[85] |
McKinlay JB, Vielle C, Zeikus JG (2007) Prospects for a bio-based succinate industry. Appl Microbiol Biotechnol 76: 727–740. doi: 10.1007/s00253-007-1057-y
![]() |
[86] |
Bechthold I, Bretz K, Kabasci S, et al. (2008) Succinic Acid: A new platform chemical for biobased polymers from renewable resources. Chem Eng Technol 31: 647–654. doi: 10.1002/ceat.200800063
![]() |
[87] | Mitschka R, Oehldrich J, Takahashi K, et al. (1981) General approach for the synthesis of polyquinanes. Facile generation of molecular complexity via reaction of 1,2-dicarbonyl compounds with dimethyl 3-. Tetrahedron 37: 4521–4542. |
[88] |
Kawasumi R, Narita S, Miyamoto K, et al. (2017) One-step conversion of levulinic acid to succinic acid using I2/t-BuOK system: The iodoform reaction revisited. Scientific Reports 7: 1–8. doi: 10.1038/s41598-016-0028-x
![]() |
[89] |
Podolean I, Kuncser V, Gheorghe N, et al. (2013) Ru-based magnetic nanoparticles (MNP) for succinic acid synthesis from levulinic acid. Green Chem 15: 3077–3083. doi: 10.1039/c3gc41120f
![]() |
[90] | Pandey SK, Yadav SPS, Prasad M, et al. (1999) Mechanism of Ru(III) catalysis in oxidation of levulinic acid. Asian J Chem11: 203–206. |
[91] |
Caretto A, Perosa A (2013) Upgrading of levulinic acid with dimethylcarbonate as solvent/reagent. ACS Sustainable Chem Eng 1: 989–994. doi: 10.1021/sc400067s
![]() |
[92] |
Besson M, Gallezot P, Pinel C (2014) Conversion of biomass into chemicals over metal catalysts. Chem Rev 114: 1827–1870. doi: 10.1021/cr4002269
![]() |
[93] |
Stoute VA, Winnik MA, Csizmadia IG (1974) Theoretical model for the Baeyer-Villiger rearrangement. J Am Chem Soc 96: 6388–6393. doi: 10.1021/ja00827a023
![]() |
[94] |
Wang M, Ma J, Liu H, et al. (2018) Sustainable productions of organic acids and their derivatives from biomass via selective oxidative cleavage of C–C Bond. ACS Catal 8: 2129–2165. doi: 10.1021/acscatal.7b03790
![]() |
[95] |
Van de Vyver S, Thomas J, Geboers J, et al. (2011) Catalytic production of levulinic acid from cellulose and other biomass-derived carbohydrates with sulfonated hyperbranched poly(arylene oxindole)s. Energy Environ Sci 4: 3601–3610. doi: 10.1039/c1ee01418h
![]() |
[96] |
Kong X, Wu S, Li X, et al. (2016) Efficient conversion of levulinic acid to ethyl levulinate over a silicotungstic-Acid-Modified commercially Silical-Gel sphere catalyst. Energy Fuels 30: 6500–6504. doi: 10.1021/acs.energyfuels.6b01207
![]() |
1. | Hiroshi Habe, Yuya Sato, Kohtaro Kirimura, Microbial and enzymatic conversion of levulinic acid, an alternative building block to fermentable sugars from cellulosic biomass, 2020, 104, 0175-7598, 7767, 10.1007/s00253-020-10813-7 | |
2. | Augustine Omoniyi Ayeni, Oluranti Agboola, Michael Olawale Daramola, Bianca Grabner, Babalola Aisosa Oni, Damilola Elizabeth Babatunde, Joseph Evwodere, Kinetic study of activation and deactivation of adsorbed cellulase during enzymatic conversion of alkaline peroxide oxidation-pretreated corn cob to sugar, 2021, 38, 0256-1115, 81, 10.1007/s11814-020-0667-2 | |
3. | Giulia Fiorani, Claudia Crestini, Maurizio Selva, Alvise Perosa, Advancements and Complexities in the Conversion of Lignocellulose Into Chemicals and Materials, 2020, 8, 2296-2646, 10.3389/fchem.2020.00797 | |
4. | Andrea Dell'Acqua, Bernhard M. Stadler, Sarah Kirchhecker, Sergey Tin, Johannes G. de Vries, Scalable synthesis and polymerisation of a β-angelica lactone derived monomer, 2020, 22, 1463-9262, 5267, 10.1039/D0GC00338G | |
5. | Puteri Nurain Syahirah Megat Muhammad Kamal, Nur Izzatti Mohamad, Magdalyn Empina Anak Serit, Nur Syafiiqah Abdul Rahim, Nur Izwani Jimat, Amin Safwan Alikasturi, Study on the effect of reaction and calcination temperature towards glucose hydrolysis using solid acid catalyst, 2020, 31, 22147853, 282, 10.1016/j.matpr.2020.06.008 | |
6. | Aderemi T. Adeleye, Chuks Kenneth Odoh, Obieze Christian Enudi, Oluwakemi Oluwabunmi Banjoko, Osigbeminiyi Oludare Osiboye, Emmanuel Toluwalope Odediran, Hitler Louis, Sustainable synthesis and applications of polyhydroxyalkanoates (PHAs) from biomass, 2020, 96, 13595113, 174, 10.1016/j.procbio.2020.05.032 | |
7. | Fatima Zahra Jawhari, Abdelfattah E. L. Moussaoui, Mohammed Bourhia, Hamada Imtara, Hamza Saghrouchni, Kenza Ammor, Hayat Ouassou, Youssef Elamine, Riaz Ullah, Essam Ezzeldin, Gamal A. E. Mostafa, Amina Bari, Anacyclus pyrethrum var. pyrethrum (L.) and Anacyclus pyrethrum var. depressus (Ball) Maire: Correlation between Total Phenolic and Flavonoid Contents with Antioxidant and Antimicrobial Activities of Chemically Characterized Extracts, 2021, 10, 2223-7747, 149, 10.3390/plants10010149 | |
8. | Sharath Bandibairanahalli Onkarappa, Navya Subray Bhat, Saikat Dutta, Preparation of alkyl levulinates from biomass-derived 5-(halomethyl)furfural (X = Cl, Br), furfuryl alcohol, and angelica lactone using silica-supported perchloric acid as a heterogeneous acid catalyst, 2020, 10, 2190-6815, 849, 10.1007/s13399-020-00791-1 | |
9. | Seng Hon Kee, Justin Brian V. Chiongson, Jonel P. Saludes, Sevakumaran Vigneswari, Seeram Ramakrishna, Kesaven Bhubalan, Bioconversion of agro-industry sourced biowaste into biomaterials via microbial factories – A viable domain of circular economy, 2021, 271, 02697491, 116311, 10.1016/j.envpol.2020.116311 | |
10. | Fatima Zahra Jawhari, Abdelfattah El Moussaoui, Mohammed Bourhia, Hamada Imtara, Hamza Mechchate, Imane Es-Safi, Riaz Ullah, Essam Ezzeldin, Gamal A. Mostafa, Andriy Grafov, Samir Ibenmoussa, Dalila Bousta, Amina Bari, Anacyclus pyrethrum (L): Chemical Composition, Analgesic, Anti-Inflammatory, and Wound Healing Properties, 2020, 25, 1420-3049, 5469, 10.3390/molecules25225469 | |
11. | Mulisa Maumela, Sanette Marx, Reinout Meijboom, Heterogeneous Ru Catalysts as the Emerging Potential Superior Catalysts in the Selective Hydrogenation of Bio-Derived Levulinic Acid to γ-Valerolactone: Effect of Particle Size, Solvent, and Support on Activity, Stability, and Selectivity, 2021, 11, 2073-4344, 292, 10.3390/catal11020292 | |
12. | Aderemi Timothy Adeleye, Amos Adeleke Akande, Chuks Kenneth Odoh, Monday Philip, Timothy Tizhe Fidelis, Pigweh Isa Amos, Oluwakemi Oluwabunmi Banjoko, Efficient synthesis of bio-based activated carbon (AC) for catalytic systems: A green and sustainable approach, 2021, 96, 1226086X, 59, 10.1016/j.jiec.2021.01.044 | |
13. | Qiang Lv, Hong Liu, Weichao Xu, Anming Li, Xuehan Zhang, Yuhang Yi, Kunhao Guo, Feng Guo, Selective Conversion of Glucose to Levulinic Acid Catalyzed by HPAs/MOF-5 in a Hydrophilic Eutectic Solvent System, 2022, 36, 0887-0624, 14994, 10.1021/acs.energyfuels.2c03015 | |
14. | Siyu Zhai, Lihe Zhang, Xi Zhao, Qian Wang, Yin Yan, Cui Li, Xu Zhang, Enzymatic synthesis of a novel solid–liquid phase change energy storage material based on levulinic acid and 1,4-butanediol, 2022, 9, 2197-4365, 10.1186/s40643-022-00502-w | |
15. | Célia Alves, Margarita Evtyugina, Ana Vicente, Kati Lehtoranta, Organic profiles of particulate matter emissions from a Euro VI diesel city bus, 2023, 16, 1873-9318, 183, 10.1007/s11869-022-01258-0 | |
16. | Muhammad Sajid, Usman Farooq, Ghulam Bary, Muhammad Mohsin Azim, Xuebing Zhao, Sustainable production of levulinic acid and its derivatives for fuel additives and chemicals: progress, challenges, and prospects, 2021, 23, 1463-9262, 9198, 10.1039/D1GC02919C | |
17. | Nadiah Syafiqah Mohd Azlan, Chiew Lin Yap, Suyin Gan, Mohd Basyaruddin Abdul Rahman, Recent advances in the conversion of lignocellulosic biomass and its degraded products to levulinic acid: A synergy of Brønsted-Lowry acid and Lewis acid, 2022, 181, 09266690, 114778, 10.1016/j.indcrop.2022.114778 | |
18. | Juan Gabriel Segovia-Hernández, Eduardo Sánchez-Ramírez, César Ramírez-Márquez, Gabriel Contreras-Zarazúa, 2022, 9780323898706, 127, 10.1016/B978-0-323-89870-6.00009-3 | |
19. | Emilia Soszka, Marcin Jędrzejczyk, Christophe Lefèvre, Dris Ihiawakrim, Nicolas Keller, Agnieszka M. Ruppert, TiO2-supported Co catalysts for the hydrogenation of γ-valerolactone to 2-methyltetrahydrofuran: influence of the support, 2022, 12, 2044-4753, 5802, 10.1039/D2CY01044E | |
20. | Yijun Tian, Fangfang Zhang, Jieni Wang, Leichang Cao, Qiuxia Han, A review on solid acid catalysis for sustainable production of levulinic acid and levulinate esters from biomass derivatives, 2021, 342, 09608524, 125977, 10.1016/j.biortech.2021.125977 | |
21. | Xinyu Liu, Zhaohui Li, Efficient transfer hydrogenation of levulinic acid (LA) to γ-valerolactone (GVL) over Ni/NiO–MC (MC = mesoporous carbon), 2021, 5, 2398-4902, 3312, 10.1039/D1SE00228G | |
22. | Supphathee Chaowamalee, Ning Yan, Chawalit Ngamcharussrivichai, Propylsulfonic Acid-Functionalized Mesostructured Natural Rubber/Silica Nanocomposites as Promising Hydrophobic Solid Catalysts for Alkyl Levulinate Synthesis, 2022, 12, 2079-4991, 604, 10.3390/nano12040604 | |
23. | Julio A. Conti Silva, Luan M. Grilo, Marcelo H. Vasconcelos, Talita M. Lacerda, 2022, 9780128235317, 387, 10.1016/B978-0-12-823531-7.00002-0 | |
24. | Aristide Laurel Mokale Kognou, Sarita Shrestha, Zihua Jiang, Chunbao (Charles) Xu, Fubao Sun, Wensheng Qin, High-fructose corn syrup production and its new applications for 5-hydroxymethylfurfural and value-added furan derivatives: Promises and challenges, 2022, 7, 23699698, 148, 10.1016/j.jobab.2022.03.004 | |
25. | Daiana A. Bravo Fuchineco, Angélica C. Heredia, Sandra M. Mendoza, Enrique Rodríguez-Castellón, Mónica E. Crivello, Esterification of Levulinic Acid to Methyl Levulinate over Zr-MOFs Catalysts, 2022, 6, 2305-7084, 26, 10.3390/chemengineering6020026 | |
26. | Andrea Merenda, Samantha A. Orr, Yang Liu, Blanca Hernández Garcia, Amin Osatiashtiani, Gabriel Morales, Marta Paniagua, Juan A. Melero, Adam F. Lee, Karen Wilson, Continuous flow (Sulfated) Zirconia Catalysed Cascade Conversion of Levulinic Acid to γ‐Valerolactone, 2023, 15, 1867-3880, 10.1002/cctc.202201224 | |
27. | Vikneswary Rajendaren, Syed M. Saufi, M. A. K. M. Zahari, A review of the methods for levulinic acid separation and extraction, 2022, 2190-6815, 10.1007/s13399-022-03444-7 | |
28. | Aderemi Timothy Adeleye, Kingsley I. John, Promise Goodness Adeleye, Amos Adeleke Akande, Oluwakemi Oluwabunmi Banjoko, One-dimensional titanate nanotube materials: heterogeneous solid catalysts for sustainable synthesis of biofuel precursors/value-added chemicals—a review, 2021, 56, 0022-2461, 18391, 10.1007/s10853-021-06473-1 | |
29. | Daniele Di Menno Di Bucchianico, Antonella Cipolla, Jean-Christophe Buvat, Mélanie Mignot, Valeria Casson Moreno, Sébastien Leveneur, Kinetic Study and Model Assessment for n-Butyl Levulinate Production from Alcoholysis of 5-(Hydroxymethyl)furfural over Amberlite IR-120, 2022, 61, 0888-5885, 10818, 10.1021/acs.iecr.2c01640 | |
30. | Andrea Dell’Acqua, Lukas Wille, Bernhard M. Stadler, Sergey Tin, Johannes G. de Vries, Ozonolysis of α-angelica lactone: a renewable route to malonates, 2021, 57, 1359-7345, 10524, 10.1039/D1CC03820F | |
31. | Fatima El Ouahabi, Wietse Smit, Carlo Angelici, Mykola Polyakov, Uwe Rodemerck, Christine Fischer, V. Narayana Kalevaru, Sebastian Wohlrab, Sergey Tin, Gerard P. M. van Klink, Jan C. van der Waal, François Orange, Johannes G. de Vries, Conversion of Biomass-Derived Methyl Levulinate to Methyl Vinyl Ketone, 2022, 10, 2168-0485, 766, 10.1021/acssuschemeng.1c05694 | |
32. | Emilia Soszka, Marcin Jȩdrzejczyk, Nicolas Keller, Agnieszka M. Ruppert, High yield production of 2-methyltetrahydrofuran biofuel with reusable Ni-Co catalysts, 2023, 332, 00162361, 126118, 10.1016/j.fuel.2022.126118 | |
33. | Daniele Di Menno Di Bucchianico, Yanjun Wang, Jean-Christophe Buvat, Yong Pan, Valeria Casson Moreno, Sébastien Leveneur, Production of levulinic acid and alkyl levulinates: a process insight, 2022, 24, 1463-9262, 614, 10.1039/D1GC02457D | |
34. | Svetlana A. Sorokina, Stepan P. Mikhailov, Nina V. Kuchkina, Alexey V. Bykov, Alexander L. Vasiliev, Mariam G. Ezernitskaya, Andrey L. Golovin, Linda Zh. Nikoshvili, Mikhail G. Sulman, Zinaida B. Shifrina, Ru@hyperbranched Polymer for Hydrogenation of Levulinic Acid to Gamma-Valerolactone: The Role of the Catalyst Support, 2022, 23, 1422-0067, 799, 10.3390/ijms23020799 | |
35. | Liang Jiang, Guangyue Xu, Yao Fu, A nitrogen-doped carbon modified nickel catalyst for the hydrogenation of levulinic acid under mild conditions, 2021, 23, 1463-9262, 7065, 10.1039/D1GC01732B | |
36. | Goldi Sharma, S. Kamalesu, Review on sustainable synthesis of semi-amorphous Ti-BDS MOF material, activated carbon, and graphene, 2023, 22147853, 10.1016/j.matpr.2023.01.249 | |
37. | Priyanka Gautam, Sanghamitra Barman, Amjad Ali, A comparative study on the performance of acid catalysts in the synthesis of levulinate ester using biomass‐derived levulinic acid: a review, 2022, 16, 1932-104X, 1095, 10.1002/bbb.2351 | |
38. | 2023, 9781119814665, 19, 10.1002/9781119814719.ch2 | |
39. | Sarah Capecci, Yanjun Wang, Jose Delgado, Valeria Casson Moreno, Mélanie Mignot, Henrik Grénman, Dmitry Yu. Murzin, Sébastien Leveneur, Bayesian Statistics to Elucidate the Kinetics of γ-Valerolactone from n-Butyl Levulinate Hydrogenation over Ru/C, 2021, 60, 0888-5885, 11725, 10.1021/acs.iecr.1c02107 | |
40. | Zhe Sun, Heng Zhao, Xinti Yu, Jinguang Hu, Zhangxin Chen, Glucose photorefinery for sustainable hydrogen and value-added chemicals coproduction, 2024, 4, 2769-5247, 10.20517/cs.2023.41 | |
41. | Jiaye Tang, Yulei Chen, Qian Li, Wenli Xin, Ximeng Xiao, Xuemei Chen, Lixi Yang, Borui Mou, Jialian Li, Fujia Lu, Chun Fu, Wencong Long, Hong Liao, Xuebing Han, Peng Feng, Wei Li, Kedi Zhou, Liuyun Yang, Yaojun Yang, Menggen Ma, Hanyu Wang, The response mechanism analysis of HMX1 knockout strain to levulinic acid in Saccharomyces cerevisiae, 2024, 15, 1664-302X, 10.3389/fmicb.2024.1416903 | |
42. | Bhanu Priya, Vinod K. Sahu, Sanjay K. Singh, Ruthenium catalyzed transformation of levulinic acid to γ-valerolactone in water, 2024, 2, 2753-8125, 390, 10.1039/D3SU00285C | |
43. | Sunday Blessing Oladipupo, Ayanniyi Mufutau Ayanshola, Aderemi Timothy Adeleye, Kingsley Igenepo John, Assessment of plastic waste generation and management in residential locations, Ilorin, Nigeria: DPSIR analysis and circular economy pathway, 2024, 2731-6734, 10.1007/s43994-024-00130-4 | |
44. | Umasankar Samidurai, Santhana Krishnan Perumal, Srinivasan Vinju Vasudevan, Shanthi Kannan, Enhanced catalytic performance of ethyl-levulinate to γ-valerolactone: Effect of copper loading on Ni/KIT-5 catalyst, 2024, 90, 03603199, 534, 10.1016/j.ijhydene.2024.09.427 | |
45. | Rahul V. Prajapati, Sanjay Srivastava, Girirajsinh C. Jadeja, Jigisha Parikh, Optimization and kinetics study for the conversion of furfuryl alcohol towards ethyl levulinate using sulfonic acid functionalized catalyst, 2024, 22, 1542-6580, 323, 10.1515/ijcre-2023-0133 | |
46. | Nguyen Thi Hue, Truong Thi Thanh, Nguyen Hoang Chung, Le Quang Dien, Phan Huy Hoang, Preparation of sulfonated carbonaceous solid acid catalysts by fuming sulfuric acid from different lignocellulosic sources and application for the conversion of Acacia mangium wood sawdust-derived glucose and fructose into levulinic acid, 2024, 82, 0018-3768, 529, 10.1007/s00107-023-02017-3 | |
47. | Wenting Fang, Anders Riisager, Advances in Aluminophosphates for Catalytic Upgrading of Lignocellulose and Derived Compounds, 2024, 1867-3880, 10.1002/cctc.202400394 | |
48. | Théo Faverge, Antoine Bonnefont, Marian Chatenet, Christophe Coutanceau, Glucose oxidation on gold in alkaline solution: A DEMS and microkinetic modeling study, 2024, 508, 00134686, 145269, 10.1016/j.electacta.2024.145269 | |
49. | Margarita Popova, Momtchil Dimitrov, Silviya Boycheva, Ivan Dimitrov, Filip Ublekov, Neli Koseva, Genoveva Atanasova, Daniela Karashanova, Ágnes Szegedi, Ni-Cu and Ni-Co-Modified Fly Ash Zeolite Catalysts for Hydrodeoxygenation of Levulinic Acid to γ-Valerolactone, 2023, 29, 1420-3049, 99, 10.3390/molecules29010099 | |
50. | Yan Ma, Hongxiao Wang, Ziyang Wu, Weihong Tan, Guodong Feng, Jianchun Jiang, A process insight into production of ethyl levulinate via a stepwise fractionation, 2024, 9, 23699698, 233, 10.1016/j.jobab.2023.11.001 | |
51. | Kalpana Maheria, Ramesh Kumar Chowdari, Janusz Kozinski, Ajay Kumar Dalai, Synthesis of n-Butyl Levulinate Over Caesium Containing Heteropoly Acid Supported Zeolite β Catalysts, 2024, 28, 1571-1013, 36, 10.1007/s10563-023-09410-1 | |
52. | Hani Zeidan, Mustafa Esen Marti, Selective and efficient separation of levulinic, acetic and formic acids from multi-acid solutions by adjusting process parameters, 2023, 56, 22147144, 104299, 10.1016/j.jwpe.2023.104299 | |
53. | Xuran Ji, Zhijie Shen, Can Wang, Shimiao Yao, Lian Xiong, Hairong Zhang, Hongcai Zhou, Xuefang Chen, Xinde Chen, Amino‐functionalized hyper‐cross‐linked resins for levulinic acid adsorption from sugarcane bagasse hydrolysate, 2023, 98, 0268-2575, 2958, 10.1002/jctb.7502 | |
54. | Luigi di Bitonto, Enrico Scelsi, Massimiliano Errico, Hilda Elizabeth Reynel-Ávila, Didilia Ileana Mendoza-Castillo, Adrián Bonilla-Petriciolet, Marcos Lucio Corazza, Luis Ricardo Shigueyuki Kanda, Martin Hájek, Roumiana P. Stateva, Carlo Pastore, A Network of Processes for Biorefining Burdock Seeds and Roots, 2024, 29, 1420-3049, 937, 10.3390/molecules29050937 | |
55. | Amar Al-khawlani, Jiehua Bao, Xiaoli Sheng, Pingping Yu, Basheer M. Al-Maswari, Awatif Rashed Z. Almotairy, Yiwei Zhang, Zhou Yuming, SSZ-39 zeolite-based Ru catalysts for selective hydrogenation of levulinic acid to γ-valerolactone: Influence of synthesis method and zeolite acidity, 2024, 372, 13871811, 113112, 10.1016/j.micromeso.2024.113112 | |
56. | Fabrícia Vieira, Hortência E. P. Santana, Meirielly Jesus, Joana Santos, Preciosa Pires, Manuela Vaz-Velho, Daniel Pereira Silva, Denise Santos Ruzene, Coconut Waste: Discovering Sustainable Approaches to Advance a Circular Economy, 2024, 16, 2071-1050, 3066, 10.3390/su16073066 | |
57. | Fabrícia Vieira, Hortência E. P. Santana, Meirielly Jesus, Fernando Mata, Preciosa Pires, Manuela Vaz-Velho, Daniel Pereira Silva, Denise Santos Ruzene, Comparative Study of Pretreatments on Coconut Fiber for Efficient Isolation of Lignocellulosic Fractions, 2024, 16, 2071-1050, 4784, 10.3390/su16114784 | |
58. | Olunusi Samuel Olugbenga, Promise Goodness Adeleye, Sunday Blessing Oladipupo, Aderemi Timothy Adeleye, Kingsley Igenepo John, Biomass-derived biochar in wastewater treatment- a circular economy approach, 2024, 1, 29497507, 1, 10.1016/j.wmb.2023.07.007 | |
59. | Aayushi Lodhi, Kalpana C. Maheria, Zeolite-catalysed esterification of biomass-derived acids into high-value esters products: Towards sustainable chemistry, 2024, 187, 15667367, 106883, 10.1016/j.catcom.2024.106883 | |
60. | Luis Alejandro Arriaga-Arellano, Débora Álvarez-Hernández, María Isabel Domínguez, Marcela Martínez T., Anna Penkova, Svetlana Ivanova, Miguel Ángel Centeno, Levulinic to succinic acid transformation over Ru based catalysts, 2024, 3, 29498228, 100059, 10.1016/j.nxmate.2023.100059 | |
61. | Deshou Wang, Yumeng Liang, Yong Zeng, Chang Liu, Chun Zhan, Peng Chen, Shaoxian Song, Feifei Jia, Highly selective recovery of gold and silver from E-waste via stepwise electrodeposition directly from the pregnant leaching solution enabled by the MoS2 cathode, 2024, 465, 03043894, 133430, 10.1016/j.jhazmat.2024.133430 | |
62. | Csaba Árvai, Zoltán Medgyesi, Matthew Y. Lui, László T. Mika, The Chemistry of Levulinic Acid: Its Potential in the Production of Biomass‐Based Chemicals, 2024, 1615-4150, 10.1002/adsc.202401086 | |
63. | Anand Kumar Pandey, Jayanti Awasthi, Kislay Chaturvedi, Ayush Mishra, Shivangi Yadav, Soumya Rathore, Preeti Birwal, In silico Evaluation of Anacyclus pyrethrum Composition for Inhibition of Spike RBD-ACE-2 Interaction to Treat COVID-19, 2025, 6, 26667967, 10.2174/0126667975280881240102111455 | |
64. | Laksamee Jeanmard, Wichitpan Rongwong, Yusuf Chisti, Biomass-derived levulinic acid as a platform chemical for making diverse products, 2025, 195, 09619534, 107683, 10.1016/j.biombioe.2025.107683 | |
65. | Johannes G de Vries, 2025, 9780443289231, 274, 10.1016/B978-0-443-15742-4.00149-6 |