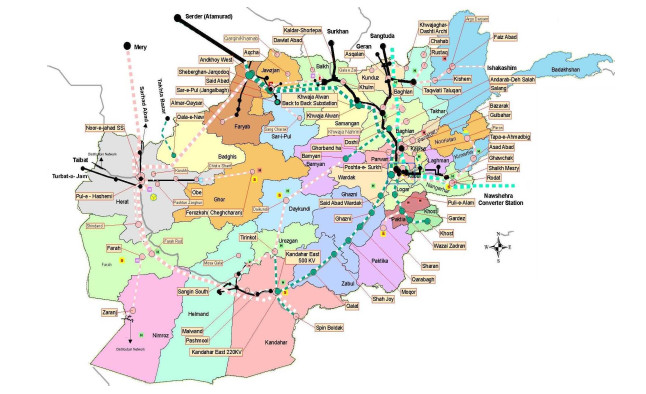
Citation: Dewey Brooke, Navid Movahed, Brian Bothner. Universal buffers for use in biochemistry and biophysical experiments[J]. AIMS Biophysics, 2015, 2(3): 336-342. doi: 10.3934/biophy.2015.3.336
[1] | Ferhat Bingol . Feasibility of large scale wind turbines for offshore gas platform installation. AIMS Energy, 2018, 6(6): 967-978. doi: 10.3934/energy.2018.6.967 |
[2] | YingTung Chen, Kristina Knüpfer, Miguel Esteban, Tomoya Shibayama . Analysis of the impact of offshore wind power on the Japanese energy grid. AIMS Energy, 2023, 11(1): 110-134. doi: 10.3934/energy.2023006 |
[3] | Navid Majdi Nasab, Jeff Kilby, Leila Bakhtiaryfard . Integration of wind and tidal turbines using spar buoy floating foundations. AIMS Energy, 2022, 10(6): 1165-1189. doi: 10.3934/energy.2022055 |
[4] | Ladislas Mutunda Kangaji, Atanda Raji, Efe Orumwense . Cutting-edge progress in offshore wind and tidal stream power technology—State-of-the-Art. AIMS Energy, 2025, 13(1): 188-230. doi: 10.3934/energy.2025007 |
[5] | Wen-Tien Tsai . Overview of wind power development over the two past decades (2000-2019) and its role in the Taiwan's energy transition and sustainable development goals. AIMS Energy, 2021, 9(2): 342-354. doi: 10.3934/energy.2021018 |
[6] | Saiyad S. Kutty, M. G. M. Khan, M. Rafiuddin Ahmed . Estimation of different wind characteristics parameters and accurate wind resource assessment for Kadavu, Fiji. AIMS Energy, 2019, 7(6): 760-791. doi: 10.3934/energy.2019.6.760 |
[7] | Rashid Al Badwawi, Mohammad Abusara, Tapas Mallick . Speed control of synchronous machine by changing duty cycle of DC/DC buck converter. AIMS Energy, 2015, 3(4): 728-739. doi: 10.3934/energy.2015.4.728 |
[8] | Ivan Nygaard, Famakan Kamissoko, Per Bromand Nørgård, Jake Badger, Luc Dewilde . Feasibility of wind power integration in weak grids in non-coastal areas of sub-saharan Africa: the case of Mali. AIMS Energy, 2017, 5(3): 557-584. doi: 10.3934/energy.2017.3.557 |
[9] | Azevedo Joaquim, Mendonça Fábio . Small scale wind energy harvesting with maximum power tracking. AIMS Energy, 2015, 2(3): 297-315. doi: 10.3934/energy.2015.3.297 |
[10] | Hassam Nasarullah Chaudhry, John Kaiser Calautit, Ben Richard Hughes . The Influence of Structural Morphology on the Efficiency of Building Integrated Wind Turbines (BIWT). AIMS Energy, 2014, 2(3): 219-236. doi: 10.3934/energy.2014.3.219 |
Modern civilization severely depends on electrical energy for agricultural, commercial, domestic, industrial and social needs purposes. To avoid expanding the electrical systems, capacitor banks are widely installed across the existing networks. Using capacitor bank offers an economical method to provide voltage support, reactive power support, and to increase power transfer capability. They are also used to compensate the XI$ ^2 $ losses in transmission systems. Capacitor banks are comparatively cost-effective and can easily be installed anywhere on the electrical network. Generation capacity release; system capacity enhancement; power losses reduction; voltage regulation, and power quality improvement are the benefits that can be achieved by SCBs installation in electrical system [1].
However, capacitor banks can create some inconveniences among which transient inrush/outrush current with a high amplitude and frequency is significant. Inrush current is produced due to load variation which results in several switching which are needed for connecting and disconnecting the capacitor bank in a typical day. Outrush current is produced when a breaker is operating to disconnect the nearby faulted circuit. The magnitude and frequency of discharged outrush current are dependent upon the total equivalent inductance between the capacitor bank and the faulted point. The inrush and outrush current conduct by a transient surge can affect the supply quality and exceed the withstand capability of the equipment [2,3,4,5].
The propose of this study is, to analyse the inconveniences created by the installed SCBs along the NEPS in Afghanistan. Supply capacity in Afghanistan consists of import power and domestic hydro and thermal resources [6,7,8,9]. NEPS is the leading energy system, provides lower-cost power to cities and towns in the Northeast parts, including Kabul, the capital city. It encompasses import power from three neighbouring countries (Uzbekistan, Tajikistan, and Turkmenistan). NEPS, as the largest electrical system supplied 77% of all electricity demand in 2011. As the power system of exporter countries are not synchronised, it obliges the country to handle the NEPS in isolated regions which each unified with its nearby supplier. However, could all be interconnected if the supplier countries decided to operate either in synchronisation or agreed to interconnect through High Voltage Direct Current (HVDC) [10,11,12]. The existing and planned electricity grid in Afghanistan, is presented in Figure 1 [13].
Due to unsynchronized operation of the system, a main part of the NEPS which is called NEPS-Uzbekistan is evaluated in this study. Depending on the grounding of the capacitor bank and types of the fault, the amplitude and frequency of the outrush transient can exceed the rating withstand limit of equipment and impose severe stress on the system. For further consideration two scenarios are evaluated to mitigate the outrush current generated by 25 Mvar and 120 Mvar capacitor banks at Pule Khumri and Chimtala substations respectively. To limit the amplitude and frequency, as well as the Rate of Rise of Outrush Current (RROC), CLI is integrated with each involved capacitor bank. As the use of CLI would otherwise create some excessive voltage, a sensitivity analysis based on Transient Recovery Voltage (TRV) is applied as well. The simulation for this study is performed by the Alternative Transients Program (ATP), which is a version of Electromagnetic Transient Program (EMTP).
Capacitor banks are assembled by the series/parallel combination of capacitor units (Figure 2). Most of the capacitor banks at distribution and transmission level are Y connected and are either grounded or ungrounded, as shown in Figure 3. Capacitor banks are comparatively cost-effective and can easily be installed anywhere in electrical networks [14,15,16]. Besides their multiple benefits, capacitor banks can impose some inconveniences, which severely affect power quality on sensitive customer loads. It may also damage key equipment, potentially having a high impact on system reliability. To study dangers caused by transient inrush/outrush current, time-domain computer programs are developed [17,18].
Capacitor banks switching transients are becoming more critical especially with increasing the rate of its installations in power systems. This is because of frequent utility operations, occurring due to multiple switching per day and hundreds of time per year depending on the need for system voltage/var support from the capacitor banks. Switching a capacitor bank makes a change in circuit which a finite amount of time is required to approach a stable operating point. In capacitive discharge, there is a fixed energy amount depends on the stored capacity of involved capacitor banks just before switching and the amount store immediately after the event. Switching transient is produced due to voltage differences between the system and installed capacitor bank, characterized by high magnitude and frequency [19,20]. The maximum inrush current produce by SCB is derived from Eq (2.1). Transient inrush current has to be limited so as not to be harmful to the circuit breakers, capacitor bank, and the network. There are two possibilities to limit switching inrush current. The first one is to connect the capacitor at an exact time such that it can match the system voltage, and another way is to use a CLI in series with the capacitor bank [21,22].
$ Imax=√2×V×√CBLS $
|
(2.1) |
where $ C_B $ is the capacitance of the shunt capacitor bank, $ L_S $ is system inductance, and $ V $ is a line to ground voltage.
Transient outrush current with high amplitude and frequency is associated with a capacitor bank and the breaker when disconnecting a nearby faulted circuit. In capacitive discharge, there is a fixed amount of energy which depends on the energy stored in capacitance just before the event. In such a situation, depending upon the grounding of the capacitor bank and types of the fault, it can break the withstand capability limit of the circuit breaker, strike the capacitor bank and affect whole the system. The magnitude and frequency of outrush transient are dependent upon the total equivalent inductance between the capacitor bank and the fault, capacitor bank rating, and the point on the voltage waveform where the breaker disconnects the circuit [23,24,25]. For further evaluation, a typical system layout and an equivalent circuit are indicated in Figures 4 and 5, respectively. Use of CLI is an important discussion to mitigate the inrush/outrush current and protect the electrical systems. In Y connected systems, CLI can be used in different places between the switching device and the capacitor, between the bus and switching device, or between the end of each capacitor bnak and the neutral point. Technically it is also possible to distribute it throughout the capacitor bank (small inductors for each capacitor unit). Sizing the CLI should be done in such a way that the transient inrush/outrush currents through the switching device will not exceed its capability. The frequency and maximum capacity of outrush current are illustrated in Eqs (2.2) and (2.3) respectively [26,27,28].
$ f=12Π√LeqC1 $
|
(2.2) |
$ ipk=V0×√C1Leq $
|
(2.3) |
where; $ f $ is frequency of transient outrush current, $ C_1 $ is the total capacitance of discharging capacitor bank, $ V_0 $ is initial voltage of $ C_1 $, $ L_1 $ is self inductance of $ C_1 $, $ L_2 $ is inductance between capacitor and fault and $ L_{eq} $ is equivalent inductance. For a three phase capacitor bank, a reasonable estimate of the peak outrush current is illustrated in Eq (2.4):
$ ipk=√10003πf×√QckvarL $
|
(2.4) |
For 50 and 60 Hertz systems the equations are approximated as shown in the following equations:
$ ipk=32√QckvarL $
|
(2.5) |
$ ipk=43√QckvarL $
|
(2.6) |
where; $ i_{pk} $ is peak current [kA], $ f $ is power system frequency [Hz], $ Q_c $ is capacitor bank rating [Kvar], and $ L $ is inductance per phase between capacitor bank and fault [$ \mu $ H].
Although hand calculations can make some estimations of capacitor outrush transients, an EMTP study is required to model the complex behaviours of the power system. Once the model is developed, many scenarios can be simulated and detailed statistical studies can be performed. In this study two scenarios are considered to investigate the challenges created by installed SCBs along NEPS. Through the first scenario, outrush current is evaluated during three phases to ground fault, nearby 25 Mvar capacitor bank in Pule Khumri substation (Figure 6). Based on simulation result, an outrush current is injected by the installed capacitor bank toward the faulted point when circuit breaker (S-PL-3) operates to disconnect the circuit. Outrush current produced with high amplitude (11.5 kA) and considerable rate of rising (2.61 kA/$ \mu $s) as presented in Figure 7 can severely damage the circuit breakers and unstable whole the system. To properly visualize the negative impacts of installed SCB into a nearby fault, the same scenario is considered with ignoring capacitor bank, as result has shown in Figure 8.
In the second scenario, the outrush current is evaluated by assuming three phases to ground fault at Chimtala substation, as presented in Figure 9. Based on calculation and simulation result, an outrush transient with a peak value of 6.5 kA and intensive rate of raising of 2.14 kA/$ \mu $s is injected by the installed 120 Mvar SCB into the faulted point as shown in Figure 10.
To mitigate the injected outrush current as well as to protect the system, 3 mH and 2 mH inductors are applied in series with each considered capacitor banks in Pule Khumri and Chimtala substations respectively. The values of integrated CLIs are calculated based on trial and error experiments as well as a minimum/maximum allowable limit to fulfil the network's withstand capability. Diagram of the system and mitigated outrush current for each considered scenario is presented in Figures 11–13, respectively.
CLIs application along NEPS provoke a possibility that if a fault occurs in such a way an excessive TRV can be imposed across the contacts of the circuit breaker when disconnecting the circuit. This is due to the high frequency of the inductor, which causes high-frequency oscillation on the load side of the circuit breaker when their is an attempt to interrupt the faulted circuit. TRV is a voltage, recovers or jumps back up to rated level across the contacts of a circuit breaker in electrical networks [29,30]. To confirm the robustness of the proposed approach, a sensitivity analysis based on TRV is applied in this study as well. For further evaluation, an EMTP calculation is performed to evaluate the TRV across the contacts of circuit breaker (S-PL-2) when a three-phase to ground fault considered in Pule Khumri substation. The result is shown in Figure 14 confirm that there is no excessive voltage produced by integrated CLIs on the NEPS.
Fast transients due to inrush/outrush produce by capacitor banks causes a change in circuit configuration and create high di/dt in electrical networks. Transient currents and other power quality disturbances cause techno-economical losses due to damage of equipment and loss of electricity production. CLI can be used to reduce the di/dt and enhance, transient ground rise potentials. It often used in series with each capacitor bank to limit transient currents during switching (inrush current) or faults (outrush current). This study investigated the transient outrush current created by the installed SCBs along the NEPS in Afghanistan. For appropriate investigation, the calculations are performed by using EMTP, ATP package. Based on the simulation results, the energized SCBs are gating discharge with a high amplitude and frequency into the nearby fault when the associated circuit breaker is disconnecting the circuit. To mitigate the outrush transient and protect the system, a set of 3 mH and 2 mH CLI is integrated with both installed SCBs in Pule Khumri and Chimtala substations respectively [31].
Sizing the fixed inductors has been done in such a way that outrush currents through the switching device would not exceed the device's capability. The values of CLIs are calculated based on trial and error experiments as well as a minimum/maximum allowable limit to fulfil the network's withstand capability. Limited outrush current for considered locations are indicated in Table 1. CLI integration otherwise may cause some unfortunate consequences by causing overvoltage that can fail circuit breakers. To limit the severity of the initial TRV across contacts of circuit breaker and confirm the robustness of the proposed approach, a sensitivity analysis based on TRV, is applied as well. Based on simulation result the peak and voltage acceleration associated with the initial TRV is less than that resulting from the ANSI-specified rate of rising of recovery voltage of 1.8 kV/$ \mu $s [30].
Capacitor location | Imax [kA] | RROC [kA/$\mu$ sec] | Applied reactor [mH] | Imax [kA] limited | RROC [kA/$\mu$ sec] limited |
Puli Khumri | 11.5 | 2.61 | 3 | 4.6 | 18.5 |
Cimtala | 6.5 | 2.14 | 2 | 4.3 | 15.3 |
Though their are numerous advantage associated with the use of capacitor bank for voltage improvement in power system. However, it usually has some drawbacks in terms of transient currents which affect the quality of power supply and exceed the withstand capability of associated equipment. This paper investigated, inconveniences caused due to outrush current injected by capacitor banks into nearby faulted point along NEPS in Afghanistan. Enhancing the capability of associated equipment with higher dielectric tolerance to withstand outrush transient, require higher renovation cost, which is economically infeasible. The CLI integrated with each considered capacitor bank, successfully reduced the severity of outrush currents, enhanced the interrupting capability of circuit breakers and improved the quality of power supply. As the use of CLI would otherwise create some excessive TRV which could exceed the withstand capability of circuit breakers. Therefore, a sensitivity analysis based on TRV is applied to confirm the robustness of the proposed approach. This approach allowed the mitigation of outrush current problem without exceeding the TRV across contacts of circuit breakers. Overall the safety and reliability of the NEPS are enhanced by using the proposed configuration.
For this paper, the authors acknowledge the support of the University of the Ryukyus through JICA (PEACE project).
All authors declare no conflicts of interest in this paper.
[1] |
Pascal SM, Yamazaki T, Singer AU, et al. (1995) Structural and dynamic characterization of the phosphotyrosine binding region of a Src homology 2 domain--phosphopeptide complex by NMR relaxation, proton exchange, and chemical shift approaches. Biochemistry 34:11353-11362. doi: 10.1021/bi00036a008
![]() |
[2] |
Zhang M, Zhou M, van Etten RL, et al. (1997) Crystal structure of bovine low molecular weight phosphotyrosyl phosphatase complexed with the transition state analog vanadate. Biochemistry 36:15-23. doi: 10.1021/bi961804n
![]() |
[3] |
Katayama DS, Nayar R, Chou DK, et al. (2006) Effect of buffer species on the thermally induced aggregation of interferon-tau. J Pharm Sci 95:1212-1226. doi: 10.1002/jps.20471
![]() |
[4] |
Hausamen TU, Helger R, Rick W, et al. (1967) Optimal conditions for determination of serum alkaline phosphatase by a new kinetic method. Clin Chim Acta 15: 241-245. doi: 10.1016/0009-8981(67)90060-5
![]() |
[5] | McComb RB, Bowers GN (1972) Study of optimum buffer conditions for measuring alkaline-phosphatase activity in human serum. Clin Chem 18: 97-103. |
[6] | Ganichkin OM, Xu XM, Carlson BA, et al. (2008) Structure and catalytic mechanism of eukaryotic selenocysteine synthase. J Biol Chem 283: 5849-5865. |
[7] | Long D, Yang D (2009) Buffer Interference with Protein Dynamics: A Case Study on Human Liver Fatty Acid Binding Protein. Biophys J 96 1482-1488. |
[8] |
Sopkova J, Renouard M, Lewit-Bentley A (1993) The crystal structure of a new high-calcium form of annexin V. J Mol Biol 234: 816-825. doi: 10.1006/jmbi.1993.1627
![]() |
[9] |
Kumar S, Sharma P, Arora K, et al. (2014) Calcium binding to beta-2-microglobulin at physiological pH drives the occurrence of conformational changes which cause the protein to precipitate into amorphous forms that subsequently transform into amyloid aggregates. PLoS One 9: e95725. doi: 10.1371/journal.pone.0095725
![]() |
[10] |
Schmitt FJ, Thaa B, Junghans C, et al. (2014) eGFP-pHsens as a highly sensitive fluorophore for cellular pH determination by fluorescence lifetime imaging microscopy (FLIM). Biochim Biophys Acta 1837: 1581-1593. doi: 10.1016/j.bbabio.2014.04.003
![]() |
[11] |
Bothner B, Schneemann A, Marshall D, et al. (1999) Crystallographically identical virus capsids display different properties in solution. Nat Struct Bio 6: 114-116. doi: 10.1038/5799
![]() |
[12] |
Bothner B, Taylor D, Jun B, et al. (2005) Maturation of a tetravirus capsid alters the dynamic properties and creates a metastable complex. Virology 339: 145-145. doi: 10.1016/j.virol.2005.04.016
![]() |
[13] |
Nam HJ, Gurda BL, McKenna R, et al. (2011) Structural Studies of Adeno-Associated Virus Serotype 8 Capsid Transitions Associated with Endosomal Trafficking. J Virol 85: 11791-11799. doi: 10.1128/JVI.05305-11
![]() |
[14] |
Bartlett JS, Wilcher R, Samulski RJ (2000) Infectious entry pathway of adeno-associated virus and adeno-associated virus vectors. J Virol 74: 2777-2785. doi: 10.1128/JVI.74.6.2777-2785.2000
![]() |
[15] |
Roos WH, Bruinsma R, Wuite GJL (2010) Physical virology. Nat Phys 6: 733-743. doi: 10.1038/nphys1797
![]() |
[16] |
Ellis DA (1961) New universal buffer system. Nature 191: 1099-1100. doi: 10.1038/1911099a0
![]() |
[17] | Henderson Y (1908) Acapnia and Shock: Carbon-dioxid as a factor in the regulation of the heart-rate. Am J Physiol 21: 126-156. |
[18] |
Good NE, Winget GD, Winter W, et al. (1966) Hydrogen ion buffers for biological research. Biochemistry 5: 467-472. doi: 10.1021/bi00866a011
![]() |
[19] | Garrett RH, Charles M, Grisham CM (2012). Biochemistry 5th ed. Cengage Learning. |
[20] | Sokolowska M, Bal J (2005) Cu(II) complexation by “non-coordinating” N-2-hydroxyethylpiperazine-N'-2-ethanesulfonic acid (HEPES buffer) J Inorg Biochem 99: 1653-1660. |
[21] |
Taha M (2011) Complex Equilibria in Aqueous Solutions of Chromium(III) with Some Biological pH Buffers. J Chem Eng Data 56: 3541-3551. doi: 10.1021/je200345a
![]() |
[22] |
Shnyrov VL, SanchezRuiz JM, Boiko BN, et al. (1997) Applications of scanning microcalorimetry in biophysics and biochemistry. Thermochim Acta 302: 165-180. doi: 10.1016/S0040-6031(97)00238-4
![]() |
[23] | Lakowicz J (2006) Principles of Fluorescence Spectroscopy. New York: Springer. |
Capacitor location | Imax [kA] | RROC [kA/$\mu$ sec] | Applied reactor [mH] | Imax [kA] limited | RROC [kA/$\mu$ sec] limited |
Puli Khumri | 11.5 | 2.61 | 3 | 4.6 | 18.5 |
Cimtala | 6.5 | 2.14 | 2 | 4.3 | 15.3 |