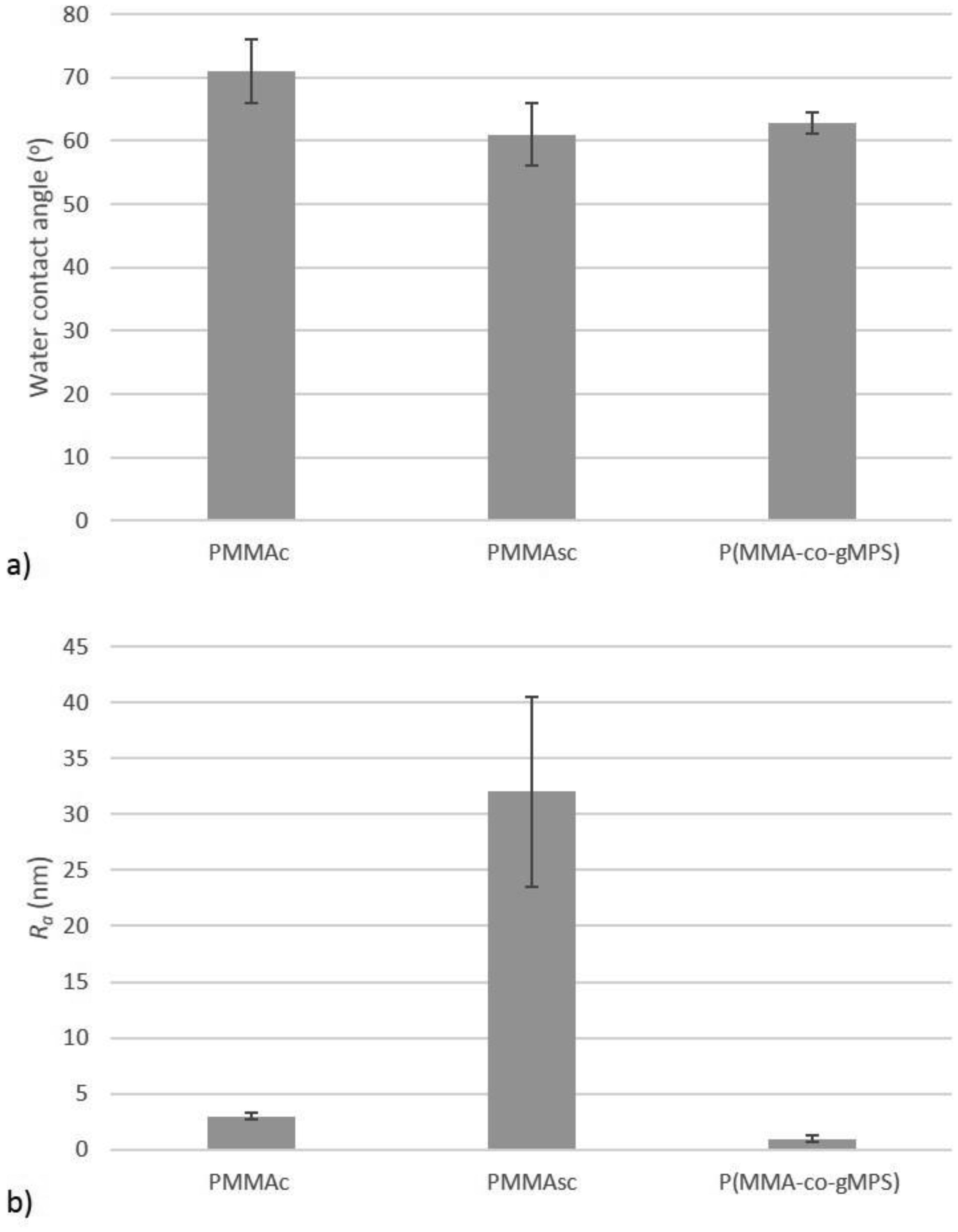
Poly(methyl methacrylate) (PMMA) surfaces, (commercial PMMA (PMMAc), spin coated PMMA (PMMAsc) and a 90% methylmethacrylate/10% 3-methacryloxypropyltrimethoxysilane random copolymer (P(MMA-co-gMPS)) were used to determine the effect of surface properties on conidia biofouling. The contact angles of the substrates demonstrated that the PMMAsc and the P(MMA-co-gMPS) polymer (62.8°) were more wettable than the PMMAc surface (71.0°). The PMMAsc had the greatest roughness value (32.0 nm) followed by the PMMAc (3.0 nm), then P(MMA-co-gMPS) (1 nm). Aspergillus niger 1957 conidia were spherical, smooth and hydrophobic (12.1%). Aspergillus niger 1988 conidia were spherical with spikes and hydrophobic (17.1%). Aureobasidium pullulans was elliptical with longitudinal ridges and hydrophilic (79.9%). Following attachment assays, cPMMA attached the greatest numbers of conidia. Following the adhesion and retention assays (washing step included in the protocol), A. niger 1957 and A. niger 1988 were least adhered to the P(MMA-co-gMPS) surface, whilst A. pulluans was least adhered to the PMMAsc surface. This work demonstrated that in the absence of a washing step, only the surface properties influenced the conidia attachment, whilst in the presence of a washing step, both the properties of the surfaces and the conidia affected conidia adhesion and retention. Hence, the methodology used (with or without a washing step) should reflect the environment in which the surface is to be applied.
Citation: Kathryn A. Whitehead, Christopher M. Liauw, Joels S. T. Wilson-Nieuwenhuis, Anthony J. Slate, Ted Deisenroth, Andrea Preuss, Joanna Verran. The effect of the surface properties of poly(methyl methacrylate) on the attachment, adhesion and retention of fungal conidia[J]. AIMS Bioengineering, 2020, 7(3): 165-178. doi: 10.3934/bioeng.2020015
[1] | Frank R. Bengelsdorf, Christina Gabris, Lisa Michel, Manuel Zak, Marian Kazda . Syntrophic microbial communities on straw as biofilm carrier increase the methane yield of a biowaste-digesting biogas reactor. AIMS Bioengineering, 2015, 2(3): 264-276. doi: 10.3934/bioeng.2015.3.264 |
[2] | Ana Meireles, Ana L. Gonçalves, Inês B. Gomes, Lúcia Chaves Simões, Manuel Simões . Methods to study microbial adhesion on abiotic surfaces. AIMS Bioengineering, 2015, 2(4): 297-309. doi: 10.3934/bioeng.2015.4.297 |
[3] | Bennet Brockhagen, Jan Lukas Storck, Timo Grothe, Robin Böttjer, Andrea Ehrmann . Improved growth and harvesting of microalgae Chlorella vulgaris on textile fabrics as 2.5D substrates. AIMS Bioengineering, 2021, 8(1): 16-24. doi: 10.3934/bioeng.2021003 |
[4] | Olga M. Tsivileva, Inna M. Uchaeva, Nikolay A. Yurasov . Biotesting of technologically important carboxy containing acridones with solid-state fungal culture. AIMS Bioengineering, 2021, 8(1): 1-13. doi: 10.3934/bioeng.2021001 |
[5] | Amreeta Sarjit, Sin Mei Tan, Gary A. Dykes . Surface modification of materials to encourage beneficial biofilm formation. AIMS Bioengineering, 2015, 2(4): 404-422. doi: 10.3934/bioeng.2015.4.404 |
[6] | Ana Y. Rioja, Maritza Muniz-Maisonet, Thomas J. Koob, Nathan D. Gallant . Effect of nordihydroguaiaretic acid cross-linking on fibrillar collagen: in vitro evaluation of fibroblast adhesion strength and migration. AIMS Bioengineering, 2017, 4(2): 300-317. doi: 10.3934/bioeng.2017.2.300 |
[7] | Nikolaos Giormezis, Konstantinos Papakonstantinou, Fevronia Kolonitsiou, Eleanna Drougka, Antigoni Foka, Styliani Sarrou, Evangelos D. Anastassiou, Efthimia Petinaki, Iris Spiliopoulou . Biofilm synthesis and its relationship with genetic characteristics in clinical methicillin-resistant staphylococci. AIMS Bioengineering, 2015, 2(4): 375-386. doi: 10.3934/bioeng.2015.4.375 |
[8] | Sven Sölmann, Anke Rattenholl, Hannah Blattner, Guido Ehrmann, Frank Gudermann, Dirk Lütkemeyer, Andrea Ehrmann . Mammalian cell adhesion on different 3D printed polymers with varying sterilization methods and acidic treatment. AIMS Bioengineering, 2021, 8(1): 25-35. doi: 10.3934/bioeng.2021004 |
[9] | Daria Wehlage, Hannah Blattner, Al Mamun, Ines Kutzli, Elise Diestelhorst, Anke Rattenholl, Frank Gudermann, Dirk Lütkemeyer, Andrea Ehrmann . Cell growth on electrospun nanofiber mats from polyacrylonitrile (PAN) blends. AIMS Bioengineering, 2020, 7(1): 43-54. doi: 10.3934/bioeng.2020004 |
[10] | Zongyuan Zhu, Rachael Simister, Susannah Bird, Simon J. McQueen-Mason, Leonardo D. Gomez, Duncan J. Macquarrie . Microwave assisted acid and alkali pretreatment of Miscanthus biomass for biorefineries. AIMS Bioengineering, 2015, 2(4): 449-468. doi: 10.3934/bioeng.2015.4.449 |
Poly(methyl methacrylate) (PMMA) surfaces, (commercial PMMA (PMMAc), spin coated PMMA (PMMAsc) and a 90% methylmethacrylate/10% 3-methacryloxypropyltrimethoxysilane random copolymer (P(MMA-co-gMPS)) were used to determine the effect of surface properties on conidia biofouling. The contact angles of the substrates demonstrated that the PMMAsc and the P(MMA-co-gMPS) polymer (62.8°) were more wettable than the PMMAc surface (71.0°). The PMMAsc had the greatest roughness value (32.0 nm) followed by the PMMAc (3.0 nm), then P(MMA-co-gMPS) (1 nm). Aspergillus niger 1957 conidia were spherical, smooth and hydrophobic (12.1%). Aspergillus niger 1988 conidia were spherical with spikes and hydrophobic (17.1%). Aureobasidium pullulans was elliptical with longitudinal ridges and hydrophilic (79.9%). Following attachment assays, cPMMA attached the greatest numbers of conidia. Following the adhesion and retention assays (washing step included in the protocol), A. niger 1957 and A. niger 1988 were least adhered to the P(MMA-co-gMPS) surface, whilst A. pulluans was least adhered to the PMMAsc surface. This work demonstrated that in the absence of a washing step, only the surface properties influenced the conidia attachment, whilst in the presence of a washing step, both the properties of the surfaces and the conidia affected conidia adhesion and retention. Hence, the methodology used (with or without a washing step) should reflect the environment in which the surface is to be applied.
Poly (methyl methacrylate) (PMMA), is a transparent thermoplastic. Due to its characteristic properties such as being lightweight and transparent, it is often used as an alternative to glass [1]. The increased knowledge in the properties of PMMA has contributed to the enhanced interest in PMMA synthesis, modification, and applications [2].
A major issue in the application of polymeric materials is fungal degradation [3]. Due to concerns over the use of biocides in the environment [4], it is important to understand how the interactions of the surface properties and microbial characteristics can influence biofouling, so that surfaces with anti-adhesive properties can be developed, thus potentially reducing their likelihood of biodeterioration. This biodegradation process typically begins with the attachment, adherence, and then the retention of the cells onto the surface [5]. Once fungal spores attach to a polymeric surface, hydration events may result in the release of an extracellular matrix and growth of the microorganism [6]. Over time, the fungi can degrade polymers via a number of mechanisms including physical disruption, enzymatic activity and increased reduction of the surface plasticizers. Such degradation can result in damaged, weakened and unsightly materials [7]. Previously, it has been determined that surface properties, such as the topography [8], chemistry [9],[10] and physicochemistry [11] influenced initial cell or conidium attachment, adhesion and retention [12],[13].
Aspergillus spp. produces fungal spores, which are dispersed into the air and can remain in the atmosphere for prolonged periods of time. The degradation of polymers has been shown to be related to the production of various organic acids produced by Aspergillus spp. [14]. Furthermore, the adhesion, germination and subsequent growth of Aspergillus niger conidia may cause surface deterioration on different substrates and has been associated with colonization of contact lenses [15]. It is the initial attachment and germination of the conidia that leads to the subsequent hyphal invasion [16].
Aureobasidium pullulans is a ubiquitous saprophytic fungus that is often described as an environmental contaminant in temperate, tropical and polar areas, as it has exceptional tolerance to a broad range of ecological conditions [17]. A. pullans has been shown to colonise a range of habitats including common household products, including bathroom surfaces [18], dishwashers [19], tap water [20] and the surfaces of synthetic polymers and polyvinyl chloride (PVC) plastics [7],[21].
The fabrication method of the polymeric production will influence the substratum properties, this includes the surface topography and physicochemistry. Surfaces may be produced by manufacturing processes such as casting or spin coating, the latter allowing the chemical moieties of the substrata to be controlled. Often these properties are classified under the broader term of “responsive surfaces” [22].
An understanding of the interactions at the cell:substratum interface is essential to prevent biofouling [10]. The effect of the surface properties on the prevention of biofouling can be assessed by modifying surfaces or coatings with precisely controlled parameters. In order to better understand how different surface properties, affect the processes of spore attachment and retention to a range of polymeric surfaces, fungal conidia were used in a range of assays against three different surfaces, each with chemical variants of PMMA.
Spin coated samples were prepared by spin coating a polymer solution or mixture onto a silicon wafer (Montco, USA). Two spin coated surfaces were produced and included PMMA spin coated from solution (PMMAsc) and a 90% methylmethacrylate/10% 3-methacryloxy propyltrimethoxysilane (g-MPS) random copolymer P(MMA-co-gMPS). The desired polymer (or polymerising mixture) was dropped onto silicon wafer disks so that the entire disk was covered. Samples were centrifuged at 2000 rpm for 10–15 s.
The sessile drop technique method using contact angle measurements (KRÜSS GMBH, Germany) at room temperature was carried out to determine the wettability of the surface using five microliter volumes of HPLC grade water (BDH, UK). Five different surfaces were used and three replicate contact angles were taken from each sample (n = 5).
Roughness measurements were obtained using an Explorer AFM (Veeco Instruments, UK) in contact mode. The cantilever used had a spring constant of 0.05 N·m−1. In non-contact mode the cantilever spring constant was 50 N·m−1 (Veeco Instruments, UK). Samples were carried out in triplicate on different surfaces (n = 3).
Fungal cultures were re-suspended from freeze dried lyophilizates. The fungal pellet was transferred into a test tube and 3 mL NaCl-Triton X-100 (A. niger spp.) or Sabouraud broth (A. pullulans) (BDH, UK) was added. NaCl-Triton X-100 was made by suspending one millilitre of a v/v 1% solution in 100 mL sterile distilled water, into which 0.85 g sodium chloride (Sigma, UK) was dissolved. The suspension was vortexed for 5 min or until the pellet was dispersed. The fungal suspension was dispensed onto Sabouraud agar plates (BDH, UK). The plates were incubated for 3 to 21 days at 29 °C. Following growth of the fungi, a second transfer was made. Inoculated plates were incubated for 3–21 days at 29 °C. After growth of the fungi across the plate, a third transfer was made. This transfer was used to obtain the “working” cultures from which conidia were harvested.
Following fungal growth, 5 mL of a Sabouraud broth was pipetted onto the fungal culture. Conidia were removed from the culture by rubbing a sterile glass Pasteur pipette gently over the surface of the culture on the agar. The suspension was transferred into a sterile beaker containing a sterile magnetic stirrer. This process was repeated several times until all the visible sporulating growth was removed from the agar. The suspension was stirred for 30 min then filtered through a funnel filled with glass wool (VWR, UK) to obtain a homogeneous conidium suspension. Conidia were harvested at 1721 g for 10 min, washed three times in sterile distilled water, and re-suspended to an optical density (OD) of 1.0 ± 0.1 at 610 nm which equated to 6.53 ± 1.88 × 106 conidia cm2. Conidium suspensions were stored at 4 °C and used within two weeks and were checked using a light microscopy for purity prior to use.
The hydrophobicity of the conidia was measured using an adapted microbial adhesion to hexadecane method
Aliquots of 100 µL of conidium suspension was pipetted onto a 1 cm × 1 cm polished silicon wafer surface (Montco Silicon Technologies, US) and air dried in a class 2 microbiological containment hood. Surfaces were placed for 1 week in a desiccator containing phosphorous pentoxide (Sigma, UK). Substrata plus retained conidia were immersed in 4% v/v glutaraldehyde (Agar Ltd., UK) for 24 h at 4 °C. After fixing, substrata were washed gently with distilled water from a bottle at a 45° angle, with a 3 mm nozzle. Prior to examination, samples were stored at room temperature, in a desiccator containing phosphorous pentoxide. For SEM imaging, samples were fixed onto stubs for gold sputter coating (Polaron E5100, UK). Samples were coated at a vacuum of 0.09 mbar, for 3 min, at 2500 V, in argon gas at a power of 19 mA. Images of the substrata were obtained using a JEOL JSM 5600LV scanning electron microscope (n = 3).
To carry out the attachment assay, three replicate substrata (1 cm × 1 cm) were attached to a stainless steel tray using adhesive gum (Impega, Malaysia). The tray and attached substrata were placed vertically in a class 2 flow hood. The conidium suspension (adjusted to an of 1.0 ± 0.1 at 610 nm) was placed into the spray reservoir of a Badger Airbrush (Shesto, UK). The airbrush was propelled by a liquid gas canister (Esselte Letraset Ltd., UK). The airbrush was set to the finest spray setting, and at a distance of 10 cm the airbrush was passed left to right, ten times, at a speed of 50 mm·sec−1 over the substrata. The flow rate was 0.2 mL·sec−1 per pass. Immediately following spraying, the substrata were either laid horizontally and dried (attachment assay) or for the adhesion assay, the inoculated coupons were retained vertically and rinsed once, gently with 5 cm3 distilled H2O, with the distilled water bottle with a 3 mm nozzle at a 45° angle, to remove loosely attached conidia. Substrata with retained conidia were laid horizontally and air dried in a laminar flow hood. For the retention assay, three replicate substrata were placed horizontally in a sterile glass Petri dish. Twenty five milliliters of conidial suspension was added. The Petri dish containing the substrata was incubated without agitation for 1 h. The samples were removed, rinsed and air dried in a microbiological class 2 hood. The retained cells were stained for 2 min using 0.03% acridine orange in 2% glacial acetic acid (Sigma, UK), rinsed, and air dried. Substrata plus adherent conidia were visualised using epifluorescence microscopy (Nikon Eclipse E600, UK). The numbers of cells cm2 was determined via a total of 60 fields of view across three different substrata, for each different surface type (n = 3).
All results were presented as mean ± standard deviation (SD). Mean values were compared using student t-tests to determine significant differences at the 95% confidence level (p < 0.05).
The surface properties of the different PMMA substrata were determined in order to investigate their effects on conidia attachment, adhesion and retention.
The contact angles of the substrates demonstrated that there was no significant difference between the PMMAsc and the P(MMA-co-gMPS) polymer (62.8°) whereas the PMMAc surface was significantly different at 71.0° (Figure 1a).
All the PMMA surfaces tested demonstrated low surface roughness and had Ra values within the nanoscale range (1.0–32 nm). The spin coated PMMA sample (PMMAsc) had the greatest roughness value (32.0 ± 8.5 nm) followed by the PMMAc sheet (3.0 ± 0.3 nm) and then the P(MMA-co-gMPS) (1.0 nm ± 0.3 nm) (Figure 1b).
SEM images were taken of the conidia (Figure 2), it was evident that the morphology of the conidia varied dramatically. A. niger 1957 conidia were spherical (overall diameter: 2 to 3 µm), with a smooth surface. A. niger 1988 conidia were also spherical (overall diameter: 4 to 5 µm) but with spike-like projections on its surface. A. pullulans was ellipsoidal in shape with longitudinal ridges. The presence of dried extracellular polysaccharide substances (EPS) was evident in the SEM images of A. pullulans.
Fungal conidia hydrophobicity was carried out using hexadecane and water (Figure 3). The absorbance in the aqueous phase for the three different conidia after treatment with both solvents demonstrated that A. pullulans was significantly different from the A. niger 1957 and 1988 spores since it was predominantly hydrophilic as it resided in the aqueous phase (79.9%) (Figure 3). A. niger 1957 (12.1%) and A. niger 1988 (17.1%) conidia were also significantly different from one another, however, they demonstrated hydrophobicity, with A. niger 1957 being the most hydrophobic.
A range of microbiological assays were carried out to demonstrate how the conidia were retained on the surfaces under a range of conditions.
Following the attachment assay, A. niger 1957 conidia demonstrated significantly greater levels of attachment to all three surfaces than the other conidia types, the greatest amount of conidia was retained on the PMMAc substrata (A. niger 1957: 3.88 × 105 conidia cm−2, A. niger 1988: 7.62 × 104 conidia cm−2 and A. pullulans: 2.55 × 105 conidia cm−2) whilst the PMMAsc attached the least number of conidia overall (A. niger 1957: 9.34 × 104 conidia cm−2, A. niger 1988: 2.08 × 104 conidia cm−2 and A. pullulans: 3.78 × 104 conidia cm−2) (Figure 4).
For the A. niger 1957 conidia, the greatest numbers were adhered on the PMMAc and PMMAsc surfaces (4.78 × 103 conidia cm−2 and 7.12 × 103 conidia cm−2), respectively (Figure 5). The least number of conidia were adhered to the P(MMA-co-gMPS) surface (9.37 × 101 conidia cm−2).
The PMMAc surface demonstrated the greatest adherence for the A. niger 1988 (6.46 × 104 conidia cm−2) compared with the PMMAsc (1.78 × 103 conidia cm−2) and P(MMA-co-gMPS) surfaces (9.37 × 101 conidia cm−2).
Following adherence assays with A. pullulans, there was a significant difference in the number of conidia adhered to all three surface types, with the greatest conidia amount observed on the PMMAc (1.78 × 103 conidia cm−2), followed by the P(MMA-co-gMPS) surface (6.72 × 102 conidia cm−2), and with the least amount of conidia on the surface, PMMAsc (9.37 × 101 conidia cm−2). Overall, A. niger 1957 and A. niger 1988 were least adhered to the P(MMA-co-gMPS) surface, whilst A. pullulans was least attached to the PMMAsc surface.
The PMMAc surface retained the most conidia, with all the fungal conidia being retained in similar numbers (A. niger 1957: 1.87 × 104 conidia cm−2, A. niger 1988: 1.23 × 104 conidia cm−2 and A. pullulans: 1.54 × 104 conidia cm−2) (Figure 6). Only the A. niger 1957 conidia on the PMMAsc retained similar numbers of spores (1.80 × 104 conidia cm−2). Whilst, the PMMAsc retained A. niger 1988 (3.37 × 103 conidia cm−2) and A. pullulans (1.03 × 103 conidia cm−2) at a similar level. The P(MMA-co-gMPS) retained A. pullulans conidia in the greatest numbers (2.81 × 103 conidia cm−2) followed by A. niger 1957 (9.37 × 102 conidia cm−2) and then A. niger 1988 (3.75 × 102 conidia cm−2). Thus, following the retention assays, A. niger 1957 and A. niger 1988 conidia were retained in the lowest numbers on the P(MMA-co-gMPS), whilst A. pullulans conidia was retained in the least numbers on the PMMAsc surface.
PMMA is utilised in a plethora of industrial applications. This study aimed to determine if the differences in the surface properties of a range of PMMA based substrata influenced fungal conidia attachment, adhesion and retention. Contact angles using water were reported from the PMMA derivatives and all surfaces resulted in values between 0° and 90°, which demonstrated wettable substrata. The contact angle values of the c-PMMA, P(MMA-co-gMPS) and PMMAsc represented moderate hydrophilic attributes as would be expected from the structure of such polymers [24].
In order to determine the surface topography of the PMMA surfaces Ra values were reported. Although a difference within an order of 10 was observed, all the surfaces had Ra values of less than 33 nm. The lowest Ra value was demonstrated on the PMMAsc and was closely followed by the PMMAc substrata. During the fabrication process of PMMAc, the sheet is cast against glass sheets, therefore it was not surprising that the Ra value was low and in agreement with our results, PMMAsc has previously been shown to have a Ra value of <5 nm [25]. The increased roughness observed from the spin coated surface, P(MMA-co-gMPS) may be due to the more widely distributed chemical structures of the surfaces producing a greater Ra value [26].
It is important to understand the fundamental mechanisms that influence the binding of fungal spores to surfaces. This requires enhanced knowledge of the interactions between the conidia and the physicochemical, chemical and roughness properties of the surfaces. With regards to the surface physicochemistry affecting fungal spore binding to a surface, it has been shown that fungal spores may be strongly adhered to hydrophilic substrates [27]. In agreement with our study, previous work has demonstrated that pycnidiospores [28], Magnaporthe grisea conidia [29], and the conidia of Penicillium expansum [30] were found to be significantly more adherent to a non wettable surface. However, other studies have demonstrated that surface wettability did not play a major role in the adhesion of conidia [31].
Surface topography has been shown to influence the number bacterial spores that adhere to a surface [32]. However, Foschino et al. [33] demonstrated that the type of stainless steel finish (shot treated or not) had no significant effect on the cleanability of A. niger from the stainless steel. The results presented in this work demonstrated that the surface properties, including roughness did affect fungal conidia binding to the surfaces, but the results were dependant in part on the methodology used.
The affinity to solvent assay demonstrated a significant difference in the surface physicochemistry of the conidia hydrophobicity. Previous literature has shown that fungal conidia are highly hydrophobic [34]. The cell wall of A. niger is highly dynamic and contains several classes of polysaccharides, including, β-glucans, α-glucans, chitin, galactomannan, and cell wall mannoproteins [35],[36]. The cell morphology and therefore the conidium shape may therefore influence both attachment and adhesion. The spiny ornamentation may reduce the contact area of the conidia with smooth surfaces but may also increase the conidia numbers by interlocking on dynamic or responsive surfaces. The solvent assay confirmed that A. pullulans produced the most hydrophilic conidia. This is possibly due to the production of the polysaccharide, pullulan, as it has been suggested that it can form a hydrophilic, non-ionic surface layer that can reduce protein adhesion [37].
Following attachment, adhesion and retention assays it was demonstrated that the attachment assays generally retained most conidia whilst the adhesion assays resulted in the least attached conidia. This could be due to the conidia being unable to bind to the surface quickly enough, prior to the surface being washed.
Following the adhesion assays, A. niger 1957 conidia were generally retained in greater numbers than the A. niger 1988 conidia on the spin coated surfaces. This may be due to the varying spore morphology, as A. niger 1957 exhibited a rounder structure which can increase conidial attachment. The rougher morphology of the A. niger 1988 conidia, demonstrated a higher hydrophilicity and a spiny outer coat, the overall result being a lower surface contact and the lowest levels of adhesion/coverage observed.
Overall, this work demonstrated that the method of assay used is of paramount importance. Although it is possible to modify PMMA surfaces in order to control biodeterioration [38], this work aimed to look at PMMA surfaces that were chemically and structurally similar. In assays without a wash step, only the surface properties were found to influence conidia attachment, adhesion and retention; although it should be noted that all the surfaces were comparably smooth (1–32 nm). Following the assays, it was demonstrated that generally all three conidial types adhered in the greatest numbers to the PMMAc surfaces (which was the most hydrophobic and least rough surface), whilst all the three types of conidia were attached in the least numbers to the PMMAsc (the most hydrophilic and roughest surface). This may also be in part due to the chemistry of the surfaces. The PMMAsc is PMMA spin coated from solution (in tetrahydrofuran (THF)) onto a silicon wafer. The way in which this surface was fabricated could have resulted in branched chains of the polymer, which may have made the surface more dynamic which could potentially reduce conidia binding to the surfaces. In the attachment assay, the differences in the size, shape and chemistry of the conidia had no influence on microbial attachment, rather the results were driven by the surface properties.
In assays with a washing step (adherence and retention) the surface and the conidia properties influenced the results. Although as with the attachment assays, the PMMAc retained the most conidia, regardless of the species. Following the adhesion and retention assays, the A. niger spores were the least retained on the P(MMA-co-gMPS) surface, whilst the A. pullulans were least retained on the PMMAsc surface. This effect may be due to the rougher PMMAsc surface topography demonstrating a greater contact area between the conidia and the surface. This is in agreement with the findings of Nomura et al. [27] who demonstrated that the area between a spore and substrate depended on the substrate roughness. The results demonstrated throughout the different assays could potentially explain the variance in opinions regarding the influence of surface and microbial properties observed in the literature.
This work used three chemically similar polymers with different surface roughness and wettabilities to determine if the effect of the surface properties could be related to the amount of conidia attached. Three different types of binding assays, attachment, adhesion and retention were utilised. It was demonstrated that the least wettable surface generally retained the most conidia. This work further demonstrated that the method of assay used to assess conidia attachment is of paramount importance. In the attachment assays, only the surface properties were found to influence conidia retained. In assays with a washing step (adherence and retention) the properties of the surface and the conidia influenced the results observed. Thus, the assay used to assess the surface efficacy should reflect the microbial:surface interface of the applied environment.
[1] | Pawar E (2016) A review article on acrylic PMMA. IOSR J Mech Civil Eng 13: 1-4. |
[2] |
Ali U, Karim KJBA, Buang NA (2015) A review of the properties and applications of poly (methyl methacrylate) (PMMA). Polymer Rev 55: 678-705. doi: 10.1080/15583724.2015.1031377
![]() |
[3] |
John MJ (2017) Environmental degradation in biocomposites. Biocomposites for High-Performance Applications. Current Barriers and Future Needs Towards Industrial Development Woodhead Publishing, 181-194. doi: 10.1016/B978-0-08-100793-8.00007-7
![]() |
[4] |
Bell GM, Chadwick J (1994) Regulatory controls on biocides in the United Kingdom and restrictions on the use of triorganotin-containing antifouling products. Int Biodeter Biodegr 34: 375-386. doi: 10.1016/0964-8305(94)90095-7
![]() |
[5] |
Rajab FH, Liauw CM, Benson PS, et al. (2018) Picosecond laser treatment production of hierarchical structured stainless steel to reduce bacterial fouling. Food Bioprod Process 109: 29-40. doi: 10.1016/j.fbp.2018.02.009
![]() |
[6] |
Chaky J, Anderson K, Moss M, et al. (2001) Surface hydrophobicity and surface rigidity induce spore germination in Colletotrichum graminicola. Phytopathology 91: 558-564. doi: 10.1094/PHYTO.2001.91.6.558
![]() |
[7] |
Cappitelli F, Sorlini C (2008) Microorganisms attack synthetic polymers in items representing our cultural heritage. Appl Environ Microbiol 74: 564-569. doi: 10.1128/AEM.01768-07
![]() |
[8] |
Whitehead KA, Colligon J, Verran J (2005) Retention of microbial cells in substratum surface features of micrometer and sub-micrometer dimensions. Coll Surf B: Biointerfaces 41: 129-138. doi: 10.1016/j.colsurfb.2004.11.010
![]() |
[9] |
Ma H, Winslow CJ, Logan BE (2008) Spectral force analysis using atomic force microscopy reveals the importance of surface heterogeneity in bacterial and colloid adhesion to engineered surfaces. Coll Surf B: Biointerfaces 62: 232-237. doi: 10.1016/j.colsurfb.2007.10.007
![]() |
[10] |
Whitehead KA, Deisenroth T, Preuss A, et al. (2011) The effect of surface properties on the strength of attachment of fungal spores using AFM perpendicular force measurements. Coll Surf B: Biointerfaces 82: 483-489. doi: 10.1016/j.colsurfb.2010.10.001
![]() |
[11] |
Kipanga PN, Luyten W (2017) Influence of serum and polystyrene plate type on stability of Candida albicans biofilms. J Microbiol Meth 139: 8-11. doi: 10.1016/j.mimet.2017.04.006
![]() |
[12] |
Bigerelle M, Anselme K, Dufresne E, et al. (2002) An unscaled parameter to measure the order of surfaces: a new surface elaboration to increase cells adhesion. Biomol Eng 19: 79-83. doi: 10.1016/S1389-0344(02)00048-5
![]() |
[13] |
Beauvais A, Schmidt C, Guadagnini S, et al. (2007) An extracellular matrix glues together the aerial-grown hyphae of Aspergillus fumigatus. Cell Microbiol 9: 1588-1600. doi: 10.1111/j.1462-5822.2007.00895.x
![]() |
[14] |
Ramage G, Rajendran R, Gutierrez-Correa M, et al. (2011) Aspergillus biofilms: clinical and industrial significance. FEMS Microbiol Lett 324: 89-97. doi: 10.1111/j.1574-6968.2011.02381.x
![]() |
[15] |
Marques-Calvo MS (2002) In vitro colonization of hydrophilic contact lenses by Aspergillus niger. J Ind Microbiol Biotech 29: 6-9. doi: 10.1038/sj.jim.7000255
![]() |
[16] |
Filler SG, Sheppard DC (2006) Fungal invasion of normally non-phagocytic host cells. PLOS Pathog 2: e129. doi: 10.1371/journal.ppat.0020129
![]() |
[17] |
Gostinčar C, Ohm RA, Kogej T, et al. (2014) Genome sequencing of four Aureobasidium pullulans varieties: biotechnological potential, stress tolerance, and description of new species. BMC Genomics 15: 549. doi: 10.1186/1471-2164-15-549
![]() |
[18] | Lotrakul P, Deenarn P, Prasongsuk S, et al. (2009) Isolation of Aureobasidium pullulans from bathroom surfaces and their antifungal activity against some Aspergilli. Afr J Microbiol Res 3: 253-257. |
[19] |
Zalar P, Novak M, De Hoog GS, et al. (2011) Dishwashers—a man-made ecological niche accommodating human opportunistic fungal pathogens. Fungal Biol 115: 997-1007. doi: 10.1016/j.funbio.2011.04.007
![]() |
[20] |
Arvanitidou M, Kanellou K, Constantinides TC, et al. (1999) The occurrence of fungi in hospital and community potable waters. Lett Appl Microbiol 29: 81-84. doi: 10.1046/j.1365-2672.1999.00583.x
![]() |
[21] |
Shah AA, Hasan F, Hameed A, et al. (2008) Biological degradation of plastics: a comprehensive review. Biotech Adv 26: 246-265. doi: 10.1016/j.biotechadv.2007.12.005
![]() |
[22] |
Stuart MAC, Huck WTS, Genzer J, et al. (2010) Emerging applications of stimuli-responsive polymer materials. Nat Mat 9: 101-113. doi: 10.1038/nmat2614
![]() |
[23] |
Rosenberg M, Kjellerberg S (1986) Hydrophobic interactions: role in bacterial adhesion. Advances in Microbial Ecology Boston: Springer, 353-393. doi: 10.1007/978-1-4757-0611-6_8
![]() |
[24] |
Rezaei F, Abbasi-Firouzjah M, Shokri B (2014) Investigation of antibacterial and wettability behaviours of plasma-modified PMMA films for application in ophthalmology. J Phys D: Appl Phys 47: 085401. doi: 10.1088/0022-3727/47/8/085401
![]() |
[25] |
Sabia R, Stevens HJ, Varner JR (1999) Pitting of a glass-ceramic during polishing with cerium oxide. J Non-Cryst Solids 249: 123-130. doi: 10.1016/S0022-3093(99)00316-6
![]() |
[26] |
Söz CK, Yilgör E, Yilgör I (2015) Influence of the average surface roughness on the formation of superhydrophobic polymer surfaces through spin-coating with hydrophobic fumed silica. Polymer 62: 118-128. doi: 10.1016/j.polymer.2015.02.032
![]() |
[27] |
Nomura T, Minamiura M, Fukamachi K, et al. (2018) Adhesion control of fungal spores on solid surfaces using hydrophilic nanoparticles. Adv Powder Technol 29: 909-914. doi: 10.1016/j.apt.2018.01.007
![]() |
[28] |
Kuo KC, Hoch HC (1996) Germination of Phyllosticta ampelicida Pycnidiospores: Prerequisite of adhesion to the substratum and the relationship of substratum wettability. Fungal Genet Biol 20: 18-29. doi: 10.1006/fgbi.1996.0005
![]() |
[29] |
Hamer JE, Howard RJ, Chumley FG, et al. (1988) A mechanism for surface attachment in spores of a plant pathogenic fungus. Science 239: 288-290. doi: 10.1126/science.239.4837.288
![]() |
[30] |
Amiri A, Cholodowski D, Bompeix G (2005) Adhesion and germination of waterborne and airborne conidia of Penicillium expansum to apple and inert surfaces. Phys Mol Plant Pathol 67: 40-48. doi: 10.1016/j.pmpp.2005.07.003
![]() |
[31] |
Sammonds J, Jaspers MV, Jones EE (2016) Pre-infection processes of Botryosphaeriaceae spp.: adhesion of conidia to different substrata. Plant Pathol 65: 1142-1152. doi: 10.1111/ppa.12485
![]() |
[32] |
Faille C, Jullien C, Fontaine F, et al. (2002) Adhesion of Bacillus spores and Escherichia coli cells to inert surfaces: role of surface hydrophobicity. Can J Microbiol 48: 728-738. doi: 10.1139/w02-063
![]() |
[33] |
Foschino R, Picozzi C, Civardi A, et al. (2003) Comparison of surface sampling methods and cleanability assessment of stainless steel surfaces subjected or not to shot peening. J Food Eng 60: 375-381. doi: 10.1016/S0260-8774(03)00060-8
![]() |
[34] |
Luke B, Faull J, Bateman R (2015) Using particle size analysis to determine the hydrophobicity and suspension of fungal conidia with particular relevance to formulation of biopesticide. Biocontrol Sci Technol 25: 383-398. doi: 10.1080/09583157.2014.979396
![]() |
[35] |
Klis FM, Ram AFJ, De Groot PWJ (2007) A molecular and genomic view of the fungal cell wall. Biology of the fungal cell Heidelberg: Springer, 97-120. doi: 10.1007/978-3-540-70618-2_4
![]() |
[36] |
Temple MJ, Cuskin F, Baslé A, et al. (2017) A Bacteroidetes locus dedicated to fungal 1, 6-β-glucan degradation: unique substrate conformation drives specificity of the key endo-1, 6-β-glucanase. J Biol Chem 292: 10639-10650. doi: 10.1074/jbc.M117.787606
![]() |
[37] | Rekha MR, Sharma CP (2007) Pullulan as a promising biomaterial for biomedical applications: a perspective. Trends Biomater Artif Organs 20: 116-121. |
[38] |
Prashantha KVH, Lakshmanb K, Shamalab TR, et al. (2005) Biodegradation of chitosan-graft-polymethylmethacrylate films. Int Biodeter Biodegr 56: 115-120. doi: 10.1016/j.ibiod.2005.06.007
![]() |
1. | Mohsin Amin, Andrea Preuss, Ted Deisenroth, Christopher M. Liauw, Joanna Verran, Kathryn A. Whitehead, Use of spherical particles to understand conidial attachment to surfaces using atomic force microscopy, 2021, 24, 25890042, 101962, 10.1016/j.isci.2020.101962 | |
2. | I. Devine Akhidime, Anthony J. Slate, Anca Hulme, Kathryn A. Whitehead, The Influence of Surface Topography and Wettability on Escherichia coli Removal from Polymeric Materials in the Presence of a Blood Conditioning Film, 2020, 17, 1660-4601, 7368, 10.3390/ijerph17207368 | |
3. | Kathryn A. Whitehead, Christopher M. Liauw, Stephen Lynch, Mohamed El Mohtadi, Mohsin Amin, Andrea Preuss, Ted Deisenroth, Joanna Verran, Diverse surface properties reveal that substratum roughness affects fungal spore binding, 2021, 24, 25890042, 102333, 10.1016/j.isci.2021.102333 | |
4. | Jake McClements, Luciana C. Gomes, Joshua Spall, Fabien Saubade, Devine Akhidime, Marloes Peeters, Filipe J. Mergulhão, Kathryn A. Whitehead, Drawing inspiration from nature to develop anti-fouling coatings: the development of biomimetic polymer surfaces and their effect on bacterial fouling, 2021, 93, 0033-4545, 1097, 10.1515/pac-2021-0108 | |
5. | Kathryn A. Whitehead, Ted Deisenroth, Andrea Preuss, Christopher M. Liauw, Joanna Verran, Lateral force removal of fungal spores to demonstrate how surface properties affect fungal spore retention, 2022, 380, 1364-503X, 10.1098/rsta.2021.0344 | |
6. | Liam A. Crawford, Valentina Cuzzucoli Crucitti, Amy Stimpson, Chloe Morgan, Jonathan Blake, Ricky D. Wildman, Andrew L. Hook, Morgan R. Alexander, Derek J. Irvine, Simon V. Avery, A potential alternative to fungicides using actives-free (meth)acrylate polymers for protection of wheat crops from fungal attachment and infection, 2023, 25, 1463-9262, 8558, 10.1039/D3GC01911J | |
7. | Kathryn A. Whitehead, Stephen Lynch, Mohsin Amin, Ted Deisenroth, Christopher M. Liauw, Joanna Verran, Effects of Cationic and Anionic Surfaces on the Perpendicular and Lateral Forces and Binding of Aspergillus niger Conidia, 2023, 13, 2079-4991, 2932, 10.3390/nano13222932 |