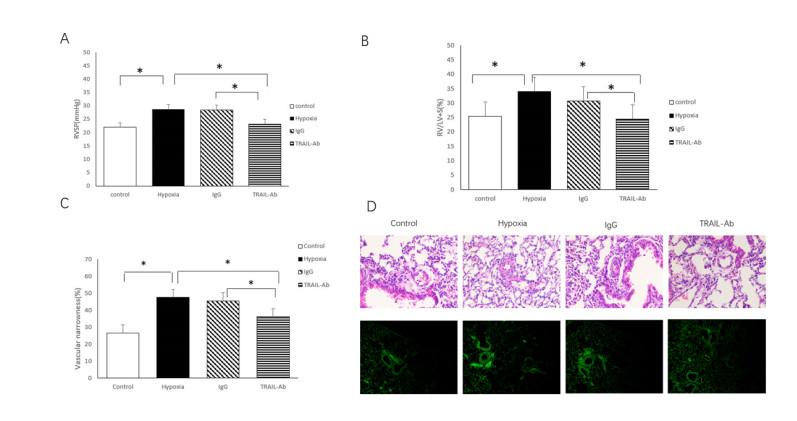
Citation: Erli Yang, Xiaobei Zhang, Qiangsheng Chen, Chandong Ding. Tumor necrosis factor-related apoptosis-inducing ligand regulate the accumulation of extracelluar matrix in pulmonary artery by activating the phosphorylation of Smad2/3[J]. Mathematical Biosciences and Engineering, 2020, 17(2): 1372-1380. doi: 10.3934/mbe.2020069
[1] | Christian Winkel, Simon Neumann, Christina Surulescu, Peter Scheurich . A minimal mathematical model for the initial molecular interactions of death receptor signalling. Mathematical Biosciences and Engineering, 2012, 9(3): 663-683. doi: 10.3934/mbe.2012.9.663 |
[2] | Xiao-Wei Ye, Xiao-Peng Zhang, Feng Liu . CSB modulates the competition between HIF-1 and p53 upon hypoxia. Mathematical Biosciences and Engineering, 2019, 16(5): 5274-5262. doi: 10.3934/mbe.2019262 |
[3] | Ming Zhang, Yingying Zhou, Yanli Zhang . High Expression of TLR2 in the serum of patients with tuberculosis and lung cancer, and can promote the progression of lung cancer. Mathematical Biosciences and Engineering, 2020, 17(3): 1959-1972. doi: 10.3934/mbe.2020104 |
[4] | Donggu Lee, Sunju Oh, Sean Lawler, Yangjin Kim . Bistable dynamics of TAN-NK cells in tumor growth and control of radiotherapy-induced neutropenia in lung cancer treatment. Mathematical Biosciences and Engineering, 2025, 22(4): 744-809. doi: 10.3934/mbe.2025028 |
[5] | Wei Huo, Xiao-Min Zhu, Xin-Yan Pan, Min Du, Zhuo Sun, Zhi-Min Li . MicroRNA-527 inhibits TGF-β/SMAD induced epithelial-mesenchymal transition via downregulating SULF2 expression in non-small-cell lung cancer. Mathematical Biosciences and Engineering, 2019, 16(5): 4607-4621. doi: 10.3934/mbe.2019231 |
[6] | Hao Shen, Xiao-Dong Weng, Du Yang, Lei Wang, Xiu-Heng Liu . Long noncoding RNA MIR22HG is down-regulated in prostate cancer. Mathematical Biosciences and Engineering, 2020, 17(2): 1776-1786. doi: 10.3934/mbe.2020093 |
[7] | Elena Izquierdo-Kulich, Margarita Amigó de Quesada, Carlos Manuel Pérez-Amor, Magda Lopes Texeira, José Manuel Nieto-Villar . The dynamics of tumor growth and cells pattern morphology. Mathematical Biosciences and Engineering, 2009, 6(3): 547-559. doi: 10.3934/mbe.2009.6.547 |
[8] | Alexis B. Cook, Daniel R. Ziazadeh, Jianfeng Lu, Trachette L. Jackson . An integrated cellular and sub-cellular model of cancer chemotherapy and therapies that target cell survival. Mathematical Biosciences and Engineering, 2015, 12(6): 1219-1235. doi: 10.3934/mbe.2015.12.1219 |
[9] | Elena Izquierdo-Kulich, Margarita Amigó de Quesada, Carlos Manuel Pérez-Amor, José Manuel Nieto-Villar . Morphogenesis and aggressiveness of cervix carcinoma. Mathematical Biosciences and Engineering, 2011, 8(4): 987-997. doi: 10.3934/mbe.2011.8.987 |
[10] | Avner Friedman, Edward M. Lungu . Can malaria parasite pathogenesis be prevented by treatment with tumor necrosis factor-alpha?. Mathematical Biosciences and Engineering, 2013, 10(3): 609-624. doi: 10.3934/mbe.2013.10.609 |
Pulmonary hypertension (PH) is a progressive, refractory and extremely malignant vascular disease, which is characterized by small pulmonary arterial stenosis and obstruction induced by vascular remodeling. As we know, this disease ultimately leads to an elevation of pulmonary artery pressure (PAP), right heart failure and finally death [1].
Pathologically, PH is characterized by pulmonary vasoconstriction, vascular remodeling, thrombosis and inflammation [2,3]. Numerous evidences demonstrate that vascular remodeling, which was observed as accumulation of extracelluar matrix (ECM), proliferation and hypertrophy endothelial cell (EC), smooth muscle cell (SMC) and adventitial fibroblast (VAF), plays a central pathological role in the progress and prognosis of PH [4,5,6]. The ECM is mainly composed of collagen, fibronectin, tenascin and elastin produced by the vascular wall cells especially pulmonary artery smooth muscle cells (PASMCs) [7].
TNF is a cytokine that induces apoptosis and that the TNF receptor family has plenty of molecules involved in the regulation of apoptosis [8,9]. Tumor necrosis factor-related apoptosis-inducing ligand (TRAIL) is a member of the tumor necrosis factor (TNF) family of cytokines, abundantly expressed in many tissues such as lung [10]. It was reported that TRAIL protein and mRNA were significantly expressed in airway wall structural cells, including fibroblasts, endothelial cells and mainly located in the smooth muscle cells of the pulmonary artery and aorta [11,12]. The expressions of TRAIL protein and its receptors, especially R3, obviously increased in pulmonary smooth muscle cells of PH patients compared to control group [13]. There is evidence that the proliferation and migration of SMCs in vitro are TRAIL-R3-dependent [14,15]. In recent years, the role of TRAIL in PH fields has been studied gradually. We have previously demonstrated that human and rodents serum TRAIL concentrations are positively related to the severity of PH [16]. Hameed AG [17] has previously demonstrated that TRAIL-KO and administration of TRAIL inhibitors can obstruct the development of PH in mammals by reducing the expression and deposition of ECM. The activation of transforming growth factor-β (TGF-β) and BMP signaling pathways play major roles in the expression of ECM [12,13,18].
However, up till now, there is no report on which signal pathway TRAIL participates in the expression and deposition of ECM in PH. Whether TRAIL is involved in PH pathological process by affecting ECM with activation of BMP or TGF-β signaling pathways remains unknown. In our study, we hope to explore the role of TRAIL in ECM deposition and its downstream signaling pathway.
C57/BL6 WT male mice (6-8 weeks old, purchased from Jinan Pengyue Experimental Animal Co., Ltd. (Jinan, Shandong Province, China)) were stratified into four groups: Control group (n = 5), hypoxia-induced PH mice group (n = 8), TRAIL-Ab treatment group (n = 8) and IgG group (n = 8). Control group: The mice were exposed to normoxia; hypoxia-induced PH mice group: Exposed to hypoxia (10% oxygen and 90% nitrogen) for 3 weeks; TRAIL-Ab and IgG group: TRAIL-Ab (Catalog: AF1121, R & D Systems, Minnesota, USA) and IgG antibody (Catalog: IC108P, R & D Systems, Minnesota, USA) were delivered at 0.8 ng/g/h for 4 weeks after hypoxia using osmotic mini-pumps for 3 weeks.
Four weeks after TRAIL-Ab and IgG antibody pumping, all mice were anesthetized with 10% chloral hydrate (3 mL/kg). Right ventricular catheterization was performed via the right external jugular vein as previously described. Right ventricular systolic pressure (RVSP) was measured by a Power-Lab data acquisition system (AD Instruments Shanghai Trading Co.). The hearts were rapidly separated after the measurement of RVSP. Segregating the left ventricle (LV), right ventricle (RV) and ventricular septum (S) with shears then weighing them to assess RVH. The index of RVH was determined by the ratio of weight of the RV to the LV plus S [RV/(LV+S)].
The lungs were immediately taken out after the measurement of RVSP. The lung were washed with ice saline then perfused with 4% paraformaldehyde, embedded in paraffin, sectioned (5 μm in thickness), and stained with hematoxylin and eosin for observing the vascular remodeling, which was calculated by the following formula: Outer diameter-lumen diameter/outer diameter. Ten-fifteen vessels with a diameter of 30-80 μm in each group were randomly selected for statistical analysis. Lung tissue sections were deparaffinized antigen retrieval. Tissue sections (5 μm) were blocked by diluted normal serum firstly. Then were incubated with anti-α-SMA (Catalog: 19245; Cell Signmling Technology, Danvers, USA) followed by Alexa Fluor 488-conjugated secondary goat anti-rabbit IgG H & L (Catalog: Ab150077; ABCAM, Cambridge, USA). The sections were sealed with anti-quenching agent.
The PASMCs (GrowGn Biotechnology Co., Ltd.) in the experiment were cultured with F12K medium under normoxia (95% O2, 5% CO2) or hypoxia (1% O2, 5% CO2, 94% N2) for 24 hours. TRAIL-ab was added to cells during hypoxic treatment.
Plasma TRAIL was measured in duplicate by specific, commercially available ELISA kit (R & D Systems, Minneapolis, MN, USA), in accordance with the manufacturer’s instructions and analyzed with an ELISA reader at 450 nm.
Cracking on ice for 30 min after adding cell lysate and PMSF (phenylmethanesulfonyl fluoride) to PASMCs or isolated lungs from mice. Then the liquid were centrifuged at 12000 rpm for 30 minutes at 4 ℃. The supernate was taken as protein samples. Different concentrations of SDS-PAGE gels were prepared according to the molecular weight of target protein for each equal amounts of sample protein (50 μg) then transferred to PVDF membranes. The membranes were blocked for 1 h with a buffer containing 5% skim milk powder before incubated with the following primary antibodies: Collagen Ⅰ (Catalog: Ab21286; ABCAM, Cambridge, USA), Smad2/3 (Catalog: 5678; Cell Signmling Technology, Danvers, USA), p-Smad2/3 (Catalog: Sab4504208; Sigma), Smad1/5/8 (21684;SAB), p-Smad1/5/8 (AB3848-1; millipore) over night at 4 ℃. Then the membranes were incubated with goat anti-rabbit IgG (Catalog: Ab6721; ABCAM, Cambridge, USA). Proteins were performed by chemiluminescence with an ECL kit. We usually quantize the grayscale of Western Blotting figures using the software ImageJ.
Continuous data were summarized as mean ± SEM. T-test was used for analysis of two sets of quantitative data while one-way analysis of variance (ANOVA) was used for more than two sets of quantitative data. A P-value of < 0.05 was deemed statistically significant (Graphpad 5, San Diego, CA, USA; SPSS, version 16.0; SPSS inc: Chicago, IL, USA).
In our previous study, the level of TRAIL was increased in PH patients and hypoxia-indcued PH mice [18]. Mice in experiment were divided into four groups: Control, hypoxia-induced PH mice, TRAIL-Ab and IgG groups. Hypoxia-induced PH mice had significant increase in RVSP (29.48 ± 1.42 versus 21.97 ± 1.29 mmhg, P < 0.05), RVH (34.02 ± 0.26% versus 25.46 ± 0.68%, P < 0.05), vascular stenosis (47.56 ± 3.34% versus 26.56 ± 3.42%, P < 0.05) compared to controls. Mice with anti-TRAIL antibody had lower RVSP (23.11 ± 0.84 vs. 29.48 ± 1.42 mmhg, P < 0.05), RVH (24.45 ± 0.29% versus 34.02 ± 0.26%, P < 0.05), vascular stenosis (36.21 ± 5.21 versus 47.56 ± 3.34%, P < 0.05) than PH mice, whereas IgG group had no significant improvement in hemodynamics and morphology (Figure 1).
Vasoconstriction, thrombosis and vascular remodeling are key components in the pathogenesis of PH. Synthesis and deposition of ECM play an important role in vascular remodeling. We found that collagen (a component of the ECM) increased significantly in pulmonary artery after hypoxia treatment, and reduced after treatment of TRAIL-Ab (without expression in the article). In vitro experiments, we also found that TRAIL-Ab inhibit the expression of collagen (Figure 2).
So far, many studies have shown that TRAIL plays an important role in PH. Hameed AG [17] have demonstrated that TRAIL block the development of PH by reducing the expression and deposition of ECM. All we know that TGF-β and BMP signaling pathway participate in the expression of ECM. In our experiment, we confirmed that TRAIL-Ab inhibited the expression of ECM in hypoxic-PH mice and PSMC. So we hope to do further research on whether TRAIL interfere with PH through TGF-β and BMP pathway or not. After 24 hours of hypoxic treatment in PASMC, we found that the TRAIL-Ab significantly inhibited the phosphorylation of Smad2/3, which is the downstream signaling of TGF-β (Figure 2).
In this experiment, we observed a significant decrease in RVSP and a decline in the expression of ECM during hypoxia-induced PH mice with the intervention of TRAIL-Ab. The pSmad2/3 proteins were highly expressive in the cultured hypoxia-stimulated PASMCs. Addition of 100 ng/ml TRAIL-Ab resulted in a significant decline in the expression of pSmad2/3.
TRAIL, one member of the tumor necrosis factor (TNF) superfamily [19] is known as Apo-2 ligand (Apo-2L)/TNFSF10 [20]. TRAIL is a type Ⅱ transmembrane protein with extracellular C-terminal domain which can hydrolyze from the cell surface and transform into a soluble cytokine [21]. TRAIL interacts with the four transmembrane receptors TRAIL-R1, R2, R3, R4 and a soluble receptor OPG [22]. TRAIL-R1 (DR4 or TNFRSF10A) and TRAIL-R2 (DR5 or TNFRSF10B) contain cell death domains and induce apoptosis by interaction with FADD via binding caspase-8 and/or caspase-10 to their death domains [23]. Conversely, TRAIL-R3 (DcR1) and TRAIL-R4 (DcR2) are considered as decoy receptors to protect normal cells from apoptosis for lack of the intracellular death domain and the apoptosis-inducing ability [24,25]. OPG inhibits TRAIL-mediated apoptosis by competing with the binding of TRAIL-R1 and TRAIL-R2 to TRAIL [26]. In our study, we found that collagen (a component of the ECM) increased significantly in pulmonary artery after hypoxia treatment, and reduced after treatment of TRAIL-Ab, which means TRAIL influence vascular remodeling by regulating the expression of ECM. Similarly, Hassoun [5] demonstrated that ECM, with deposition of collagen and elastin, contributing to the remodeling of the adventitia. Besides, matrix metalloproteinases (MMP), a kind of proteolytic enzymes, play an important role in collagen degradation and the regulation of ECM.
Due to the role of pro-apoptotic there are many researches in oncology over the past two decades [18,27]. Previous researches demonstrated that TRAIL can induce apoptosis in malignant cells [21]. It has recently been reported that TRAIL also plays an important role in various aspects such as inflammation and immune regulation. Importantly, TRAIL abundantly expressed in vascular smooth muscle cells and can be induced to promote apoptosis by autocrine or paracrine in diabetes, hyperlipidemia, chronic renal failure and atherosclerosis [12]. There is evidence that TRAIL considerably promotes the generation of atherosclerotic plaque by inducing apoptosis of macrophages and increasing the proliferation of VSMCs [28]. In addition, the expression of TRAIL and its receptors have previously been described in abdominal aortic aneurysm and promote the calcification of its vascular wall [29].
Angiogenesis, which includes the instability of blood vessels, the degradation of ECM, and the proliferation and migration of EC and SMC [30] can be stimulated by pro-angiogenic cytokines (VEGF, bFGF, PDGF), pleiotropic cytokines such as TGF-β and chemokines (MIP) [31]. Recent work has demonstrated that TRAIL is closely related to these cytokines. It was observed that the TGF-β1 and BMPs downregulated TRAIL mRNA [11]. TGF-β plays a key role in the pathogenesis of pulmonary fibrosis and vascular remodeling. TGF-β promotes the synthesis of collagens and fibronectin by down-regulated MMPs and up-regulates TIMPs, results in fibroblast transdifferentiation and excessive ECM accumulation [32,33,34]. In addition, some researchers pointed out that TGF-β1 gene polymorphisms were associated with PAH [35]. BMP pathway plays a major role in the regulation of tissue homeostasis and vasculogenesis which including cell migration and proliferation as well as ECs and SMCs apoptosis [36,37]. Activated TGF-β and BMP signaling pathways act on their phosphorylated receptors respectively located in the cell membrane, then exert phosphorylation on the downstream Smad2/3 and Smad1/5/8, affecting the transcription of target gene and the expression of target protein after entering into the nucleus under the synergistic effect of Smad4 [38,39].
Hameed AG [17] has found that anti-TRAIL antibody resulted in the normalization of RVSP and improvement of pulmonary artery remodeling. In our study we demonstrated that anti-TRAIL antibody induced significant improvement of disease in mice with chronic hypoxia-indcued PH and suppressed hypoxia-stimulated ECM protein expression by interfering with Smad2/3 signaling.
One limitation of the mice studies was that the pathological characteristics of hypoxic-induced PH animal models could not represent all types of pulmonary hypertension. Furthermore, TRAIL is important in inducing apoptosis in a variety of tumors, using TRAIL inhibitors may increase the risk of lung cancer and respiratory infections [40]. Regardless of the fact that there are several limitations of our issue, substantial evidence shows that TRAIL is a key driver in the pulmonary vascular remodeling.
The median survival of patients with PH especially idiopathic pulmonary arterial hypertension (IPAH) are less than three years without treatment [41]. Current treatments have failed to significantly improve the prognosis. Considering the poor prognosis and limited treatment of PH, early effective therapy is very important. Although many deficiency of TRAIL, our study further demonstrated that TRAIL plays an essential pathogenic roles in hypoxia-induced PH mice model and provides a new idea to explore effective treatment of PH.
This work was funded by Scientific Research Fund of Anhui Medical University (NO. 2017xkj029).
The authors declare there is no conflict of interest.
[1] | A. B. Waxman and R. T. Zamanian, Pulmonary arterial hypertension: New insights into the optimal role of current and emerging prostacyclin therapies, Am. J. Cardiol., 111 (2013), 1A-16A. |
[2] | L. J. Rubin, Primary pulmonary hypertension, N. Engl. J. Med., 336 (1997), 111-117. |
[3] | J. R. Runo and J. E. Loyd, Primary pulmonary hypertension, Lancet, 361 (2003), 1533-1544. |
[4] | J. Hänze, N. Weissmann, F. Grimminger, et al., Cellular and molecular mechanisms of hypoxia inducible factor driven vascular remodeling, Thromb. Haemostasis, 97 (2007), 774-787. |
[5] | P. M. Hassoun, Deciphering the "matrix" in pulmonary vascular remodeling, Eur. Respir. J., 25 (2005), 778-779. |
[6] | E. Arciniegas, M. G. Frid, I. S. Douglas, et al., Perspectives on endothelial to mesenchymal transition: Potential contribution to vascular remodeling in chronic pulmonary hypertension, Am. J. Physiol. Lung Cell. Mol. Physiol., 293 (2007), L1-L8. |
[7] |
G. Burgstaller, B. Oehrle, M. Gerckens, et al., The instructive extracellular matrix of the lung: Basic composition and alterations in chronic lung disease, Eur. Respir. J., 50 (2017), 1601805. doi: 10.1183/13993003.01805-2016
![]() |
[8] | V. Jurisic, T. Srdic-Rajic, G. Konjevic, et al., TNF-α induced apoptosis is accompanied with rapid CD30 and slower CD45 shedding from K-562 cells, J. Membr. Biol., 293 (2011), 115-122. |
[9] | V. Jurisic, T. Terzic, S. Colic, et al., The concentration of TNF-α correlate with number of inflammatory cells and degree of vascularization in radicular cysts, Oral Dis., 14 (2008), 600-605. |
[10] | A. Lawrie, A. G. Hameed, J. Chamberlain, et al., Paigen diet-fed apolipoprotein E knockout mice develop severe pulmonary hypertension in an interleukin-1-dependent manner, Am. J. Pathol., 179 (2011), 1693-1705. |
[11] | N. M. Robertson, M. Rosemiller, R. G. Lindemeyer, et al., TRAIL in the Airways, Vitam. Horm., 67 (2004), 149-167. |
[12] |
B. R. Gochuico, J. Zhang, B. Y. Ma, et al., TRAIL expression in vascular smooth muscle, Am. J. Physiol. Lung Cell. Mol. Physiol., 278 (2000), L1045-L1050. doi: 10.1152/ajplung.2000.278.5.L1045
![]() |
[13] | A. Lawrie, E. Waterman, M. Southwood, et al., Evidence of a role for osteoprotrgerin in the pathogenesis of pulmonary arterial hypertension, Am. J. Pathol., 172 (2008), 256-264. |
[14] | X. D. Zhang, T. Nguyen, W. D. Thomas, et al., Mechanisms of resistance of normal cells to TRAIL induced apoptosis vary between different cell types, FEBS Lett., 482 (2000), 193-199. |
[15] |
R. Di Pietro, M. A. Mariggio, S. Guarnieri, et al., Tumor Necrosis Factor-Related Apoptosis-Inducing Ligand (TRAIL) Regulates Endothelial Nitric Oxide Synthase (eNOS) Activity and Its Localization Within the Human Vein Endothelial Cells (HUVEC) in Culture, J. Cell. Biochem., 97 (2006), 782-794. doi: 10.1002/jcb.20686
![]() |
[16] | H. Liu, E. Yang, X. Lu, et al., Serum levels of tumor necrosis factor-related apoptosis-inducing ligand correlate with the severity of pulmonary hypertension, Pulm. Pharmacol. Ther., 33 (2015), 39-46. |
[17] | A. G. Hameed, N. D. Arnold, J. Chamberlain, et al., Inhibition of tumor necrosis factor-related Apoptosis-inducing ligand reverses experimental pulmonary hypertension, J. Exp. Med., 216 (2012), 1919-1935. |
[18] | G. S. Wu, TRAIL as a target in anti-cancer therapy, Cancer Lett., 285 (2009), 1-5. |
[19] | S. R. Wiley, K. Schooley, P. J. Smolak, et al., Identification and characterization of a new member of the TNF family that induces apoptosis, Immunity, 3 (1995), 673-682. |
[20] | H. B. Schiller, I. E. Fernandez, G. Burgstaller, et al., Time- and compartment-resolved proteome profiling of the extracellular niche in lung injury and repair, Mol. Syst. Biol., 11 (2015), 819. |
[21] | H. N. LeBlanc, A. Ashkenazi, Apo2L/TRAIL and its death and decoy receptors, Cell Death Differ., 10 (2003), 66-75. |
[22] |
P. Secchiero, F. Corallini, M. G. di Iasio, et al., TRAIL counteracts the proadhesive activity of inflammatory cytokines in endothelial cells by down-modulating CCL8 and CXCL10 chemokine expression and release, Blood, 105 (2005), 3413-3419. doi: 10.1182/blood-2004-10-4111
![]() |
[23] | A. Almasan and A. Ashkenazi, Apo2L/TRAIL: Apoptosis signaling, biology, and potential for cancer therapy, Cytokine Growth Factor Rev., 14 (2003), 337-348. |
[24] | P. Secchiero, A. Gonelli, E. Carnevale, et al., TRAIL promotes the survival and proliferation of primary human vascular endothelial cells by activating the Akt and Erk pathway, Circulation, 107 (2003), 2250-2256. |
[25] | J. P. Sheridan, S. A. Marsters, R. M. Pitti, et al., Control of TRAIL-induced apoptosis by a family of signaling and decoy receptors, Science, 277 (1997), 818-821. |
[26] | C. Falschlehner, C. H. Emmerich, B. Gerlach, et al., TRAIL signalling: Decisions between life and death, Int. J. Biochem. Cell Biol., 39 (2007), 1462-1475. |
[27] | S. M. Mariani and P. H. Krammer, Differential regulation of TRAIL and CD95 ligand in transformed cells of the T and B lymphocyte lineage, Eur. J. Immunol., 28 (1998), 973-982. |
[28] |
P. Secchiero, R. Candido, F. Corallini, et al., Systemic tumor necrosis factor-related apoptosis-inducing ligand delivery shows antiatherosclerotic activity in apolipoprotein E-null diabetic mice, Circulation, 114 (2006), 1522-1530. doi: 10.1161/CIRCULATIONAHA.106.643841
![]() |
[29] | X. Liu, V. R. Winrow, M. Horrocks, et al., Differential expression of TRAIL and its receptors relative to calcification in AAA, Biochem. Biophys. Res. Commun., 358 (2007), 18-23. |
[30] | S. P. Cartland, S. W. Genner, A. Zahoor, et al., Comparative Evaluation of TRAIL, FGF-2 and VEGF-A-Induced Angiogenesis In Vitro and In Vivo, Int. J. Mol. Sci., 17 (2016), 2025. |
[31] | J. R. Jackson, M. P. Seed, C. H. Kircher, et al., The codependence of angiogenesis and chronic inflammation, FASEB J., 11 (1997), 457-465. |
[32] | A. A. Eddy and C. M. Giachelli, Renal expression of genes that promote interstitial inflammation and fibrosis in rats with protein-overload proteinuria, Kidney Int., 47 (1995), 1546-1557. |
[33] | F. Verrecchia, and A. Mauviel, Transforming growth factor-beta signaling through the Smad pathway: Role in extracellular matrix gene expression and regulation, J. Invest. Dermatol., 118 (2002), 211-215. |
[34] | A. Leask, D. J. Abraham, D. R. Finlay, et al., Dysregulation of transforming growth factor beta signaling in scleroderma: Overexpression of endoglin in cutaneous scleroderma fibroblasts, Arthritis Rheumatol., 46 (2002), 1857-1865. |
[35] | K. J. Gordon, M. Dong, E. M. Chislock, et al., Loss of type III transforming growth factor beta receptor expression increases motility and invasiveness associated with epithelial to mesenchymal transition during pancreatic cancer progression, Carcinogenesis, 29 (2008), 252-262. |
[36] | G. Sánchez-Duffhues, C. Hiepen, P. Knaus, et al., Bone morphogenetic protein signaling in bone homeostasis, Bone, 80 (2015), 43-59. |
[37] | A. G. de Vinuesa, S. Abdelilah-Seyfried, P. Knaus, et al., BMP signaling in vascular biology and dysfunction, Cytokine Growth Factor Rev., 27 (2016), 65-79. |
[38] | C. H. Heldin, K. Miyazono and P. Ten Dijke, TGF-beta signaling from cell membrane to nucleus through SMAD proteins, Nature, 390 (1997), 465-471. |
[39] | K. Miyazono, K. Kusanagi and H. Inoue, Divergence and convergence of TGF-beta/BMP signaling, J. Cell. Physiol., 187 (2001), 265-276. |
[40] | H. Yagita, K. Takeda, Y. Hayakawa, et al., TRAIL and its receptors as targets for cancer therapy, Cancer Sci., 95 (2004), 777-783.. |
[41] | T. Kunieda, N. Nakanishi, T. Satoh, et al., Prognoses of primary pulmonary hypertension and chronic majorvessel thromboembolic pulmonary hypertension determined from cumulative survival curves, Intern. Med., 38 (1999), 543-546. |