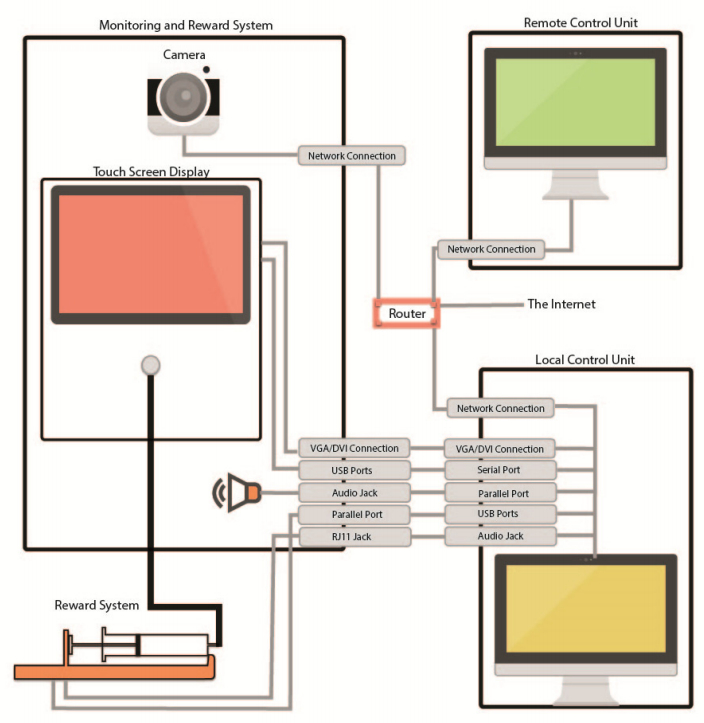
Citation: Michaela D Curry, Abigail Zimmermann, Mohammadbagher Parsa, Mohammad-Reza A. Dehaqani, Kelsey L Clark, Behrad Noudoost. A Cage-Based Training System for Non-Human Primates[J]. AIMS Neuroscience, 2017, 4(3): 102-119. doi: 10.3934/Neuroscience.2017.3.102
[1] | Robert Friedman . Themes of advanced information processing in the primate brain. AIMS Neuroscience, 2020, 7(4): 373-388. doi: 10.3934/Neuroscience.2020023 |
[2] | Yang Yu, Tsuyoshi Setogawa, Jumpei Matsumoto, Hiroshi Nishimaru, Hisao Nishijo . Neural basis of topographical disorientation in the primate posterior cingulate gyrus based on a labeled graph. AIMS Neuroscience, 2022, 9(3): 373-394. doi: 10.3934/Neuroscience.2022021 |
[3] | Marian E. Berryhill . Longitudinal tDCS: Consistency across Working Memory Training Studies. AIMS Neuroscience, 2017, 4(2): 71-86. doi: 10.3934/Neuroscience.2017.2.71 |
[4] | I. Prieto, A.B. Segarra, M. de Gasparo, M. Ramírez-Sánchez . Neuropeptidases, Stress, and Memory—A Promising Perspective. AIMS Neuroscience, 2016, 3(4): 487-501. doi: 10.3934/Neuroscience.2016.4.487 |
[5] | Wanda M. Snow, Brenda M. Stoesz, Judy E. Anderson . The Cerebellum in Emotional Processing: Evidence from Human and Non-Human Animals. AIMS Neuroscience, 2014, 1(1): 96-119. doi: 10.3934/Neuroscience.2014.1.96 |
[6] | Timothy J. Ricker . The Role of Short-term Consolidation in Memory Persistence. AIMS Neuroscience, 2015, 2(4): 259-279. doi: 10.3934/Neuroscience.2015.4.259 |
[7] | Mirela Dubravac, Beat Meier . Stimulating the parietal cortex by transcranial direct current stimulation (tDCS): no effects on attention and memory. AIMS Neuroscience, 2021, 8(1): 33-46. doi: 10.3934/Neuroscience.2021002 |
[8] | Damien Moore, Paul D Loprinzi . The association of self-reported physical activity on human sensory long-term potentiation. AIMS Neuroscience, 2021, 8(3): 435-447. doi: 10.3934/Neuroscience.2021023 |
[9] | Anastasios A. Mirisis, Anamaria Alexandrescu, Thomas J. Carew, Ashley M. Kopec . The Contribution of Spatial and Temporal Molecular Networks in the Induction of Long-term Memory and Its Underlying Synaptic Plasticity. AIMS Neuroscience, 2016, 3(3): 356-384. doi: 10.3934/Neuroscience.2016.3.356 |
[10] | Chris Cadonic, Benedict C. Albensi . Oscillations and NMDA Receptors: Their Interplay Create Memories. AIMS Neuroscience, 2014, 1(1): 52-64. doi: 10.3934/Neuroscience.2014.1.52 |
The human brain is highly complex. Replication of its functions through laboratory tests and computer models is not always feasible. The use of animals in neuroscience research provides a model similar in complexity to the human brain and is necessary to further research advances. Non-human primates (NHPs) are extensively used as animal models for scientific research. An NHP brain has many similarities to a human brain in regards to size, connectivity, functional areas, and aging processes, making it an ideal research model. NHPs can be trained to perform tasks that allow researchers to evaluate cognition and behavior. Specifically in neurophysiological studies, NHPs are trained on cognitive tasks prior to collecting neurophysiological data. A well-trained animal is necessary to obtain sufficient experimental results. Therefore, optimizing the training method is fundamental to successful research involving NHPs.
However, training procedures are likely far from being optimized. Various experiments require physically restraining the NHP during training or collecting data, both for the nature of the data itself and for the safety of the personnel involved. The primate chair is a common restraint device that, with the right modifications, is suitable for a wide array of scientific experiments. However, the animal is required to maintain a fixed position in the chair throughout the training and its ability to move is reduced. Providing a more comfortable training environment ultimately reduces stress, potentially improving the animal's well-being, the speed of training, and the animal's performance. Here we present the details of a system to train animals in their home cage instead of the classic chair-restraint training.
Training primates without chair-restraint has been used for a variety of species including chimpanzees, baboons, and gorillas [1,2,3,4,5,6,7], and also for bonnet macaques, marmosets, pigtail macaques, tufted capuchin monkeys, squirrel monkeys, lion-tailed macaques, long-tailed macaques, and mouse lemurs [2,3,4,8,9,10,11,12,13,14,15,16,17]. Several groups have also trained rhesus macaques without chair restraining the animal [13,18,19,20,21,22,23,24,25,26,27,28,29,30]. Most of these groups have provided a computer-based interactive environment interface where animals are individually or even group housed [2,3,4,5,6,7,10,11,12,13,14,15,16,17,19,21,23,24,25,26,27,28,29,30,31,32,33,34,35,36]. Overall, in-cage touch screen training has been useful to many experiments, such as those conducted by Srihasam et al. [37]. Similar to the training methods which used rhesus macaques, focusing on behavior and cognition using a touch screen within the animal's own home cage [25,26,27,28], we have designed, built and successfully utilized a fully portable cage-mountable system to train NHPs and here we describe the technical specifications, construction, and operation of the system. This system has the potential to ease and expedite the behavioral training of NHPs on a variety of tasks by allowing the animal greater control over when and how long it chooses to work, being less disruptive to the monkey's social interactions, and presumably eliminating some of the stress found in chair training. Moreover, we present data collected during the behavioral training on a visual memory and change detection task, demonstrating the feasibility of this approach for training on cognitive tasks.
The protocols for all experimental and behavioral procedures were approved by the Montana State University Institutional Animal Care and Use Committee. The in-cage training system described here was designed for training rhesus macaques, but may be utilized to train and test various species of NHPs (Figure 1). This system makes home cage training possible for all NHPs with a design allowing the system to be portable and mountable on the NHP's cage. Additionally, the training system is designed to be safe, robust, and suitable for animal operation in-cage.
The system includes four components: (1) local control unit, (2) remote control unit, (3) animal interface, and (4) reward delivery system (Figure 1).
(1) Local Control Unit: includes a desktop computer controlling the behavioral paradigm using two software. The minimum requirements for the desktop computer included an INTEL Pentium or Core i2 Processor, 2 GB of memory (DDR3 RAM), and 2 VGA ports for display. The system also included a line print terminal (LPT) also known as a parallel port to trigger the pump for reward delivery, 1 serial port to control the pump, and 1 RJ-45 port. The minimum hardware requirements for building or purchasing the desktop computer for the local control unit are listed in Table 1. The local control unit was located within the animal research facility but outside of the room housing the rhesus macaques. The graphical representation of the training behavioral paradigm was sent from the local control unit to the animal interface inside the subject's home cage (Figure 2).
Part Name | Minimum Requirement |
CPU | INTEL Pentium or i3 |
RAM | 2 GB DDR3 |
Graphics card | 1 GB with 2 VGA port |
Ports and interfaces | Includes 1 parallel port, 1 VGA port, 1 serial port, and 1 RJ-45 |
Touchscreen monitor | ELO 1739L open-frame touchscreen LCD monitor, 43.18 cm, 1280 × 1024 |
USB cable | USB 2.0 A to B cable |
Speaker | Logitech plug & play 10 watt |
Pump | NE-4500 double OEM syringe pump |
Camera | Day/night D-link IP camera |
Cage mounting frame | 30.5 × 37.15 cm |
Listed are the various parts of the in-cage training system and the minimum requirement for each part. |
We employed the Presentation software (Neurobehavioral Systems, Inc., Berkeley, CA) for the working memory task and Matlab Psychophysics Toolbox (The MathWorks, Inc., Natick, MA) for the change detection task to control the stimulus presentation, behavioral data acquisition and reward delivery. The various programming scripts used for the working memory task and change detection task are available upon request. Both toolboxes are available to download online and they are widely used in psychological and neurophysiological experiments.
(2) Animal Interface: includes a touchscreen monitor, a mounting frame, a speaker for auditory feedback, and a lever. The minimum requirements for the animal interface include an ELO 1739L open-frame touchscreen LCD monitor 17 inch with a 1280 × 1024 resolution (Elo Touch Solutions, Inc., Milpitas, CA), and a USB 2.0 A to B cable. The minimum requirements for the electrical and mechanical parts of the animal interface are listed in Table 1. The monitor is 4.90 × 36.80 × 30.48 cm in size, embedded in a mounting frame of 30.5 × 37.15 cm in size, which was designed to snugly fit the side panel of the primate's cage. The frame stayed on the cage during the week and was removed once a week for cleaning. The frame was light enough (approximately 16 kilograms) for a single person to easily mount and remove it. The frame was designed in a way that the animal did not need to reach outside the cage to access the monitor. The designed frame fitting the Primate Products cages is commercially available upon request. Tasks were designed in a way that required a specific touch answer from the animal in response to a visual stimulus. The touch position was recorded by the local control unit. In the attention task, a lever response was also used in addition to a touch response and these data were also recorded by the local control unit. The local control unit could then send feedback based on the animal's performance via a speaker. We used a speaker as part of the animal interface as well as a juice delivery pump as a reward system.
(3) Reward System: includes a syringe pump managed by the local control unit. When the trial was correct, the local control unit triggered the reward system via a TTL pulse through a parallel port. Once the pump was triggered, it delivered a user defined juice amount to the subject. The reward system allowed the reward duration or the number of rewards to be set via the SyringePumpPro application (New Era Pump Systems, Inc., Farmingdale, NY) in the local control unit. We used a NE-4500 double OEM syringe pump. As mentioned above, in addition to the juice reward the animal could receive auditory feedback. Figure 2 shows the local control unit, animal interface, and the reward system; the minimum requirements for the monitoring and reward system are listed in Table 1.
(4) Remote Control Unit: includes a camera and a remote desktop computer. We used a night vision capable D-Link IP-camera (D-Link Systems, Inc, Fountain Valley, CA) to screen the subject's activity during the experiment. The camera was accessible over the internet to the remote control unit. This arrangement provided the possibility of monitoring the entire system remotely when the experimenter was not physically present in the animals' vivarium. The remote control unit could also remotely manage the experiment by accessing the local control unit. The subject's screen, the reward settings, and the behavioral paradigm could all be monitored and manipulated via the remote control unit.
The in-cage training system was designed to allow the subject to have his own self-paced engagement with the task. The animal was in the same room with other monkeys but was separated into his own home cage for training. While training the subject, the system was turned on in the morning and remained on throughout the day, then turned off in the evening. This allowed the subject to have control over when and how long to work throughout the day and to take breaks on his own time. The subject worked five days a week, with two vacation days per week. Throughout the work days the subject's access to water was limited to the juice he earned performing the task. If the animal chose not to work he would receive water instead of juice at the end of the day. This water restriction helped enhance the animal's motivation to perform the task, however, later during the training phase, the animal usually preferred performing the task and receiving juice, even in the presence of full water access. We tested the system by training a rhesus monkey in two behavioral paradigms:
Delayed Match to Sample (DMS) Task: DMS is an object working memory task. In this task the animal has to remember an object throughout a delay and choose the same object among multiple distractors after the delay period (Figure 3). The DMS task can be a time consuming and challenging task for NHPs. We broke down the DMS task into several steps to train the rhesus macaque in his home cage. In the first stage of training we focused on encouraging the subject to touch the touchscreen monitor. A sample image that encompassed the entire screen was presented as the target area and by touching anywhere on the screen, the subject earned a 4 mL juice reward per correct trial. After the subject's performance was consistently 85% correct, the image was shrunk by approximately 20%. This process was continued until the sample image size was reduced to one square inch on the touchscreen. To verify that the subject was looking at the sample image and not just the center of the screen, the second stage of training involved moving the sample image to a new screen location for each new trial. Throughout all trials until this point, the same image was used each day for each trial, with the goal of keeping all experimental factors consistent except for the specific behavioral task. After the subject was proficient with the moving sample image, the match to sample task was introduced. This third stage of training consisted of an initially presented sample image which the subject touched, followed by two target images; one of the target images was the same as the initial sample image and the other target image was a distracter image. To perform a correct trial, the subject had to select the target image that matched the initial sample image. To discourage the subject from being impatient and randomly touching the screen, and to encourage the subject to focus on deliberately touching the target, preferably with only one finger, a punishment was introduced in which an incorrect trial resulted in a three second inter-trial pause. After the subject mastered the match to sample stage, a delay was introduced between the presentation of the sample image and the two target images. The delay started at 100 ms and eventually escalated to a 2 second delay. After learning how to maintain the object information throughout a 2 second delay, new images were introduced to teach the subject how to generalize the DMS rule. After proficiency with a certain pair of images (performance rate 85%) a new pair of images was used. The subject became familiar with approximately 39 different images, generalizing the DMS rule. The training process was completed after 100 training sessions.
Change Detection Task: We used the same in-cage system to also train the animal with a change detection task (Figure 4). In this task the animal monitors a visual stimulus to detect a temporary contrast change. Again, the change detection task was broken down into several steps to train the rhesus macaque in his home cage. The first stage involved the subject learning how to press the lever. The subject had to press down the lever for a set amount of time in order to receive a juice reward. It occurred naturally by introducing a new object (the lever) to the curious animal's environment. After the subject learned how to sufficiently depress the lever, a visual target was introduced (a red dot) and the subject had to depress the lever and touch the target while keeping the lever pressed. After the target was touched, a visual stimulus (Gabor grating) would appear onscreen. The animal had to hold the lever for the duration of the stimulus. The stimulus duration was then gradually increased. Once the subject learned how to keep the lever pressed while maintaining its focus to detect when the stimulus turns off, then the "blink detection" part was added. In blink trials the stimulus would turn off and the subject would release the lever and receive the juice but then the stimulus would reappear. We gradually reduced the blink time to 100 ms and the subject had to release the lever quickly after the stimulus change in order to receive a juice reward. The quick release of the lever was the key to trace whether the animal was detecting the change. Once the subject was able to detect a changing Gabor, we gradually introduced variability in the location of the stimulus and the time of the change. To ensure that the subject was validly responding to the change, a no change condition was added to the task. For the no change trial, the subject had to press the lever, touch the target on the monitor, and keep the lever depressed for the duration of a non-changing stimulus in order to receive a juice reward. The ultimate goal was to detect a "contrast change". Therefore, once the animal was trained on detecting a 100 ms blink (100% change in contrast), then the duration of stimulus change (100 ms) was kept constant and the animal was gradually trained to detect smaller and smaller changes in the stimulus contrast.
Training the object working memory task took about 100 training sessions. The training goal for the animal is to be able to generalize the rule to a novel object. Figure 5A shows the animal's ability to maintain object information throughout a delay period for two different objects early in training (day 17). At the start of the training session, the animal chose object A more often than object B regardless of which object was the sample. This bias decreased over the course of the training session and the animal gradually improved his performance for object B. This performance improvement showed the animal's ability to learn new objects. The animal's ability to generalize the rule is shown in Figure 5B. Later during the training (day 34), a new pair of objects were introduced and the animal was capable of maintaining both objects in his working memory from the start of the session.
Training was completed for the change detection task after 43 training sessions. The goal for the animal is to report the change by releasing the lever in response to a change in the visual stimulus. Figure 6A shows the animal's release time relative to the stimulus change early in training (day 16). At this point, the animal's release time did not closely match the stimulus change time, indicating that the animal had not yet mastered the task. Figure 6B shows the animal's performance later in training. The animal's release time more reliably followed the stimulus change time, showing that the animal was following the task rule. Figure 6C illustrates the animal's overall performance for an early vs. late day of training, showing a performance improvement as the training progressed.
The change detection task measures the animal's sensitivity to changes in a sensory signal (visual stimulus blink). After the animal was trained to detect a blink for the duration of 100 ms, we reduced the total signal change by keeping the blink duration constant and varying the magnitude of the luminance change. The animal performed well for the 100% contrast change condition (stimulus completely disappeared). To cover the dynamic range of the animal's performance the contrast change varied between 5%-65%. Figure 7A shows that the animal's performance depended on the contrast change, indicating that the animal was following the visual signal. The animal's performance gradually improved over time (Figure 7B), showing that the training stages enabled the animal to learn the task.
It is imperative to provide a low stress environment to enhance learning [38]. The ability to train NHPs in their own home cage could potentially expedite training and improve the animal's quality of life. Here we described the system we designed and used to successfully train an NHP on a change detection and a working memory task. We observed that the animal's motivation to perform these tasks was drastically higher compared to our previous experience of training other animals on similar tasks using a primate chair. This heightened motivation was also associated with faster training and higher performance. Moreover, once the animal was more familiar with the task they chose to perform the task even without water restriction. Rhesus monkeys are highly intelligent animals and a huge part of their daily care is providing them with sufficient mental activity and enrichment. Moving the training to the animal's cage not only expedited the training, it provided a great repertoire of behavioral enrichment throughout the day available at the animal's discretion.
The main impediment to exclusively using in-cage training is the need to track the eye position in most visual tasks. Eye tracking systems usually require the head to be stationary. Other groups have successfully used face masks to restrict the animal's head movement, potentially enabling tracking of their eye movements in their home cage [39,40,41,42,43,44]. Training the animal to use a face mask while performing behavioral tasks could drastically change the set of behavioral paradigms that these animals can learn in their home cage. If successful, this addition of eye tracking to this system could move most of the behavioral training to the animal's home cage. Ultimately once wireless electrophysiological recording devices are improved, recording the animal's behavioral and neural responses in more natural environments will be possible.
The work was supported by MSU start-up fund, Whitehall 2014-5-18, NIH R01EY026924, and NSF1439221 and 1632738 grants to BN.
All authors declare no conflicts of interest in this paper.
[1] |
Rumbaugh DM, Hopkins WD, Washburn DA, et al. (1989) Lana chimpanzee learns to count by NUMATH: a summary of a videotaped experimental repxort. Psychol Rec 39: 459-470. doi: 10.1007/BF03395074
![]() |
[2] |
Spinelli S, Pennanen L, Dettling AC, et al. (2004) Performance of the marmoset monkey on computerized tasks of attention and working memory. Cogn Brain Res 19: 123-137. doi: 10.1016/j.cogbrainres.2003.11.007
![]() |
[3] |
Mandell DJ, Sackett GP (2009) Comparability of developmental cognitive assessments between standard and computer testing methods. Dev Psychobiol 51: 1-13. doi: 10.1002/dev.20329
![]() |
[4] | Truppa V, Garofoli D, Castorina G, et al. (2010) Identity concept learning in matching-to-sample tasks by tufted capuchin monkeys (Cebus apella). Anim Cogn 13: 835-848. |
[5] |
Vonk J, Torgerson-White L, McGuire M, et al. (2014) Quantity estimation and comparison in western lowland gorillas (Gorilla gorilla gorilla). Anim Cogn 17: 755-765. doi: 10.1007/s10071-013-0707-y
![]() |
[6] |
Allritz M, Call J, Borkenau P (2016) How chimpanzees (Pan troglodytes) perform in a modified emotional Stroop task. Anim Cogn 19: 435-449. doi: 10.1007/s10071-015-0944-3
![]() |
[7] |
Wagner KE, Hopper LM, Ross SR (2016) Asymmetries in the production of self-directed behavior by chimpanzees and gorillas during a computerized cognitive test. Anim Cogn 19: 343-350. doi: 10.1007/s10071-015-0937-2
![]() |
[8] |
Andrews MW, Rosenblum LA (1993) Live-Social-Video Reward Maintains Joystick Task Performance in Bonnet Macaques. Percept Mot Skills 77: 755-763. doi: 10.2466/pms.1993.77.3.755
![]() |
[9] |
Andrews MW (1993) Video-task paradigm extended to Saimiri. Percept Mot Skills 76: 183–191. doi: 10.2466/pms.1993.76.1.183
![]() |
[10] | Andrews MW, Rosenblum LA (1994) Automated recording of individual performance and hand preference during joystick-task acquisition in group-living bonnet macaques (Macaca radiata). J. Comp Psychol 108: 358-362. |
[11] | Andrews MW (1994) Effective use of a joystick by an infant monkey. Am J Primatol 32: 141-144. |
[12] |
Leighty KA, Fragaszy DM (2003) Joystick acquisition in tufted capuchins (Cebus apella). Anim Cogn 6: 141-148. doi: 10.1007/s10071-003-0176-9
![]() |
[13] | Scott L, Pearce P, Fairhall S, et al. (2010) Training Nonhuman Primates to Cooperate With Scientific Procedures in Applied Biomedical Research. J Appl Anim Welf Sci 6: 199-207. |
[14] | Judge PG, Kurdziel LB, Wright RM, et al. (2012) Picture recognition of food by macaques (Macaca silenus). Anim Cogn 15: 313-325. |
[15] |
Joly M, Ammersdörfer S, Schmidtke D, et al. (2014) Touchscreen-Based Cognitive Tasks Reveal Age-Related Impairment in a Primate Aging Model, the Grey Mouse Lemur (Microcebus murinus). PLoS One 9: e109393. doi: 10.1371/journal.pone.0109393
![]() |
[16] |
Schmitt V, Federspiel I, Eckert J, et al. (2016) Do monkeys compare themselves to others? Anim Cogn 19: 417-428. doi: 10.1007/s10071-015-0943-4
![]() |
[17] | Jacobsen ME, Barros M, Maior RS (2017) MK-801 reduces sensitivity to Müller-Lyer's illusion in capuchin monkeys. Behav Brain Res 316: 54-58. |
[18] |
Washburn DA, Hopkins WD, Rumbaugh DM (1989) Video-task assessment of learning and memory in macaques (Macaca mulatta): effects of stimulus movement on performance. J Exp Psychol Anim Behav Process 15: 393-400. doi: 10.1037/0097-7403.15.4.393
![]() |
[19] | Richardson WK, Washburn DA, Hopkins WD, et al. (1990) The NASA/LRC computerized test system. Behav Res Methods Instruments & amp Comput 22: 127-131. |
[20] | Washburn DA, Hopkins WD, Rumbaugh DM (1991) Perceived control in rhesus monkeys (Macaca mulatta): enhanced video-task performance. J Exp Psychol Anim Behav Process 17: 123-129. |
[21] | Washburn DA, Rumbaugh DM (1992) Investigations of rhesus monkey video-task performance: evidence for enrichment. Contemp Top Lab Anim Sci 31: 6-10. |
[22] |
Washburn DA, Hopkins WD (1994) Videotape-versus pellet-reward preferences in joystick tasks by macaques. Percept Mot Skills 78: 48-50. doi: 10.2466/pms.1994.78.1.48
![]() |
[23] |
Weed MR, Taffe M A, Polis I, et al. (1999) Performance norms for a rhesus monkey neuropsychological testing battery: acquisition and long-term performance. Brain Res Cogn Brain Res 8: 185-201. doi: 10.1016/S0926-6410(99)00020-8
![]() |
[24] |
Fagot J, Paleressompoulle D (2009) Automatic testing of cognitive performance in baboons maintained in social groups. Behav Res Methods 41: 396-404. doi: 10.3758/BRM.41.2.396
![]() |
[25] |
Watson KK, Ghodasra JH, Furlong MA, et al. (2012) Visual preferences for sex and status in female rhesus macaques. Anim Cogn 15: 401-407. doi: 10.1007/s10071-011-0467-5
![]() |
[26] |
Gazes RP, Brown EK, Basile BM, et al. (2013) Automated cognitive testing of monkeys in social groups yields results comparable to individual laboratory-based testing. Anim Cogn 16: 445-458. doi: 10.1007/s10071-012-0585-8
![]() |
[27] |
Hutsell BA, Banks ML (2015) Effects of environmental and pharmacological manipulations on a novel delayed nonmatching-to-sample 'working memory' procedure in unrestrained rhesus monkeys. J Neurosci Methods 251: 62-71. doi: 10.1016/j.jneumeth.2015.05.009
![]() |
[28] |
Calapai A, Berger M, Niessing M, et al. (2017) A cage-based training, cognitive testing and enrichment system optimized for rhesus macaques in neuroscience research. Behav Res Methods 49: 35-45. doi: 10.3758/s13428-016-0707-3
![]() |
[29] |
Sorwell KG, Renner L, Weiss AR, et al. (2017) Cognition in aged rhesus monkeys: effect of DHEA and correlation with steroidogenic gene expression. Genes Brain Behav 16: 361-368. doi: 10.1111/gbb.12351
![]() |
[30] | Fiuzat EC, Rhodes SEV, Murray EA (2017) The Role of Orbitofrontal–Amygdala Interactions in Updating Action–Outcome Valuations in Macaques. J Neurosci 10: 1839-1816. |
[31] |
Fagot J, Parron C (2010) Relational Matching in Baboons (Papio papio) With Reduced Grouping Requirements. J Exp Psychol Anim Behav Process 36: 184-193. doi: 10.1037/a0017169
![]() |
[32] |
Fagot J, Bonté E (2010) Automated testing of cognitive performance in monkeys: Use of a battery of computerized test systems by a troop of semi-free-ranging baboons (Papio papio). Behav Res Methods 42: 507-516. doi: 10.3758/BRM.42.2.507
![]() |
[33] | Fagot J, Gullstrand J, Kemp C, et al. (2014) Effects of freely accessible computerized test systems on the spontaneous behaviors and stress level of Guinea baboons (Papio papio). Am J Primatol 76: 56-64. |
[34] | Washburn DA, Rumbaugh DM (1992) Testing primates with joystick-based automated apparatus: Lessons from the Language Research Center's Computerized Test System. Behav Res Methods Instruments Comput 24: 157-164. |
[35] |
Washburn DA, Harper S, Rumbaugh DM (1994) Computer-task testing of rhesus monkeys (Macaca mulatta) in the social milieu. Primates 35: 343-351. doi: 10.1007/BF02382730
![]() |
[36] |
Bennett AJ, Perkins CM, Tenpas PD, et al. (2016) Moving evidence into practice: cost analysis and assessment of macaques' sustained behavioral engagement with videogames and foraging devices. Am J Primatol 78: 1250-1264. doi: 10.1002/ajp.22579
![]() |
[37] | Srihasam K, Vincent JL, Livingstone MS (2014) Novel domain formation reveals proto-architecture in inferotemporal cortex. Nat Neurosci 12: 1776-1783. |
[38] |
Bangasser DA, Shors TJ (2010) Critical brain circuits at the intersection between stress and learning. Neurosci Biobehav Rev 34: 1223-1233. doi: 10.1016/j.neubiorev.2010.02.002
![]() |
[39] |
Slater H, Milne AE, Wilson B, et al. (2016) Individually customisable non-invasive head immobilisation system for non-human primates with an option for voluntary engagement. J Neurosci Methods 269: 46-60. doi: 10.1016/j.jneumeth.2016.05.009
![]() |
[40] |
Howell LL, Hoffman JM, Votaw JR, et al. (2001) An apparatus and behavioral training protocol to conduct positron emission tomography (PET) neuroimaging in conscious rhesus monkeys. J Neurosci Methods 106: 161-169. doi: 10.1016/S0165-0270(01)00345-4
![]() |
[41] |
Drucker CB, Carlson ML, Toda K, et al. (2015) Non-invasive primate head restraint using thermoplastic masks. J Neurosci Methods 253: 90-100. doi: 10.1016/j.jneumeth.2015.06.013
![]() |
[42] |
Machado CJ, Nelson EE (2011) Eye-tracking with nonhuman primates is now more accessible than ever before. Am J Primatol 73: 562-569. doi: 10.1002/ajp.20928
![]() |
[43] | Fairhall SJ, Dickson CA, Scott L, et al. (2006) A non-invasive method for studying an index of pupil diameter and visual performance in the rhesus monkey. J Med Primatol 35: 67-77. |
[44] |
Kiorpes L, Price T, Hall-Haro C, et al. (2012) Development of sensitivity to global form and motion in macaque monkeys (Macaca nemestrina). Vision Res 63: 34-42. doi: 10.1016/j.visres.2012.04.018
![]() |
1. | Laura Hansmeyer, Pinar Yurt, Naubahar Agha, Attila Trunk, Michael Berger, Antonino Calapai, Stefan Treue, Alexander Gail, Home-Enclosure-Based Behavioral and Wireless Neural Recording Setup for Unrestrained Rhesus Macaques, 2023, 10, 2373-2822, ENEURO.0285-22.2022, 10.1523/ENEURO.0285-22.2022 | |
2. | Thilo Womelsdorf, Christopher Thomas, Adam Neumann, Marcus R. Watson, Kianoush Banaie Boroujeni, Seyed A. Hassani, Jeremy Parker, Kari L. Hoffman, A Kiosk Station for the Assessment of Multiple Cognitive Domains and Cognitive Enrichment of Monkeys, 2021, 15, 1662-5153, 10.3389/fnbeh.2021.721069 | |
3. | Lalitta Suriya-Arunroj, Motee Chimngam, Chutikan Chamnongpakdee, Thipchompoo Sing-Ayudthaya, Chunapa Linchekhaw, Nopparat Kongsombat, Nutchanat Suttisan, Behavioral Training in First-Generation Long-Tailed Macaques (Macaca fascicularis) for Improved Husbandry and Veterinary Procedures, 2024, 14, 2076-2615, 2369, 10.3390/ani14162369 | |
4. | Francesco Ceccarelli, Fabrizio Londei, Giulia Arena, Aldo Genovesio, Lorenzo Ferrucci, Home-Cage Training for Non-Human Primates: An Opportunity to Reduce Stress and Study Natural Behavior in Neurophysiology Experiments, 2025, 15, 2076-2615, 1340, 10.3390/ani15091340 |
Part Name | Minimum Requirement |
CPU | INTEL Pentium or i3 |
RAM | 2 GB DDR3 |
Graphics card | 1 GB with 2 VGA port |
Ports and interfaces | Includes 1 parallel port, 1 VGA port, 1 serial port, and 1 RJ-45 |
Touchscreen monitor | ELO 1739L open-frame touchscreen LCD monitor, 43.18 cm, 1280 × 1024 |
USB cable | USB 2.0 A to B cable |
Speaker | Logitech plug & play 10 watt |
Pump | NE-4500 double OEM syringe pump |
Camera | Day/night D-link IP camera |
Cage mounting frame | 30.5 × 37.15 cm |
Listed are the various parts of the in-cage training system and the minimum requirement for each part. |
Part Name | Minimum Requirement |
CPU | INTEL Pentium or i3 |
RAM | 2 GB DDR3 |
Graphics card | 1 GB with 2 VGA port |
Ports and interfaces | Includes 1 parallel port, 1 VGA port, 1 serial port, and 1 RJ-45 |
Touchscreen monitor | ELO 1739L open-frame touchscreen LCD monitor, 43.18 cm, 1280 × 1024 |
USB cable | USB 2.0 A to B cable |
Speaker | Logitech plug & play 10 watt |
Pump | NE-4500 double OEM syringe pump |
Camera | Day/night D-link IP camera |
Cage mounting frame | 30.5 × 37.15 cm |
Listed are the various parts of the in-cage training system and the minimum requirement for each part. |