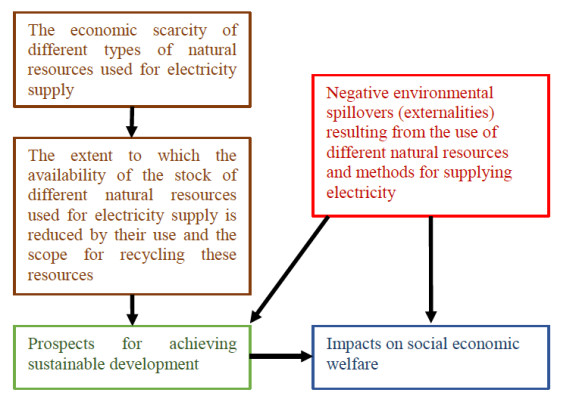
Citation: Clement Allan Tisdell. Renewable energy use and the renewable energy sector’s development: public finance, environmental externalities and sustainability[J]. Green Finance, 2019, 1(2): 156-173. doi: 10.3934/GF.2019.2.156
[1] | Wen-Tien Tsai . Green finance for mitigating greenhouse gases and promoting renewable energy development: Case study in Taiwan. Green Finance, 2024, 6(2): 249-264. doi: 10.3934/GF.2024010 |
[2] | Ali Omidkar, Razieh Es'haghian, Hua Song . Developing a machine learning model for fast economic optimization of solar power plants using the hybrid method of firefly and genetic algorithms, case study: optimizing solar thermal collector in Calgary, Alberta. Green Finance, 2024, 6(4): 698-727. doi: 10.3934/GF.2024027 |
[3] | Ana Ramos, Filipa Magalhães, Diogo Neves, Nelson Gonçalves, António Baptista, Teresa Mata, Nuno Correia . Wind energy sustainability in Europe—A review of knowledge gaps, opportunities and circular strategies. Green Finance, 2023, 5(4): 562-602. doi: 10.3934/GF.2023022 |
[4] | Yoram Krozer . Financing of the global shift to renewable energy and energy efficiency. Green Finance, 2019, 1(3): 264-278. doi: 10.3934/GF.2019.3.264 |
[5] | Reinhard Haas, Amela Ajanovic, Jasmine Ramsebner, Theresia Perger, Jaroslav Knápek, Jan W. Bleyl . Financing the future infrastructure of sustainable energy systems. Green Finance, 2021, 3(1): 90-118. doi: 10.3934/GF.2021006 |
[6] | Mihaela Onofrei, Bogdan Narcis Fîrțescu, Florin Oprea, Dana Claudia Cojocaru . The effects of environmental patents on renewable energy consumption. Green Finance, 2024, 6(4): 630-648. doi: 10.3934/GF.2024024 |
[7] | Alexander Ryota Keeley, Shunsuke Managi . The importance of financial cost for renewable energy projects: economic viability assessment of renewable hybrid mini-grid systems in Indonesia. Green Finance, 2019, 1(2): 139-155. doi: 10.3934/GF.2019.2.139 |
[8] | Kalomoira Zisopoulou, Dionysia Panagoulia . Solar Power: A new mathematical definition and theoretical proof it is a Green Public Good. Green Finance, 2019, 1(3): 312-327. doi: 10.3934/GF.2019.3.312 |
[9] | Biljana Ilić, Dragica Stojanovic, Gordana Djukic . Green economy: mobilization of international capital for financing projects of renewable energy sources. Green Finance, 2019, 1(2): 94-109. doi: 10.3934/GF.2019.2.94 |
[10] | Laura Grumann, Mara Madaleno, Elisabete Vieira . The green finance dilemma: No impact without risk – a multiple case study on renewable energy investments. Green Finance, 2024, 6(3): 457-483. doi: 10.3934/GF.2024018 |
Human-induced global warming and climate change pose a serious threat to worldwide ecological, economic, and social sustainability. The major contributor to global warming (but not the only one) is the release into the atmosphere of greenhouse gases (particularly carbon dioxide) as a result of the combustion of fossil fuels, especially coal (Liski and Vehviläinen, 2017). Despite the dismissal of this interconnection by climate change deniers (such as Mr. Donald Trump, the current President of the USA), scientific evidence in support of the above relationship is extremely strong. As a result, several governments (for example, in Australia and China)1 are providing financial support to increase renewable energy use and to foster the development of their natural renewable energy sectors in order to reduce their dependence particularly on coal (and to a lesser extent on natural gas) as sources of electricity generation. At the federal level in Australia, a government body, the Clean Energy Finance Corporation is investing in new renewable projects and the government is underwriting loans for clean energy projects and guaranteeing a floor price for the energy produced (White, 2018). Several Australian State Governments are also providing economic incentives for increased use of clean electricity. The International Energy Agency reported in 2015 that worldwide, a quarter of a trillion euros is being spent annually to foster the renewable energy sector (Liski and Vehviläinen, 2017)
1 Several states in the United States have also adopted economic policies to promote the use and development of renewable energy technologies (Joskow, 2011). However, at the federal level, the Trump administration has adopted policies that favor fossil fuel use, unlike the previous administration.
Global energy use and carbon dioxide emissions have risen rapidly since 1800, particularly since 1950 (Whitmee et al., 2015). Furthermore, dependence on electricity as a source of energy is likely to increase in the coming years, as combustion engines are increasingly replaced by electric motors, for example, as electric cars become more common. Electronic technologies are also highly dependent on the supply of electricity, and their use is expanding rapidly. Consequently, the demand for electricity is likely to rise substantially in the future. This will increase the importance of producing "clean" electricity.
The subject of this article is important because prevailing methods of energy use for electricity supply are major contributors to global warming and therefore, increase the likelihood of unsustainable economic development occurring. In addition, the use of some natural resources for producing electricity depletes their stock and this can pose a long-term barrier to supplying electricity. Further, apart from the climate change consequences of some forms of energy production, e.g. those involving the combustion of fossil fuels, other types of negative environmental externalities (spillovers) generated by natural resource use to produce energy for supplying electricity need to be considered. The nature and importance of these spillovers vary with the types of natural resources used to produce electricity and the techniques used for this production.
Figure 1 provides an indication of the type of natural resource and environmental factors that need to be taken into account in assessing the prospects and social worth of using different types of natural resources to supply electricity.
The purpose of this article is to consider (as well as to assess, in the light of economic and other principles) why and how governments provide financial support for the use of renewable energy resources and for the development of renewable energy sectors. The main focus will be on the use of solar and wind energy for supplying electricity and the challenges that dependence on these energy sources poses. However, in order to appropriately analyze the type of resources and environmental challenges we face and the relevance of public financial policies for retarding global warming, it is important to realize that a better classification of energy resources (than the current one) is needed. In addition, we must distinguish clearly between the different (general) types of environmental/sustainability concerns that arise in assessing the desirability of alternative sources of electricity (energy) production.
The method adopted in developing this article is to take into account relevant current literature about this subject and to apply economic principles to the topic. In addition, original concepts will be presented in order to provide new insights into this field of enquiry.
This article is developed in the following sequence:
1. A brief literature review;
2. An improved and more relevant classification of energy resources for sustainability purposes is proposed;
3. Sustainability concerns are identified;
4. The types of government financial measures are outlined which could encourage the use of flow energy resources (mainly solar and wind) for electricity production;
5. The reasons why governments do and should support the development of and the use of solar and wind power are presented;
6. The difficulties faced as a result of increasing the reliance on solar and wind power for electricity generation are examined; and
7. Concluding comments follow.
The development of the renewable energy sector has occurred rapidly in recent times. The academic literature is behind in its assessment of the most recent developments in this sector, for example, the increased use of lithium-ion batteries to store electricity. This is an important means of transforming electricity obtained from flow resources to dispatchable stock resources. This article gives particular attention to this development. The cost of supplying electricity by using solar and wind power has fallen rapidly. In addition, the cost of storing electricity using lithium-ion batteries has fallen dramatically since 1990, mainly due to engineering advances and learning by doing (Draper, 2019). Storage technologies for electricity can reduce large differences between the availability of electricity and the variations in the demand for it.
It is generally accepted that using fossil fuels to produce electricity is much less environmentally friendly than its generation by solar or wind power. However, much of the available literature only focuses on the environmental effects at the electricity generation stage of production with the prime focus frequently being on the extent of CO2 emissions. It is argued in this article that a wider perspective is needed for socially assessing the social worth of alternative techniques for supplying electricity. Ideally, account should be taken of the whole product chain involved in electricity supply as well as the inter-industry flow-on effects. Moreover, the complete life-cycle of production units involved in this chain should be accounted for.
In relation to public finances, Borenstein (2012) has argued that if suppliers of dirty electricity (fossil-based supply) were required to pay for the environmental costs associated with their electricity supply, there would be no case for subsidizing clean energy supplies. However, Acemoğlu, Akcigit, Hanley and Kerr (2016) point out that if there are technology spillovers from the development of clean technologies for electricity production, a subsidy for developing these technologies would still be justified on economic grounds. Furthermore, infant industry agreements may help to justify such a subsidy.
In practice, political considerations cannot be ignored by governments in their choice of policy instruments for intervening in the way electricity is produced. For example, in some jurisdictions, making electricity producers who rely on fossil fuels to generate electricity pay for their pollution can be unpopular with the electorate. One reason why this can happen is that a very high proportion of these payments are passed on to the end-users of electricity in some countries (Fabra and Reguant, 2014). On the whole, subsidies for the development and use of clean energy are likely to be more palatable to many voters than taxes or fossil fuel use. Such subsidies may, however, be opposed by enterprises that have a vested interest in the use of fossil fuels.
Liski and Vehviläinen (2017) develop a model which demonstrates that subsidizing renewable energy for electricity supply reduces the price of electricity if storage of electricity is possible. Given their model, they demonstrate that it increases consumers' surplus and reduces the economic rents of generators of electricity who rely on fossil fuels for their electricity production. Fossil fuel production of electricity is likely to come under increasing economic threat as the cost of supplying electricity by relying on renewable resources falls and the costs of storing this electricity continues to fall, thereby increasing its dispatchability.
Another issue which has been the subject of a debate in the energy economics literature is how should the costs of intermittent and dispatchable electricity technologies be compared (Joskow, 2011). Joskow argues that the levelized cost approach to doing this is flawed and that it overstates the comparative private costs of switching from fossil to solar and wind power to supply electricity. The levelized cost method has been applied by a number of organizations including the United States Energy Information Administration (2010). Joskow (2011) maintains that it would be preferable to compare the private profitability of the alternative technologies and that this would be more favorable to the solar alternatives provided that the price of electricity from solar fully reflects the variations in its market value as the demand for electricity fluctuates.
He concludes that when this is done, the private cost of reducing CO2 emissions by switching electricity production from fossil fuels to solar is less than otherwise. However, neither approach is very satisfactory. For example, planning and decommissioning costs are not accounted for in either estimates. Furthermore, the social costs and benefits of transiting from fossil fuels to solar are not explored. In addition, Joskow (2011) does not take into account the possibility of storing electricity generated by solar and wind power. This may have been because battery storage of electricity was not sufficiently advanced when Joskow wrote.
Apart from the environmental consequences of using different techniques for electricity production, a related issue involves the available long-term supply of natural resources used for electricity production. For how long will the stock of economically recoverable non-renewable energy resources last? How scarce are renewable energy resources? What is the nature of their supply? Relevant literature relating to these aspects is referenced and discussed in the text.
As mentioned above, it is important to improve our classification of energy resources. Classifying these as either renewable or non-renewable is too crude and can be misleading (Tisdell, 2009). Some are stock resources and others are flow resources. Fossil fuels, biofuels and nuclear fuels are stock resources. Biofuels are renewable within limits but the other two energy sources are not. Solar, wind, and hydro can be regarded as flow resources and the challenge for humanity is to convert them economically into energy stocks to be drawn on when their available supply (which fluctuates) is insufficient to meet demands for power, e.g. electricity.
Table 1 provides a classification of basic resources used for producing electricity and includes comments on their renewability and the extent to which they can supply electricity in a flexible manner.
Classification | Comments |
Stock Resources | |
Fossil fuels (Coal, natural gas, oil) | Not renewable. Display varying degrees of dispatchability of electricity. |
Nuclear fuels (e.g. uranium) | Not renewable. Nuclear power plants generate fixed amounts of electricity. Possible to increase flexibility in supplying electricity by various storage methods. |
Partially Stocks and Flows | |
Organic matter (e.g. biogas) | Depletable but renewable. |
Combustible garbage wastes | Partially composed of organic matter plus material derived from fossil energy resources. |
Flows (Renewable) | |
Solar power | Not dispatchable but electricity may be converted to depletable stocks to supply dispatchable electricity, e.g. by use of batteries. |
Wind power | Same comment as above. |
Hydro(water) power e.g. rivers, streams, tidal | Relies on gravity. Can be converted to stocks by impounding water to increase the flexibility of its use for supplying electricity. Multiple uses of dams are often possible. |
Note: Other sources of energy for electricity production include wave power and heat released from inside the earth. These could be regarded as flow resources. |
Joskow (2011) considers solar and wind power to be intermittent resources and regards fossil fuels as dispatchable resources for electricity production. However, the dispatchability of electricity obtained from solar and wind power is increasing as a result of technological and other advances in the storage of electricity by using batteries.
Both solar and wind power can be regarded as having a finite amount of availability (they are relatively scarce in economic terms) (see, for example, Liski and Vehviläinen, 2017, for evidence of this in the Nordic area). Furthermore, the scarcity of these flow resources varies seasonally, is time-dependent, depends on weather conditions, and their availability differs regionally.
The adverse environmental externalities (spillovers) caused by the production of heat and power from fossil fuels are substantial, especially from the use of coal, but the production and use of biofuels can also have serious negative environmental effects (Tisdell, 2011). Less serious negative environmental effects are associated with the use of solar and wind power for energy production. Rough economic estimates of the environmental costs of using different energy resources to produce power (taking into account the nature of their utilization) can be made. However, up-to-date estimates which take account of recent and projected development of technologies for meeting energy demands are not available yet.
The ability of different energy resources to meet variations in the demand for electricity varies. Currently, the use of stock energy resources (excluding nuclear), primarily natural gas, coal, petroleum fuels, display greater flexibility in supplying electricity (they are more dispatchable) than does the use of solar and wind power, the supply of which is intermittent. The supply of solar and wind power depends on prevailing weather conditions. Solar is only available in the daytime and away from the equator, the length of daylight varies throughout the year. Atmospheric pollution (dust and haze) can also adversely affect solar electricity generation, as does cloud cover. Electricity production by nuclear power plants provides a continuous (non-variable) supply of electricity (Tisdell, 1997). At present, adjusting electricity supplies to demands requires a balance between the various sources of energy supplies. Phasing out the use of fossil fuels for electricity generation and replacing their supply by solar and wind power is restricted by the desire to supply sufficient electricity to meet demands when the supply of electricity from flow resources is insufficient to do this.
However, it is likely that greater use of battery storage (or other forms of retaining solar and wind power) will in the future, enable energy from these sources to be stored and to be available to meet the differences (that would otherwise occur) between the demand for electricity and its supply from these resources. These electricity storage technologies will be able to convert energy in flows into stocks of energy.2
2 These technologies may also be applied to other sources of electricity production, e.g. the output of nuclear plants, as a way of more closely aligning electricity availability with demand.
Although increases in the retail price of electricity could theoretically be used to reduce the demand for electricity when this exceeds its supply (and in some cases are), very large fluctuations in supply could cause considerable economic disruption. Political unrest is likely if such a policy is adopted given the high degree of dependence of contemporary societies on electricity for economic production and to meet personal needs. In modern societies, the demand for electricity is very inelastic, at least in the short-run and is likely to become even more so in the future. Consequently, an important constraint on the speed of transition from fossil fuels to solar and wind power for electricity supply is the availability of technologies able to economically convert energy from the latter resources from flows to stocks (Tisdell, 2009). In the future, it is likely that improved and new technologies could be developed that will facilitate this conversion process and make it more economical.
A variety of sustainability concerns have been expressed about the use of different resources to provide heat and power and the emphasis on these various concerns has altered with the passage of time. The following provides a sample of such concerns.
As the world began to depend more heavily on fossil fuels to meet its energy requirements, concern was expressed about the depletion of fossil fuels and the possibility of their scarcity increasing. For example, Jevons (1865) raised this concern in the 19th century. In the 1970s, the Club of Rome (Meadows et al., 1972) argued that a shortage of fossil fuels could imperil the sustainability of economic development. However, the Club of Rome underestimated the availability of fossil fuel resources, and did not take into account the availability of flow energy resources.
Global reserves of coal are extremely large. According to the World Coal Association (no date), proven economically renewable coal reserves are estimated to last for around 150 years, given current levels of consumption. Similar estimates for natural gas and oil availability are about 50 years (World Coal Association no date; Singh and Singh, 2012). However, the economical length of life of these energy resources is likely to be even longer than this. The duration of the economic life of the available reserves of these energy resources depends on many factors (Tisdell, 1982, 2009). More of these reserves are usually identified as their proven stocks decline. Technological change, alterations in the prices of energy derived from different sources, and other factors are also consequential for estimating how long fossil fuel resources will last.
As recently pointed out by Ritchie and Roser (no date), there is a global abundance of fossil fuels, even though the stocks of these resources are finite. They point out that from a climate change perspective, we should leave most of these fuels in the ground. They state: "If we are to have any chance of keeping global temperature increases below our 2 ℃ target, we have to leave the majority (up to 80 percent) of our fossil fuels in the ground" (Ritchie and Roser, no date).
In 2014, fossil fuels accounted for about 62% of world electricity production with the remainder coming from other sources, mainly hydro, nuclear, wind and solar power. Coal was the largest single contributor to electricity supply. Current predictions are for electricity production in the future to become less dependent on fossil fuels, especially coal, and for solar energy and wind power to considerably increase their contribution (Rutledge, 2011). However, as pointed out later in this article, this transition away from fossil fuels may not be frictionless because of the political obstacles raised by vested interests.
Currently, the main worldwide sustainability concern about using fossil fuels to produce power and energy is the impact of this on global warming and climate change. The latter environmental changes are a threat to global economic development, ecological, and social sustainability; as well as a threat to the sustainability of some regional economies.
However, even in the absence of climate change, the mining of fossil fuels raises significant sustainability issues. Communities which base their economies on the extraction of fossil fuels often have a limited period of existence because they often become economically unsustainable once the source of their wealth is exhausted.
Furthermore, limited reserves of some minerals used in battery production could restrict their use to store electricity. For example, the demand for lithium for battery production is rising but global reserves of lithium are believed by some researchers to be relatively small compared to the likely demand for these. However, opinions differ about how economically scarce lithium in-ground reserves are likely to become (Hunt, 2015; Narins, 2017; The Greenage, 2017). Furthermore, there is still much unrealized scope for recycling the lithium in batteries and extending the length of useful life of lithium-based batteries, including for them to have second applications. Nevertheless, there are important economic and organizational hurdles which need to be more satisfactorily addressed to achieve greater lithium recycling (King et al., 2018). It has also been estimated that resources for producing other types of batteries, e.g. lead and zinc, could become economically scarce in the foreseeable future (Mohr et al., 2018).
It is likely that as a result of R & D, different types of batteries to the existing ones and forms of storing solar and wind power will be developed and that current battery and other power storage technologies might be made more efficient. Consequently, constraints on storing energy will become less severe than they might otherwise be. Nevertheless, the sustainability of local communities reliant on the extraction of minerals for battery production will still be an issue.
Several financial instruments are available to governments to foster the substitution of wind and solar energy for power derived from fossil fuels. These can be divided into demand-side and supply-side economic incentives. Let us consider some of these incentives.
Purchases of electricity (from the grid) derived from solar and wind power can be subsidized by governments. This subsidy can be distributed to buyers by electricity retailers on behalf of the government. This last mentioned type of policy has been adopted by some Australian State Governments but some have phased out this approach. The size of the subsidy can be varied depending on the amount of economic stimulus considered to be desirable for fostering the switch to wind and solar power. Indirectly, this policy provides an economic gain to producers of electricity from solar and wind power. This is because it stimulates the demand for this type of green electricity and may raise the effective price they receive for their sales of electricity. If the supply curves for these types of green energy have normal slopes (are neither perfectly inelastic nor completely elastic) the desired substitution will occur and the effective price received for green energy supplies will rise in the short to medium term. This is so given that from the point of view of most buyers of electricity, green electricity is highly substitutable for non-green electricity.
It is of course, important that subsidization discriminates in favor of green electricity, that is, that it not be offset by subsidies for the use of non-green electricity. Any subsidies for the latter could also be reduced. Furthermore, the fraction of green electricity purchases eligible for a subsidy can be varied.
Green electricity producers can be paid a subsidy on the value of their sales (via the grid) of electricity derived from solar and wind power. Other things being held constant, this is likely to increase their competitiveness with suppliers of non-green electricity. Normally, this will lower the market price for green electricity and may raise the economic surplus of suppliers of green energy, as is illustrated in the next section of this article. The level of the subsidy can be varied to manage the transition to greater reliance on wind power and solar power for electricity production.
Government financial support can also be provided for investment in equipment for electricity production derived from solar and wind power. Possibilities include subsidies for the purchase of equipment or tax concessions, for example, accelerated depreciation allowances and "soft" loans.
Furthermore, R & D can help improve the development of the green energy sector and governments can provide support for these activities. The main research and development areas worthy of support seem to be those
1. For developing more efficient and economical methods of generating electricity from solar and wind power;
2. Those for discovering improved ways of storing electricity (or energy) obtained from solar and wind power; and
3. Those for the development of more efficient and environmentally friendly ways of recycling material in batteries and extending their useful life.
Government support for these types of R & D can be justified because the negative environmental externalities associated with the use of those forms of green power are considerably less than for power derived from fossil fuels, and their use has superior sustainability consequences.
It is worthwhile giving attention to the economic analysis of why it is socially desirable to provide government financial support for the production of electricity from solar and wind power. The basic reasons are:
1. The negative environmental spillovers from the use of fossil fuels to produce electricity considerably exceed those from the generation of electricity by using solar or wind power; and
2. Dependence on the latter resources for electricity production results in better sustainability outcomes than dependence on the former resources.
Note that the threat to sustainability of relying on fossil fuels to generate electricity arises in part because their use produces larger negative environmental spillovers than does dependence on solar and wind power. However, there are also negative environmental effects from the use of fossil fuels, especially coal, which are independent of the sustainability of the supply of these fuels. These include negative health effects, e.g. respiratory diseases arising from air pollution caused by fossil fuels. These can be severe.
The negative environmental spillovers associated with the production of electricity using wind and solar power tend to be low. However, the environmental consequences of this production depend on the location of the production units. For example, if solar panels are located on buildings (used for purposes other than supplying solar energy), their environmental impact is likely to be lower than when they are located on solar farms. In the latter case, significant removal of vegetation may occur, and existing ecosystems are altered. 3
3 In some cases, visual amenity is negatively impacted. Also solar farms located on valuable agricultural land (or land valuable for alternative uses) can have a high economic opportunity cost.
The whole chain of electricity production and distribution needs to be considered when assessing the environmental impacts of alternative forms of producing electricity. For example, the environmental effects of manufacturing equipment for producing electricity for solar and wind power should be taken into account. When this is done, the environmental costs of supplying electricity from solar and wind power are likely to be much lower than using fossil fuels, particularly coal, for this purpose. For example, the environmental costs of mining and transporting coal are often high and coal combustion adds considerably to air pollution and global warming. However, it might be noted that there are also likely to be some negative environmental consequences from the supply of batteries intended to store electricity generated by solar and wind power. Furthermore, the whole life cycle of units involved in electrical supply needs to be taken into account when assessing environmental costs. These include those costs associated with their decommissioning. The full extent of all these environmental costs have yet to be measured.
The environmental costs involved in battery production include those which result from mining materials for batteries, their manufacture, the recycling of the components of batteries and in many cases, the disposal of batteries in solid waste (King et al., 2018; Messenger, 2018). A high proportion of lithium batteries are dumped as solid waste globally and the percentage is high in Australia (King, et al., 2018). There is some risk of water and soil contamination as a result. Furthermore, there are environmental costs involved in mining and processing lithium for use in lithium-ion batteries, as is indicated by Draper (2019). This is so whether the lithium is obtained from hard-rock minerals by physical extraction and chemical processing, or from underground lithium-bearing water bodies. In the latter case, the water is evaporated and the resulting concentrate is then chemically processed to obtain lithium. At the other end of the product chain (product life cycle) one also has to consider the cost of disposing of solar panels once they reach the end of their useful life. Nevertheless, the environmental costs of relying on a combination of solar and wind power for electricity production and storage using batteries can be expected to be substantially lower than relying on fossil fuels, especially coal, for this purpose.
The import of the above is that it would be a folly to argue that there are no environmental costs associated with the supply of electricity by relying on solar or wind power. Nevertheless, these costs are likely to be much less than relying on fossil fuels to supply electricity. The modeling which follows is based on the assumption that the sustainability and environmental disadvantages of obtaining electricity supplies from solar and wind power are less than those from its supply by the use of fossil fuels.
Figure 2 provides an analytical illustration (example) of the increase in social benefits which can be attained by subsidizing the supply of electricity obtained from the use of solar and wind power.4 Line DD represents the demand for this type of electricity and line AS indicates the private supply (equals the private marginal cost) of electricity produced from solar and wind power. It is assumed that the extra social marginal benefit of supplying electricity from solar and wind power compared to supplying it from fossil fuels, is equivalent to AB dollars per unit of energy produced. Taking this into account, the marginal social cost of supplying electricity from solar and wind power is shown by the line BSʹ. There may still be some negative externalities from the supply of electricity from solar and wind but these are supposed to be negligible. Note that the comparative social economic benefit of supplying electricity from solar and wind power (rather than fossil fuel) could include an allowance not only for the lower environmental spillovers involved but also for the better sustainability outcomes associated with the use of solar and wind power.
4 It is supposed that at this stage of development, electricity production from solar and wind power (particularly the former) is still in the infant industry phase. However, electricity supply from these sources is becoming increasingly competitive with the supply of fossil fuels in some parts of the world.
In the absence of a subsidy for the supply of electricity from solar and wind power, the market for this source of electricity in the case shown in Figure 2, comes into equilibrium at E1. The price per unit of this electricity is then P1 and its market supply is X1. However, this is not a socially ideal situation. A superior outcome would be for this market to be in equilibrium at E2. This would result in additional social economic benefits indicated by the area of the hatched quadrilateral. This could be achieved by paying a subsidy of AB dollars per unit on all electricity produced by solar and wind power and sold on the market.
The subsidy paid to suppliers of electricity obtained from solar and wind power could be interpreted as being a payment for environmental services provided by this economic sector. Nevertheless, the economic benefits of this subsidy in the case illustrated (which is a normal case) are distributed in the short-run between buyers of green electricity and suppliers of it. As a result of the subsidy, the buyer's economic surplus rises by an amount equivalent to the area of the quadrilateral P1P2E1E2. Prior to the subsidy, the economic surplus of suppliers of green electricity is equal to the area of triangle AE1P1. After the subsidy, it is equal to the area of triangle P2BE2. The latter triangle is greater in area than the former one because the distance BP2 exceeds AP1 and also the distance P2E2 exceeds P1E1. Market forces influencing the incidence of the subsidy are similar to those of a tax on production (see Tisdell and Hartley, 2008).
It is possible to pay a smaller amount of subsidy to producers of green electricity and still reach the short run market equilibrium corresponding to E2 in Figure 2. This can be achieved by paying a subsidy per unit of output which rises with the volume of production. For example, if a miserly payment is desired in the case illustrated in Figure 2, the subsidy on each unit of production could be paid on each unit of output greater than X0, rising by an amount on each additional unit of production equal to the difference between the line GK and GE2. However, this can result in green electricity producers having a lower economic surplus than before the subsidy (compare Duncan and Tisdell, 1971). The negative effect of this miserly policy (or a similar one) is that by failing to raise the economic surplus of supplies of green electricity, it reduces their incentive to invest in the development of this sector and restrains the entry of new firms into this sector.
Taking this observation into account, it can be concluded that the use of comparative static market analysis to analyze the social desirability of subsidizing the supply of green electricity provides a limited policy perspective. This is because it does not pay enough attention to economic dynamics. Nevertheless, static equilibrium analysis is widely relied on, for example, by Liski and Vehviläinen (2017).
In my view, infant industry considerations (List, 1856) provide the strongest case for government financial support for the development of green electricity production. Eventually, this support is likely to be no longer necessary once the green electricity sector has developed sufficiently. As this sector becomes increasingly self-reliant economically, the sustainability and environmental externality benefits of greater dependence on green electricity will then flow on to the population at large.
Nevertheless, in transiting to a greater reliance on green power for electricity production, the reliability of the whole electricity system must be taken into account, that is, its ability to meet fluctuating electricity demands. In the absence of adequate storage facilities for energy derived from solar and wind power, increasing reliance on these energy sources reaches a point (if electricity is supplied via the grid system) where the system can have insufficient electricity to always meet demands. As a result, blackouts and brown outs can occur.
There are other ways that governments could encourage the use of solar and wind power for supplying electricity. These include the imposition of taxes on the supply or purchase of electricity obtained from fossil fuels and the use of tradeable permit systems for the generation of electricity from these sources. In normal circumstances, these will increase the price of electricity to buyers and may result in a political backlash from buyers and suppliers of electricity generated by the use of fossil fuels. Therefore, even if there is an economic case for making the polluter pay, politics may limit the adoption of this approach. Vested special interests can be expected to lobby against it. However, as the green energy sector expands and the fossil fuel sector declines, the balance of political power can be expected to alter in favor of green energy. In practice, economic policies cannot be divorced from political realities.
The question has also arisen of whether or not users of electricity should continue to depend on the electricity grid for their supplies. In some regions, it is possible for some households and businesses to go off grid and collect solar power by relying on solar panels and storing electricity by using batteries. This tends to make electricity grids less economic and can impose extra costs on those who continue to rely on electricity grids for their supply of electricity, including households and businesses that buy and sell solar energy via the grid. The economics of off-grid self-efficiency in electricity supplies requires future research.
The following are some of the innovative or important highlights of this article:
1. It identifies significant gaps and shortcomings in the existing literature about the social economic desirability of relying on fossil fuels rather than solar and wind power to supply electricity.
2. It proposes a taxonomy of natural energy resources used for electricity production which classifies these as stock or flow resources. It is argued that this is more appropriate than only trying to categorize them as renewable or non-renewable resources.
3. It points out that new technologies for storing electricity have developed rapidly and are becoming more economical, e.g., the use of lithium-ion batteries. This has important implications for the development of the electricity sector. These implications are not yet fully accounted for in the academic literature. For instance, electricity obtained from intermittent sources, e.g., solar and wind power (flow resources) can be converted to a stock. Consequently, electricity from these sources has become easily dispatchable. Furthermore, it is becoming more economic to adopt this storage technique.
4. The economic availability of natural resources for producing electricity can constrain the growth and sustainability of this supply. Estimates of how long fossil fuels will be economically available to supply electricity were discussed. The difficulties of determining this was highlighted as well as different and conflicting views which have been expressed in the literature about their long-term availability.
5. It was pointed out that the development of the solar and wind energy sector might be constrained by future scarcity of raw materials used in battery production, e.g., lithium. This aspect needs more research. So far, it has received little attention in the relevant literature.
6. A significant limitation of much of the current literature which assesses the comparative social desirability of using fossil fuels to generate electricity rather than solar or wind power is its focus only on the environmental consequences of using these resources at the final stage of electricity production. Furthermore, it is often only their contribution to greenhouse gas emissions which is considered. It is argued that scientifically a more holistic approach is needed. Ideally, environmental effects along the whole product chain, inter-industry environmental consequences, and the full environmental life-cycle of alternative techniques should be taken into account in determining the social desirability of adopting alternative techniques for electricity supply. However, it is recognized that bounded rationality may limit the extent to which this can be done.
7. It was shown that some methods used in the economic literature for comparing the value of relying on fossil fuels rather than alternatives for the supply of electricity are inadequate from a social economics point of view. In particular, this is true of levelized-cost comparisons and private profitability comparisons.
8. It was demonstrated how static economic modeling can be applied to strengthen the social case for subsidizing the development and use of solar and wind power for electricity production. However, this type of analysis does not take account of infant-industry dynamics. Infant-industry arguments further strengthen the case for government financially supporting the development of the use of solar and wind power for electricity generation.
9. It was pointed out that all the available techniques for electricity have some negative environmental consequence. This includes electricity supplied from renewable resources. A holistic and balanced assessment requires all of these consequences to be identified and taken into account.
This short article has presented a case in favor of governments providing financial support for the increased use of solar and wind power as a means for supplying electricity. In doing so, it has suggested an improved classification of energy resources for sustainability assessments, and has identified the nature of sustainability concerns about the use of different energy resources for generating heat and power, particularly electricity. In this regard, it has focused on the use of solar and wind power in comparison to the use of fossil fuels, especially coal. Different types of government financial incentives which can be employed to increase the demand and supply of electricity from solar and wind power were outlined. Furthermore, an illustrative example was provided of the social economic case for subsidizing the supply of electricity obtained from solar and wind power.
Nevertheless, at present, there are some limitations on the extent to which it is possible to rely on the supply of electricity from solar and wind power. Storage constraints are one such restriction but these are being overcome. Also some regions of the world are not well placed to produce electricity from solar and wind power. In some areas, wind velocities are low and unreliable, and while some regions are blessed with a lot of regular sunlight, this is not so in all places on earth. Solar and wind power resources are geographically unevenly distributed. The extent to which regions can import green electricity also varies. These factors all limit the ability of humankind to depend on solar and wind energy for their electricity requirements. Despite this, there is still considerable scope in many countries and regions to make increased use of solar and wind power for electricity production and thereby raise social welfare by making economic development more sustainable and reducing the magnitude of adverse environmental externalities (costs) associated with electricity production.
This research did not receive any specific grant from funding agencies in the public, commercial or not-for-profit sectors. I am grateful to Professor Tooraj Jamasb, Durham University, UK for bringing some relevant references to my attention. The author wishes to thank the reviewers for their constructive comments on the first draft of this article.
I declare no conflict of interest in this paper.
[1] |
Acemoglu D, Akcigit U, Hanley D, et al. (2016) Transition to clean technology. J Polit Econ 124: 52–104. doi: 10.1086/684511
![]() |
[2] |
Borenstein S (2012) The private and public economics of renewable electricity generation. J Econ Perspect 26: 67–92. doi: 10.1257/jep.26.1.67
![]() |
[3] | Draper R (2019) The rush for white gold. Natl Geogr 235: 78–103. |
[4] |
Duncan R, Tisdell CA (1971) Research and technical progress: the returns to producers. Econ Rec 47: 124–129. doi: 10.1111/j.1475-4932.1971.tb00752.x
![]() |
[5] |
Fabra N, Reguant M (2014) Pass-through of emissions costs in electricity markets. Am Econ Rev 104: 2872–2899. doi: 10.1257/aer.104.9.2872
![]() |
[6] | Hunt T (2019) Is There Enough Lithium to Maintain the Growth of the Lithium-Ion Battery Market? Are we nearing peak lithium? Greentech Media 2015. Available from: https://www.greentechmedia.com/articles/read/is-there-enough-lithium-to-maintain-the-growth-of-the-lithium-ion-battery-m#gs.IGpbj3tI. |
[7] | Jevons WS (1865) The Coal Question, London: Macmillan. |
[8] |
Joskow PL (2011) Comparing the Costs of Intermittent and Dispatchable Electricity Generating Technologies. Am Econ Rev 101: 238–241. doi: 10.1257/aer.101.3.238
![]() |
[9] | King S, Boxall NJ, Bhatt A (2018) Lithium battery recycling in Australia. Available from: https://www.csiro.au/~/media/EF/Files/Lithium-battery-recycling-in Australia.PDF?la=en&hash = 924B789725A3B3319BB40FDA20F416EB2FA4F320. |
[10] | Liski M, Vehviläinen I (2017) Gone with the Wind? An Empirical Analysis of the Renewable Energy Rent Transfer. Cambridge: University of Cambridge. |
[11] | List F (1856) Das Nationale System der politischen Oekonomie (The National System of Political Economy), Translated by G. A. Matile, Philadelphia, USA: J B Lippencott and Co. |
[12] | Meadows D, Ronders HJ, Behrens W (1972) The Limits of Growth: A Report for the Club of Rome's Project on the Predicament of Mankind, New York: Universe Books. |
[13] | Messenger B (2019) In depth: Lithium battery recycling-the clean energy clean up. Waste Management World 2018. Available from https://waste-management-world.com/a/in-depth-lithium-battery-recycling-the-clean-energy-clean-up. |
[14] |
Mohr S, Giurco D, Retamal M, et al. (2018) Global projection of lead-zinc supply from known resources. Resources 7: 17. doi: 10.3390/resources7010017
![]() |
[15] | Narins TP (2017) The battery business: Lithium availability and the growth of the global electric car industry. Extr Ind Soc 4: 321–328. |
[16] | Ritchie H, Roser M (2018) Fossil fuels. Our World in Data no date. Available from https://ourworldindata.org/fossil-fuels. |
[17] |
Rutledge D (2011) Estimating long-term world coal production with logit and probit transforms. Int J Coal Geol 85: 23–33. doi: 10.1016/j.coal.2010.10.012
![]() |
[18] | Singh BR, Singh O (2012) Global change of fossil fuel reserves adn climate change in the 21st Century, In Fossil Fuel and the Environment, edited by S. Kahn, London: IntechOpen. |
[19] | The Greenage (2019) Are we facing a lithium shortage? The Greenage 2017. Available from https://www.thegreenage.co.uk/facing-lithium-shortage/. |
[20] | Tisdell CA (1982) Microeconomics of Markets, Brisbane: John Wiley. |
[21] |
Tisdell CA (1997) The Environment and Sustainable Development in the Asia-Pacific: Views and Policies. Asian-Pac Econ Lit 11: 39–55. doi: 10.1111/1467-8411.00003
![]() |
[22] | Tisdell CA (2009) Resource and Environmental Economics: Modern Issues and Applications, Hackensack (NJ), London and Singapore: World Scientific. |
[23] | Tisdell CA (2011) The production of biofuels: Welfare and environmental consequences for Asia, In Climate Change and Growth in Asia, edited by M. Hossain and E. Selvanathan. Cheltenham, UK and Northampton, MA, USA: Edward Elgar. |
[24] | Tisdell CA, Hartley K (2008) Microeconomic Policy: A New Perspective, Cheltenham, UK and Northampton, MA, USA: Edward Elgar. |
[25] | United States Energy Administration (2010) Annual Energy Outlook 2010, Washington DC: United States Energy Administration. |
[26] | White A (2018) Canberra's big stick belts gentailers as nimble rivals rush to invest. The Australian, Dec 25, 13–14. |
[27] |
Whitmee S, Haines A, Beyrer C, et al. (2015) Safeguarding human health in the Anthropocene epoch: report of The Rockefeller Foundation-Lancet Commission on planetary health. Lancet 386: 1973–2028. doi: 10.1016/S0140-6736(15)60901-1
![]() |
[28] | World Coal Association (2018) Where is coal found? no date. Available from https://www.worldcoal.org/coal/where-coal-found. |
1. | D. Hemanand, Nilamadhab Mishra, G. Premalatha, Dinesh Mavaluru, Amit Vajpayee, Sumit Kushwaha, Kibebe Sahile, Vijay Kumar, Applications of Intelligent Model to Analyze the Green Finance for Environmental Development in the Context of Artificial Intelligence, 2022, 2022, 1687-5273, 1, 10.1155/2022/2977824 | |
2. | Junhong Qu, Xiaoli Hao, Digital Economy, Financial Development, and Energy Poverty Based on Mediating Effects and a Spatial Autocorrelation Model, 2022, 14, 2071-1050, 9206, 10.3390/su14159206 | |
3. | Yun Liu, Meng Liu, Gege Wang, Lulu Zhao, Pan An, Effect of Environmental Regulation on High-quality Economic Development in China—An Empirical Analysis Based on Dynamic Spatial Durbin Model, 2021, 28, 0944-1344, 54661, 10.1007/s11356-021-13780-2 | |
4. | Muhammad Azam, Muhammad Haroon, Saeed ur Rahman, Hammad Ali, Muhammad Irfan Chani, 2024, Chapter 2, 978-3-031-65755-9, 19, 10.1007/978-3-031-65756-6_2 | |
5. | Xialing Sun, Zheng Meng, Xuchao Zhang, Jiaxi Wu, The role of institutional quality in the nexus between green financing and sustainable development, 2025, 73, 02755319, 102531, 10.1016/j.ribaf.2024.102531 | |
6. | Qiao Zeng, Caixia Li, Cosimo Magazzino, Impact of green energy production for sustainable economic growth and green economic recovery, 2024, 10, 24058440, e36643, 10.1016/j.heliyon.2024.e36643 | |
7. | Nura Garba, Bashir Abdulrahman, Renewable Energy Sources, Sustainability and Environmental Protection: A Review, 2024, 2, 2786-7447, 449, 10.59324/ejtas.2024.2(2).39 | |
8. | Minqiang Zhang, Jia Hou, Yishu Liu, Achieving development goals via digital government strategies for a sustainable digital economy that integrate natural resource governance and energy security, 2025, 101, 03014207, 105330, 10.1016/j.resourpol.2024.105330 | |
9. | Yu Han, Xiaopan Li, Yu Zhang, Nang Goi, Balancing economic growth and ecological sustainability: Factors affecting the development of renewable energy in developing countries, 2025, 116, 03603199, 601, 10.1016/j.ijhydene.2025.02.277 | |
10. | Yuwen Wu, Liu Ziqi, Are advancements in renewable energy technologies being driven by the digital economy and financial structure? An overview of the key eight nations, 2025, 59, 2211467X, 101679, 10.1016/j.esr.2025.101679 | |
11. | Yunsheng Ruan, Wenqing Wang, Muhammad Abubakar, Nazeer Ahmad, Global Economic Resilience: Developing Green Growth Strategies, Renewable Energy Integration, and Environmental Economics for Sustainability, 2025, 09601481, 123341, 10.1016/j.renene.2025.123341 |
Classification | Comments |
Stock Resources | |
Fossil fuels (Coal, natural gas, oil) | Not renewable. Display varying degrees of dispatchability of electricity. |
Nuclear fuels (e.g. uranium) | Not renewable. Nuclear power plants generate fixed amounts of electricity. Possible to increase flexibility in supplying electricity by various storage methods. |
Partially Stocks and Flows | |
Organic matter (e.g. biogas) | Depletable but renewable. |
Combustible garbage wastes | Partially composed of organic matter plus material derived from fossil energy resources. |
Flows (Renewable) | |
Solar power | Not dispatchable but electricity may be converted to depletable stocks to supply dispatchable electricity, e.g. by use of batteries. |
Wind power | Same comment as above. |
Hydro(water) power e.g. rivers, streams, tidal | Relies on gravity. Can be converted to stocks by impounding water to increase the flexibility of its use for supplying electricity. Multiple uses of dams are often possible. |
Note: Other sources of energy for electricity production include wave power and heat released from inside the earth. These could be regarded as flow resources. |
Classification | Comments |
Stock Resources | |
Fossil fuels (Coal, natural gas, oil) | Not renewable. Display varying degrees of dispatchability of electricity. |
Nuclear fuels (e.g. uranium) | Not renewable. Nuclear power plants generate fixed amounts of electricity. Possible to increase flexibility in supplying electricity by various storage methods. |
Partially Stocks and Flows | |
Organic matter (e.g. biogas) | Depletable but renewable. |
Combustible garbage wastes | Partially composed of organic matter plus material derived from fossil energy resources. |
Flows (Renewable) | |
Solar power | Not dispatchable but electricity may be converted to depletable stocks to supply dispatchable electricity, e.g. by use of batteries. |
Wind power | Same comment as above. |
Hydro(water) power e.g. rivers, streams, tidal | Relies on gravity. Can be converted to stocks by impounding water to increase the flexibility of its use for supplying electricity. Multiple uses of dams are often possible. |
Note: Other sources of energy for electricity production include wave power and heat released from inside the earth. These could be regarded as flow resources. |