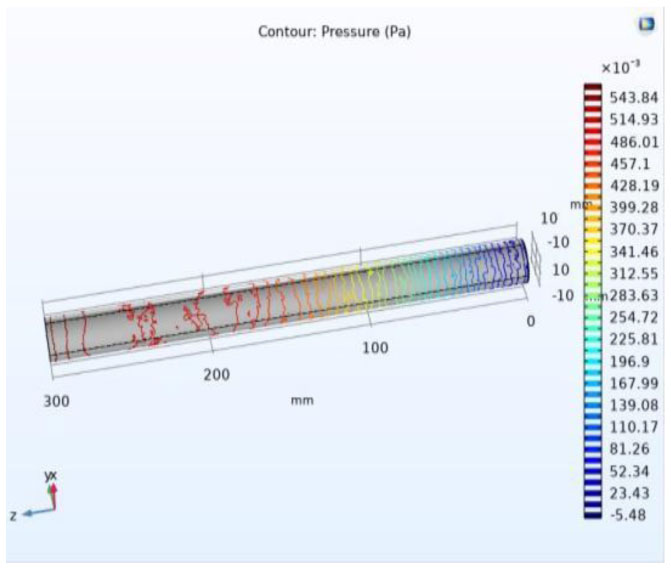
The primary objective of the research article is to describe the functionality of wrist watch which acts as a digital stethoscope to measure health constraints such as heart pulse through blood pressure. The second objective is the detection of blockages of an artery due to fatty, cholesterol-deposited, over a period when the blood is passing through due to stress and exercise, etc. Pressure and velocity are two inputs with their respective results of contrast and expansion of veins at the outputs. The major parameters in detecting the laminar flow are pressure and velocity. These parameters of a vein are analyzed by integrating valves in the vein. The movement of blood laminar flow in the vein is captured by a MEMS-based piezoelectric sensor by its functionality. The proposed design performance accuracy is estimated by modeling of vein's laminar flow when blood is circulating. The coagulation effect of the vein is used to measure heart stroke by placing MEMS along with the stent, as MEMS are tiny in size. The functionality of a digital Stethoscope works on the piezoelectric effect that generates an electrical signal when pressure is applied from the vein. The accuracy, functionality, and performance of the design can be analyzed by COMSOL multi-physics. Applications of MEMS include detection, prevention, and alert during the second heart stroke, and also used in the bionic eye and automotive electronics.
Citation: Vasudeva Reddy Tatiparthi, Madhava Rao, Santosh Kumar, Hindumathi. Detection and analysis of coagulation effect in vein using MEMS laminar flow for the early heart stroke diagnosis[J]. AIMS Bioengineering, 2023, 10(1): 1-12. doi: 10.3934/bioeng.2023001
[1] | Kuna Dhananjay Rao, Mudunuru Satya Dev Kumar, Paidi Pavani, Darapureddy Akshitha, Kagitha Nagamaleswara Rao, Hafiz Tayyab Rauf, Mohamed Sharaf . Cardiovascular disease prediction using hyperparameters-tuned LSTM considering COVID-19 with experimental validation. AIMS Bioengineering, 2023, 10(3): 265-282. doi: 10.3934/bioeng.2023017 |
[2] | Poovayar Priya Mohan, Ezhilarasan Murugesan . A novel approach for detecting the presence of a lymphatic rosary in the iris using a 3-stage algorithm. AIMS Bioengineering, 2024, 11(4): 506-526. doi: 10.3934/bioeng.2024023 |
[3] | Torsten Schüling, Alina Eilers, Thomas Scheper, Johanna Walter . Aptamer-based lateral flow assays. AIMS Bioengineering, 2018, 5(2): 78-102. doi: 10.3934/bioeng.2018.2.78 |
[4] | Daniel N. Riahi, Saulo Orizaga . Modeling and computation for unsteady blood flow and solute concentration in a constricted porous artery. AIMS Bioengineering, 2023, 10(1): 67-88. doi: 10.3934/bioeng.2023007 |
[5] | Naeimeh Faraji, Yan Zhang, Ajay K. Ray . Impact of operating conditions on chromatographic column performance: experimental studies on adsorption of high-value minor whey proteins. AIMS Bioengineering, 2017, 4(2): 223-238. doi: 10.3934/bioeng.2017.2.223 |
[6] | Sumaiya Noor, Salman A. AlQahtani, Salman Khan . Chronic liver disease detection using ranking and projection-based feature optimization with deep learning. AIMS Bioengineering, 2025, 12(1): 50-68. doi: 10.3934/bioeng.2025003 |
[7] | Xiao-Li Gu, Li Yu, Yu Du, Xiu-Peng Yang, Yong-Gang Xu . Identification and validation of aging-related genes and their classification models based on myelodysplastic syndromes. AIMS Bioengineering, 2023, 10(4): 440-465. doi: 10.3934/bioeng.2023026 |
[8] | Jingwen Zhang, Lu Wang, Huolin Chen, Tieying Yin, Yanqun Teng, Kang Zhang, Donghong Yu, Guixue Wang . Effect of Caspase Inhibitor Ac-DEVD-CHO on Apoptosis of Vascular Smooth Muscle Cells Induced by Artesunate. AIMS Bioengineering, 2014, 1(1): 13-24. doi: 10.3934/bioeng.2014.1.13 |
[9] | Shital Hajare, Rajendra Rewatkar, K.T.V. Reddy . Design of an iterative method for enhanced early prediction of acute coronary syndrome using XAI analysis. AIMS Bioengineering, 2024, 11(3): 301-322. doi: 10.3934/bioeng.2024016 |
[10] | Guang Long, Bo Lin, Lu Wang, Lingyan Wu, Tieying Yin, Donghong Yu, Guixue Wang . Sappan Lignum Extract Inhibits Restenosis in the Injured Artery through the Deactivation of Nuclear Factor-κB. AIMS Bioengineering, 2014, 1(1): 25-39. doi: 10.3934/bioeng.2014.1.25 |
The primary objective of the research article is to describe the functionality of wrist watch which acts as a digital stethoscope to measure health constraints such as heart pulse through blood pressure. The second objective is the detection of blockages of an artery due to fatty, cholesterol-deposited, over a period when the blood is passing through due to stress and exercise, etc. Pressure and velocity are two inputs with their respective results of contrast and expansion of veins at the outputs. The major parameters in detecting the laminar flow are pressure and velocity. These parameters of a vein are analyzed by integrating valves in the vein. The movement of blood laminar flow in the vein is captured by a MEMS-based piezoelectric sensor by its functionality. The proposed design performance accuracy is estimated by modeling of vein's laminar flow when blood is circulating. The coagulation effect of the vein is used to measure heart stroke by placing MEMS along with the stent, as MEMS are tiny in size. The functionality of a digital Stethoscope works on the piezoelectric effect that generates an electrical signal when pressure is applied from the vein. The accuracy, functionality, and performance of the design can be analyzed by COMSOL multi-physics. Applications of MEMS include detection, prevention, and alert during the second heart stroke, and also used in the bionic eye and automotive electronics.
A Micro-Electro-Mechanical System (MEMS) is a miniaturized device with a combination of electronic features built on a mechanical structure. The basic functionality of MEMS is to act as a switch and also act as a transducer. In the structural view, MEMS consists of a mass suspended between two capacitive plates. This makes a difference in electric potential. MEMS sizes range from micro to millimeters [1]. The MEMS sensor model is tiny and applicable to measuring all portable consumer electronics, such as blood pressure, heartbeat, and flow measurement. Vein expansion and contract are analyzed under different degrees of freedom of design. Modeling, simulation, and verification are done using laminar flow analysis to measure the heart stroke in the early stage. A few objectives of the research article include the design of different shapes of the vein to calculate the different pressure and velocity across values. Another objective indicates the design of a vein network using vein blockages using valves to analyze the result. One more objective represents making a fifty percent open path of a vein by semi-close of vein for better analysis. The final objective designates pressure across the vein, in other words, diastole and systole are captured by a piezoelectric sensor.
Laminar flow analysis is the process of allowing or passing liquid or gas through the pipe without any obstacle across it. A single vein is considered to measure the pressure and velocity of blood across. Analyzing pressure with the straight vein structure is described in Figure 1. Laminar flow analysis of different shaped veins is created and analyzed. A few models designed in this article are straight pipe structures, L-type, U-type, and T shaped. The blood flow analysis is considered according to its inlet and outlet.
Laminar flow analysis [2] allows blood to flow smoothly across it. Position 1 and 2 of the vein are treated as inlet and outlet, respectively. The vein is modeled using COMSOL multi-physics. After the pressure analysis at the inlet, the position usually is higher than at the outlet. Simulation results in Figure 1 indicate the pressure applied at the inlet is 0.09 nw decreased to −5.48 × 10−3 Pascal's at the outlet. Blood velocity concentration in laminar flow is indicated in Figure 2. Under high pressure, velocity is more at the center position, there will be less across boundary walls. At inlet center position indicates the red mark at the boundary walls marked blue outlet. Figure 3 with an internal view, represents the red-blue marks. The analysis shows that the concentration is more at the center and less at the outer surface [3].
Velocity at different places is indicated in Figure 3. Velocity is more at the center than the boundaries [4]. The conclusion for a straight line/pipe is that when pressure is applied, the velocity is 0.07 m/s at the center and 0.01 m/s at the boundaries as the most negligible value.
The L-type vein is created using COMSOL multiphysics, considering its inlet and outlet. Equal pressure is applied at the inlet, measuring the pressure response across the output. Fully open does not require any valves. Partially open states have been designed by applying a valve across the vein. A partial state represents the 50% open and 50% closed state, and a fully open state indicates the completely open. Figure 4 indicates the modeling of L-type vein design using COMSOL laminar flow analysis is done for the smooth representation of pressure and velocity of blood across the vein.
Fully open: when the shape is changed, the pressure gets affected. A change in pressure can be observed in Figure 4. at the bending point. Pressure at the instant is 0.05108 Pascal's, which is less than the pressure applied at the inlet. Figure 5 indicates the modeling of veins and analysis of pressure at different positions. When there were two blockages across the vein with partially open states represented in Figure 6.
There will be a blockage of the vein whenever clots are formed due to cholesterol. This phenomenon is treated as a coagulation effect. During this instant, the pressure and velocity of the blood in the vein change, and the same is modeled geometrically designed by inserting valves at various places with 50% open as indicated in Figure 7 [5]. The L-shaped vein with two blockages indicates the color change and represents the change in pressure or velocity. Red-marked pressure increases when a blockage comes in the middle of the laminar flow. A portion marked with blue color indicates increased velocity [6]. Changes that occur when the vein is 50% closed are visible in Figure 7. A portion marked with blue color indicates increased velocity [6].
Figure 8 represents the fully open path without any obstacles across it and the type of vein indicates U shaped vein. Pressure and velocity can be easily measured when the flow is continuous. across the U-shaped vein, the pressure decreased near the bending and more at the outlet. Figure 9 indicates the velocity, and Figure 10 shows that the pressure analysis can be verified.
Figure 10 indicates the design of a U-shaped vein with two blockages in the path. The design is verified before the mesh and geometry analysis are finished. The pressure applied at the input is 0.09 Pascal's at the inlet. However, parameters will be affected due to the coagulation effect as velocity and pressure are inversely proportional in this case by comparing Figure 10. Moreover, in Figure 11 are compared, we know that at the blockage point, the pressure decreases and velocity increases [7].
At the divisional point, the pressure gets reduced, so at both outlets, the pressure decreases to 0.0557 Pascal's. The speed gets reduced when velocity is more at the center of the inlet at the center and outer surface. The main reason for velocity reduction is the flow breakage due to division in the path, as shown in Figure 13. A divisional flow of blood pressure is a briefing in the T-shaped vein, where the pressure and velocity equally split into two outlets, as shown in Figure 12.
The capacity of certain materials to “create an electric charge in response to mechanical stress” is known as the piezoelectric effect. The piezoelectric effect can also be reversible, which means materials that exhibit the direct piezoelectric effect, in other words, the creation of electricity when stress is applied, can also exhibit the opposite piezoelectric effect, which means the generation of stress when an electric field is applied. The concept of the inverse piezoelectric effect is applied, which produces acoustic waves using the inverse piezoelectric effect [8]. Red arrow marks represent the amount of pressure applied on one surface of the MEMS piezo, as shown in Figure 14.
Pressure from the vein is applied as input to MEMS piezoelectric sensor. When piezoelectric material is subjected to mechanical stress, positive and negative charge carriers accumulate in centers in material change, resulting in an external electrical field. The electrical area at the outer surface stretches or compresses when it reverses. These electrical signals detect patients suffering from secondary heart stroke conditions or abnormalities. The above analysis is based on the working of wristbands in digital stethoscopes [9]. Figure 15 indicates the functionality of the piezoelectric sensor. The design of the wristband with MEMS is represented in Figure 16. MEMS transducer, the electrical response for pressure is indicated with blue and red marks as the inlet and outlet are illustrated in Figure 17.
The data collected from the different blood pressure stages are considered to measure and analyze the blood pressure status indicated in Figure 18.
To select assumptions in COMSOL multiphysics.
The pressure of each model is evaluated using COMSOL multiphysics, as shown in Figure 19. Maximum pressure at the output node and especially in the free path compared to other paths. The coagulation effect of a vein can be easily identified when the shape of a vein is straight and has a laminar flow, as shown in Table 1.
Pressure |
||||
Open | Half close | |||
Vein Model | Min | Max | Min | Max |
Free path | 0.36 | 19.56 | −0.022 | 19.56 |
L | 0.00106 | 0.09609 | −0.0067 | 0.09609 |
U | 0.00153 | 0.09577 | 0.0004 | 0.09577 |
T | 0.00098 | 0.09571 | 0.00098 | 0.0002 |
The coagulation effect of the vein can be easily identified by blood velocity when the shape of the vein is straight and T-shaped with laminar flow detection, as described in Figure 20. The velocity of each model is evaluated using COMSOL multiphysics. There will be a maximum pressure and velocity at the outlet node and in the free path and T-shaped model compared to other types of designs, as indicated in Table 2.
Pressure |
||||
Open | Half close | |||
Vein Model | Min | Max | Min | Max |
Free Path | 0 | 0.35 | 0 | 0.012 |
L | 0 | 0.004 | 0 | 0.009 |
U | 0 | 0.0025 | 0 | 0.003 |
T | 0 | 0.0012 | 0 | 0.001 |
The design and analysis of different types of veins were modeled using COMSOL multiphysics. The coagulation effect on different types of veins may be analyzed by incorporating valves in the designs. A vein is considered in the free path and 50% open state. The pressure and velocity will be abnormal veins when there is a coagulation effect due to the change in pressure and velocity. These two parameters help analyze the blockages of the vein. The same values are applied to the MEMS sensor and converted into electrical signals to analyze diastole and systole values. MEMS piezoelectric model includes a gel that protects the skin from damage. The piezoelectric effect principle that has been proved using COMSOL multiphysics will help make wristband acts as a digital stethoscope different from regular stethoscopes. Pressure and velocity are the two essential parameters indicated in the blue and red marked designs. These two parameters are directly proportional in the free path laminar flow designs and inversely proportional when there is a coagulation effect. As MEMS is a tiny particle, this can be combined with a stent to protect during the second state of heart stroke.
[1] | Aswal N, Nawal M, Bundle M, et al. (2020) Pressure and velocity measurements in the pipeline for leak detection using COMSOL multiphysics. Int J Recent Technol Eng 9: 1812-1816. https://doi.org/10.35940/ijrte.A2727.059120 |
[2] | Iersel MV (2019) Analysis of flow patterns and interface behavior in simulations of immiscible liquid-liquid two-phase-flow in microchannels using the conservative level set method [Master Thesis]. Netherlands: Delft University of Technology Faculty of Applied Science. |
[3] | Nguyen H, Hoang T (2017) Numerical simulation of laminar flow through a pipe using COMSOL multiphysics. Int J Sci Eng Res 8: 290-295. |
[4] | Zhuang S Modeling and simulation of control valves via COMSOL multiphysics (2017). Available from: https://cn.comsol.com/paper/download/437642/zhuang_paper.pdf |
[5] |
Raza SS (2017) Analysis of a fully developed laminar flow b/w two parallel plates separated by a distance by using COMSOL multiphysics. J Fundam Appl Sci 9: 618. https://doi.org/10.4314/jfas.v9i1.36 ![]() |
[6] | Turgay MB (2017) Numerical simulation of fluid flow and heat transfer in a trapezoidal microchannel with COMSOL multiphysics. Int J Comput Method 73: 332-346. https://doi.org/10.1080/10407782.2017.1420302 |
[7] | Reddy RS, Payal G, Karkulali P, et al. (2016) Pressure and flow variation in gas distribution pipeline for leak detection. Int Conf Ind Technol 2016: 679-683. https://doi.org/10.1109/ICIT.2016.7474831 |
[8] | Kwon HJ (2013) Use of COMSOL simulation for undergraduate fluid dynamics course. Comput Educ J 23: 63-67. https://doi.org 10.18260/1-2-22167 |
[9] | Velez C, Ariza LF, Osma JF, et al. (2010) Velocity and pressure analysis for microchannel networks. IEEE ANDESCON 2010: 1-5. https://doi.org 10.1109/ANDESCON.2010.5632209 |
1. | Wenfang He, Siqiang Zheng, Hua Zhang, Bowen Gao, Juan Jin, Mingzhen Zhang, Qiang He, Plant-Derived Vesicle-Like Nanoparticles: Clinical Application Exploration and Challenges, 2023, Volume 18, 1178-2013, 5671, 10.2147/IJN.S428647 | |
2. | Balamurugan A.G, Saravanan Srinivasan, Preethi D, Monica P, Sandeep Kumar Mathivanan, Mohd Asif Shah, Robust brain tumor classification by fusion of deep learning and channel-wise attention mode approach, 2024, 24, 1471-2342, 10.1186/s12880-024-01323-3 | |
3. | Sirvan Khalighi, Kartik Reddy, Abhishek Midya, Krunal Balvantbhai Pandav, Anant Madabhushi, Malak Abedalthagafi, Artificial intelligence in neuro-oncology: advances and challenges in brain tumor diagnosis, prognosis, and precision treatment, 2024, 8, 2397-768X, 10.1038/s41698-024-00575-0 | |
4. | Hui Feng, Yang Yue, Yan Zhang, Jingqi Liang, Liang Liu, Qiong Wang, Qian Feng, Hongmou Zhao, Plant-Derived Exosome-Like Nanoparticles: Emerging Nanosystems for Enhanced Tissue Engineering, 2024, Volume 19, 1178-2013, 1189, 10.2147/IJN.S448905 | |
5. | Yonglu Wang, Zhijia Li, Yupei Ye, Yun Li, Ran Wei, Kaiyan Gan, Yuxin Qian, Lingxi Xu, Yue Kong, Luyang Guan, Hui Fang, Gongkai Jiao, Xiaoyan Ke, HD-tDCS effects on social impairment in autism spectrum disorder with sensory processing abnormalities: a randomized controlled trial, 2025, 15, 2045-2322, 10.1038/s41598-025-93631-z | |
6. | Eduardo Pinos, David Farfán, Juan Gózales, María del Cisne Ortega, Adriana Martínez, 2025, Chapter 4, 978-3-031-88063-6, 47, 10.1007/978-3-031-88064-3_4 | |
7. | Nikita Shinde, Mrunal Patil, Rakesh Kumar Sharma, Sachin Kadam, Shivaji Kashte, 2025, Chapter 16, 978-981-96-4240-3, 311, 10.1007/978-981-96-4241-0_16 |
Pressure |
||||
Open | Half close | |||
Vein Model | Min | Max | Min | Max |
Free path | 0.36 | 19.56 | −0.022 | 19.56 |
L | 0.00106 | 0.09609 | −0.0067 | 0.09609 |
U | 0.00153 | 0.09577 | 0.0004 | 0.09577 |
T | 0.00098 | 0.09571 | 0.00098 | 0.0002 |
Pressure |
||||
Open | Half close | |||
Vein Model | Min | Max | Min | Max |
Free Path | 0 | 0.35 | 0 | 0.012 |
L | 0 | 0.004 | 0 | 0.009 |
U | 0 | 0.0025 | 0 | 0.003 |
T | 0 | 0.0012 | 0 | 0.001 |
Pressure |
||||
Open | Half close | |||
Vein Model | Min | Max | Min | Max |
Free path | 0.36 | 19.56 | −0.022 | 19.56 |
L | 0.00106 | 0.09609 | −0.0067 | 0.09609 |
U | 0.00153 | 0.09577 | 0.0004 | 0.09577 |
T | 0.00098 | 0.09571 | 0.00098 | 0.0002 |
Pressure |
||||
Open | Half close | |||
Vein Model | Min | Max | Min | Max |
Free Path | 0 | 0.35 | 0 | 0.012 |
L | 0 | 0.004 | 0 | 0.009 |
U | 0 | 0.0025 | 0 | 0.003 |
T | 0 | 0.0012 | 0 | 0.001 |