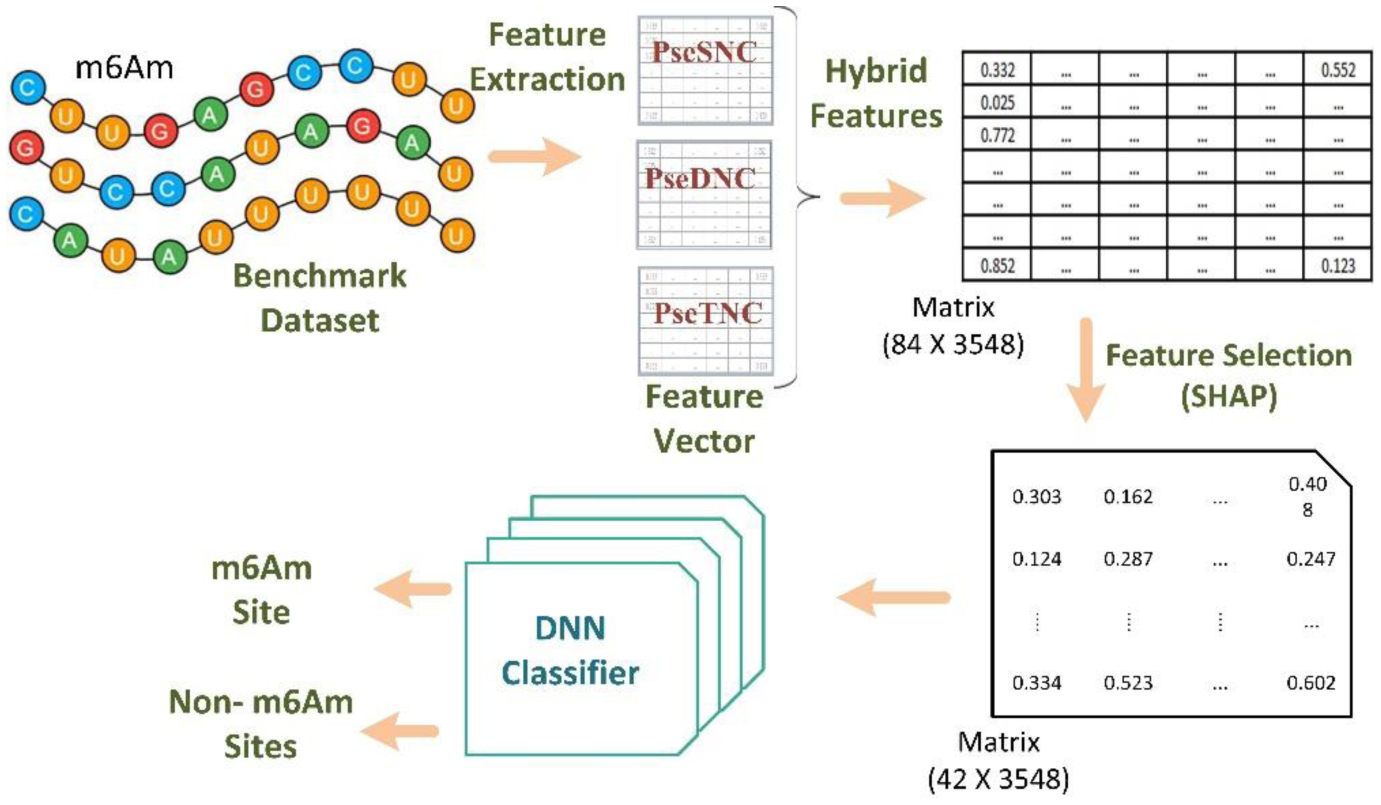
Citation: Yihan Zhang. Manufacture of complex heart tissues: technological advancements and future directions[J]. AIMS Bioengineering, 2021, 8(1): 73-92. doi: 10.3934/bioeng.2021008
[1] | Chunhong Li, Dandan Yang, Chuanzhi Bai . Some Opial type inequalities in (p, q)-calculus. AIMS Mathematics, 2020, 5(6): 5893-5902. doi: 10.3934/math.2020377 |
[2] | İbrahim Aktaş . On some geometric properties and Hardy class of q-Bessel functions. AIMS Mathematics, 2020, 5(4): 3156-3168. doi: 10.3934/math.2020203 |
[3] | Ghulam Farid, Saira Bano Akbar, Shafiq Ur Rehman, Josip Pečarić . Boundedness of fractional integral operators containing Mittag-Leffler functions via (s,m)-convexity. AIMS Mathematics, 2020, 5(2): 966-978. doi: 10.3934/math.2020067 |
[4] | Xiaoli Zhang, Shahid Khan, Saqib Hussain, Huo Tang, Zahid Shareef . New subclass of q-starlike functions associated with generalized conic domain. AIMS Mathematics, 2020, 5(5): 4830-4848. doi: 10.3934/math.2020308 |
[5] | Pinhong Long, Huo Tang, Wenshuai Wang . Functional inequalities for several classes of q-starlike and q-convex type analytic and multivalent functions using a generalized Bernardi integral operator. AIMS Mathematics, 2021, 6(2): 1191-1208. doi: 10.3934/math.2021073 |
[6] | Khadeejah Rasheed Alhindi, Khalid M. K. Alshammari, Huda Ali Aldweby . Classes of analytic functions involving the q-Ruschweyh operator and q-Bernardi operator. AIMS Mathematics, 2024, 9(11): 33301-33313. doi: 10.3934/math.20241589 |
[7] | Muhammad Sabil Ur Rehman, Qazi Zahoor Ahmad, H. M. Srivastava, Nazar Khan, Maslina Darus, Bilal Khan . Applications of higher-order q-derivatives to the subclass of q-starlike functions associated with the Janowski functions. AIMS Mathematics, 2021, 6(2): 1110-1125. doi: 10.3934/math.2021067 |
[8] | Hengxiao Qi, Muhammad Yussouf, Sajid Mehmood, Yu-Ming Chu, Ghulam Farid . Fractional integral versions of Hermite-Hadamard type inequality for generalized exponentially convexity. AIMS Mathematics, 2020, 5(6): 6030-6042. doi: 10.3934/math.2020386 |
[9] | Ghulam Farid, Maja Andrić, Maryam Saddiqa, Josip Pečarić, Chahn Yong Jung . Refinement and corrigendum of bounds of fractional integral operators containing Mittag-Leffler functions. AIMS Mathematics, 2020, 5(6): 7332-7349. doi: 10.3934/math.2020469 |
[10] | Waseem Ahmad Khan, Kottakkaran Sooppy Nisar, Dumitru Baleanu . A note on (p, q)-analogue type of Fubini numbers and polynomials. AIMS Mathematics, 2020, 5(3): 2743-2757. doi: 10.3934/math.2020177 |
N6,2′-O-dimethyladenosine (m6Am) is a significant RNA modification that plays a vital role in regulating various cellular processes, including gene expression, RNA stability, and the general integrity of RNA metabolism. This modification occurs at the five untranslated regions (UTRs) of messenger RNA (mRNA), influencing key RNA functions such as capping, translation initiation, and RNA decay [1]. m6Am has been shown to affect the interaction of RNA molecules with RNA-binding proteins, modulating critical processes like RNA splicing, transport, and stability. These modifications help regulate gene expression in response to cellular conditions and environmental cues, making them essential for maintaining cellular homeostasis. The dynamic and reversible nature of m6Am modifications in RNA is crucial for regulating mRNA's fate and ensuring the translation machinery's proper functioning [2]. The m6Am modification has gained attention due to its potential implications in disease pathogenesis and cellular dysfunction. The m6Am is linked to various biological processes, such as cell growth, differentiation, stress responses, and RNA surveillance mechanisms. The m6Am role in regulating mRNA stability suggests that it could regulate gene expression in response to stress or environmental changes, making it an essential factor in cellular adaptation and survival [3],[4]. Similarly, alterations in m6Am modification patterns have been associated with several diseases, including cancer, neurological disorders, and metabolic conditions, highlighting its significance in both health and disease. Its importance and accurate identification of m6Am sites within RNA sequences is essential for advancing the understanding of gene regulation and the molecular mechanisms that govern disease progression [5]. The ability to detect m6Am modifications opens new avenues for therapeutic interventions, enabling the development of targeted strategies for diseases that involve aberrant RNA modifications. As a result, computational methods that allow efficient and precise detection of m6Am sites are critical for advancing research in RNA biology and molecular medicine.
Advancements in computational biology have led to several learning tools for predicting RNA modifications, particularly m6Am. For example, Song et al. [6] introduced MultiRM, an attention-based multi-label neural network capable of predicting 12 RNA modifications simultaneously. Using an attention mechanism, MultiRM identifies modification sites and interprets key sequence contexts, revealing strong associations between different RNA modifications. The model achieves 71.13% accuracy with an MCC of 0.427 and an AUC of 0.805 on sequence-based RNA modification mechanisms. Jiang et al. [7] proposed m6AmPred using the eXtreme gradient boosting with dart (XGBDart) algorithm and EIIP-PseEIIP encoding for feature representation. m6AmPred achieved 73.10% accuracy with an MCC of 0.462 and an AUC of 0.820 on cross-validation. Similarly, Luo et al. [8] developed another model named DLm6Am, i.e., an ensemble deep-learning framework combining one-hot encoding, nucleotide chemical property (NCP), and nucleotide density (ND) for feature extraction. DLm6Am integrates CNN, BiLSTM, and multi-head attention modules, outperforming tools like m6AmPred and MultiRM with 79.55% accuracy, 81.71% sensitivity, 77.40% specificity, MCC of 0.591, and AUC of 0.863 on independent testing data. Recently, Jia et al. [9] proposed EMDL_m6Am, a stacking ensemble model employing one-hot encoding and integrating DenseNet, inflated convolutional network (DCNN), and deep multiscale residual network (MSRN) for feature extraction. EMDL_m6Am achieved 80.98% accuracy, 82.25% sensitivity, 79.72% specificity, MCC of 0.619, and AUC of 0.823 on training data, with independent testing (80.98% accuracy, AUC of 0.8211). Despite advancements, existing methods struggle with limited encoding schemes, inefficient feature selection, and reliance on single deep learning frameworks, leading to suboptimal performance and high computational costs. The lack of explainability in current models significantly hinders the interpretation and improvement of accuracy, robustness, and interpretability in m6Am site prediction techniques.
Based on the aforementioned considerations, in this study, we propose Deep-m6Am, a novel deep learning (DL) model designed to accurately identify m6Am sites in RNA sequences. The model integrates multiple feature extraction techniques, including pseudo single nucleotide composition (PseSNC), pseudo dinucleotide composition (PseDNC), and pseudo trinucleotide composition (PseTNC), to capture complex sequence patterns essential for precise prediction. A SHAP (SHapley Additive exPlanations)-based feature selection mechanism is incorporated to enhance computational efficiency and eliminate irrelevant or redundant features, ensuring that only the most informative features contribute to the model's predictions. The Deep-m6Am framework addresses the limitations of single-model approaches by leveraging a multilayer deep neural network (DNN) classifier, improving robustness and generalizability. The model's performance was rigorously evaluated using 5-fold cross-validation and independent testing. The Deep-m6Am demonstrates state-of-the-art results across multiple evaluation metrics, including accuracy, sensitivity, specificity, AUC, and MCC, outperforming existing models and traditional ML algorithms. Integrating cutting-edge feature extraction, selection, and deep learning methodologies, Deep-m6Am provides a powerful and interpretable tool for predicting RNA modifications. This advancement significantly contributes to RNA biology by offering more profound insights into RNA modifications and their roles in disease mechanisms, opening promising avenues for further research into RNA modification patterns. Therefore, Deep-m6Am is a robust computational framework for addressing key challenges in RNA modification analysis, as illustrated in Figure 1.
The rest of the paper is organized as follows: Section 2 presents material and methods, Section 3 illustrates performance metrics and evaluation, Section 4 provides experimental results and analysis, and the work is concluded in Section 5.
A valid and reliable benchmark dataset is essential for designing a powerful and robust computational model. In this study, we utilized the same benchmark datasets employed by Jia et al. [9]. These sites were regarded as highly confident, providing a solid foundation for accurate and reliable model development. Initially, sample sequences were extracted for the training dataset, as depicted in Eq. 1.
Where T1 represents the total RNA sequences,
Where T2 represents the total RNA sequences,
Dataset | Number of samples | Positive samples | Negative samples |
Cross validation | 3548 | 1774 | 1774 |
Training dataset | 2838 | 1419 | 1419 |
Independent dataset | 710 | 355 | 355 |
Several techniques have been developed to convert DNA, protein, and RNA sequences into discrete mathematical models, maintaining the nucleotides' outstanding features and structural integrity. These methods ensure that the biological sequences are accurately described in numerical formats, enabling computational analysis without losing critical sequence-specific information. Accordingly, several bioinformatics approaches have been developed that can transform RNA sequences into various statistical equations with the preservation of the uniqueness and inherent patterns of the measures [10]–[13]. Following the second rule of Chou's 5-step guidelines, several feature extraction techniques have been implemented in this paper to improve the representation of RNA sequences. These techniques include pseudo K-tuple nucleotide composition (PseKNC), comprising methods like PseSNC (Ƙ = 1), PseDNC (Ƙ = 2), and PseTNC (Ƙ = 3). Feature extraction methods are explained in detail in the next section. The PseKNC approach represents RNA sequences as functional vectors by encoding their composition and sequence patterns. This method suppresses detailed order data, focusing on capturing essential features that suggest similarities between RNA samples. By transforming the sequences into structured mathematical representations, PseKNC facilitates efficient computational analysis while preserving key biological characteristics of the RNA [14]. Let us consider an RNA sequence R with N number of nucleotides, represented in Eq. 3.
Where N represents the number of nucleotides in a RNA sequence (i.e. the length of a RNA sequence) and
The Eq. 3, can be expressed in the general form of the PseKNC as
In RNA sequence representation, T is the transposed vector representing a mathematical transformation, z represents a numeric value typically corresponding to an output or dependent variable in the analysis, and ϕy represents the actual value of the RNA sequence's feature vector and can be computed using Eq. 5.
Where θj represent the jth tier correlation factor or jth rank correlation factor that reflects the sequence order correlation in most contiguous K-tuple nucleotides. λ represents the total number correlation rank and w represents the weight. This paper uses the PseKNC technique to convert the provided sequences into discrete feature vectors while maintaining the sequence order data. By designating different values to K (i.e., K = 1, 2, 3) in Eq. 4, three distinct modes of PseKNC were obtained, i.e., PseSNC (K = 1), PseDNC (K = 2), and PseTNC (K = 3), defined as follows:
This study used three distinct feature extraction methods to encode RNA sequences into discrete feature vectors, as summarized in Table 2. These features include PseSNC, PseDNC, and PseTNC, which integrate pseudo, composition, and transitional probability features to improve the differentiation and interpretation of nucleotide sequences [17]–[19]. All individual features were incorporated to construct a comprehensive hybrid feature vector by capturing diverse sequence-derived attributes. Machine learning models leveraging hybrid features benefit from combining multiple extraction techniques, enhancing predictive performance by effectively capturing complex data patterns. This approach remains a widely adopted strategy in bioinformatics and genomics for improving model interpretability and accuracy.
Feature extraction methods | Features |
Pseudo single nucleotide composition (PseSNC) | 4 |
pseudo dinucleotide composition (PseDNC) | 16 |
Pseudo trinucleotide composition (PseTNC) | 64 |
Hybrid features | 84 |
Feature selection is critical in developing models to improve overall performance and computational efficiency. Feature selection involves identifying and retaining the most informative features while eliminating irrelevant or redundant ones, which can introduce noise and reduce prediction accuracy. This study employs SHAP (SHapley Additive exPlanations) as a robust feature selection technique. SHAP leverages cooperative game theory to quantify the contribution of each feature to the model's predictions, ensuring that only the most significant features are retained [20]. This approach reduces the dataset's dimensionality and enhances the model's interpretability by providing insights into the importance of individual features. By integrating SHAP into the Deep-m6Am framework, the model achieves optimized computational efficiency and improved generalization, enabling more accurate and reliable identification of m6Am sites in RNA sequences. This feature selection strategy is pivotal in addressing the challenges of high-dimensional data and ensuring the model's robustness and scalability. This approach enhances model interpretability and supports robust data analysis; it can be expressed as in Eq. 11.
Where φi represents the SHAP value for the feature i, N is the set of all features, and S is a subset of features excluding i. Then, f(S) is the model's prediction given features in S, and
The network topology of a deep neural network, an algorithm based on machine learning or artificial intelligence inspired by the human brain, includes input and output layers and multiple hidden layers. The mechanism of neuron transmission and activation function in DNN is shown in Figure 2. Unlike traditional processing techniques, DNNs can self-learn and automatically acquire pertinent features from unstructured or raw data. Domains in which DNN has been successfully implemented include speech recognition, NLP (Natural Language Processing) and bioengineering, and imaging [21].
The proposed architecture utilizes fully connected layers to locate m6Am sites in RNA sequences. The input layer comprises 42 nodes linked to a first hidden layer of 32 nodes through weighted connections. A second hidden layer with 16 nodes processes outputs from the first layer, followed by a third and last layer with 8 nodes. Each layer employs the rectified linear unit (ReLU) activation function, enabling the model to detect nonlinear relationships and complex patterns [22]. The output layer uses the sigmoid activation function for normalized binary classification, distinguishing m6Am and non-m6Am sites in RNA sequences.
The Deep-m6Am performance is rigorously evaluated using key metrics, including accuracy (ACC), sensitivity (SN), specificity (SP), Matthews correlation coefficient (MCC), and area under the curve (AUC) [23]. SN measures the model's ability to accurately identify true m6Am sites, while SP evaluates its capacity to predict negative cases correctly. ACC reflects the overall correctness of predictions, MCC provides a balanced classification performance assessment, and AUC highlights the model's ability to distinguish between positive and negative instances. These metrics comprehensively evaluate the model's predictive power, ensuring its reliability and effectiveness in identifying m6Am sites.
Where T+ symbolizes true positives, F+ symbolizes false positives, T- Symbolizes true negatives, and F- false negatives, respectively.
In this section, we analyze the hyperparameters of the Deep-m6Am model to optimize its performance. The key hyperparameters considered include learning rate (LR), batch size, number of layers, neurons per layer, and dropout rate. A dropout rate of 0.5 and L2 regularization (0.001) is applied to prevent overfitting, while Xavier initialization ensures stable weight distribution. The model is trained using the Adam optimizer with a learning rate of 0.01 and a momentum of 0.9 to accelerate convergence. Training is conducted for 100 epochs, utilizing ReLU activation functions in the hidden layers and Softmax activation in the output layer for effective learning and classification. A grid search technique was employed to assess the proposed model performance under various hyperparameters, exploring different combinations of parameters. Specifically, the analysis focused on the hyperparameters that significantly influence the performance of the DNN model, including the activation function, learning rate, and number of iterations. Table 3 presents the optimal hyperparameters for the Deep-m6Am.
Parameter | Optimal value |
Dropout rate | 0.5 |
Weight initialization function | Xavier |
Seed | 12345L |
Dropout | 0.001 |
Number of hidden layers | 3 |
Optimizer | Adam, SGD |
L2 regularization | 0.001 |
Epochs | 100 |
Learning rate | 0.01 |
Batch size | 16 |
Activation functions | ReLU, Softmax |
Momentum | 0.9 |
In this section, we conduct the performance analysis of the proposed Deep-m6Am model. We conducted experiments to examine the effects of LR. Table 4 presents a detailed comparison of performance metrics across different learning rates and shows how the chosen learning rate significantly impacts the model's effectiveness and reliability.
LR | ACC (%) | SN (%) | SP (%) | MCC |
0.01 | 83.43 | 82.64 | 84.22 | 0.669 |
0.02 | 80.05 | 79.71 | 80.38 | 0.601 |
0.03 | 79.43 | 80.27 | 78.58 | 0.589 |
0.04 | 78.86 | 78.58 | 79.14 | 0.577 |
0.05 | 78.70 | 75.00 | 82.40 | 0.672 |
As shown in Table 4, the Deep-m6Am model achieves optimal performance with a learning rate of 0.01, attaining the highest accuracy (ACC) of 83.43%, sensitivity (SN) of 82.64%, specificity (SP) of 84.22%, and MCC of 0.669. However, as the learning rate increases, the model's performance declines, highlighting that excessively higher learning rates negatively influence overall metrics.
Furthermore, in Figure 3, we analyze the model's fluctuation in performance with different dropout rates, offering valuable guidance for optimizing this hyperparameter. Proper optimization is crucial for balancing generalization and overfitting prevention, ensuring a robust and reliable model. Figure 3 shows that the model achieves optimal performance at a dropout rate of 0.5, with the highest ACC (83.43%) and MCC (66.90%). Performance improves as the dropout rate increases from 0.1 to 0.5, highlighting 0.5 as the most effective rate for balancing generalization and accuracy.
Moreover, we analyze the effect of varying batch sizes on model performance, comparing outcomes across different sizes to identify optimal configurations. Figure 4 illustrates the impact of batch size on model performance, showing a decline as the batch size increases from 16 to 256. The model achieves optimal performance at a batch size of 16, with the highest ACC (83.43%) and MCC (69.90%). As batch size increases, performance gradually decreases, emphasizing the importance of tuning this hyperparameter for optimal results.
Evaluating the robustness of statistical learning models is essential, and this is typically achieved through validation techniques such as jackknife, k-fold cross-validation, and subsampling. Among these methods, k-fold cross-validation is particularly effective for objectively assessing model performance by dividing the dataset into multiple test sets. This approach ensures a thorough evaluation of the model's generalizability and reliability. Table 5 presents a performance comparison of the proposed Deep-m6Am model using various feature extraction techniques, including individual, hybrid, and SHAP-based feature selection methods.
Method | ACC (%) | SN (%) | SP (%) | MCC |
PseSNC | 71.70 | 66.55 | 76.40 | 0.570 |
PseDNC | 77.73 | 76.89 | 78.58 | 0.555 |
PseTNC | 79.43 | 80.27 | 78.58 | 0.589 |
Hybrid features | 80.83 | 80.27 | 81.40 | 0.617 |
Hybrid features after SHAP | 83.43 | 82.64 | 84.22 | 0.669 |
Table 5 highlights the varying predictive performance of individual features, with PseSNC, PseDNC, and PseTNC achieving ACCs of 71.70%, 77.73%, and 79.43%, respectively. The hybrid feature approach significantly improves classification, reaching an ACC of 80.83%. Further enhancement through SHAP-based feature selection optimizes feature importance, achieving the highest ACC (83.43%) and MCC (0.669). These results underscore the effectiveness of hybrid features in capturing complex patterns and the role of SHAP in refining feature selection for improved model performance.
In this section, we provide an analysis of the DNN model in comparison to well-known machine learning algorithms such as K-nearest neighbor (KNN), random forest (RF), decision tree (DT), naive Bayes (NB), and support vector machine (SVM) [16],[24]–[26]. Table 6 illustrates the importance of evaluating model performance across different classifiers. We employed a 5-fold cross-validation scheme to ensure a reliable and unbiased performance assessment.
Classifiers | ACC (%) | SN (%) | SP (%) | MCC |
RF | 68.72 | 66.91 | 70.53 | 0.667 |
DT | 71.80 | 70.22 | 73.38 | 0.706 |
KNN | 77.15 | 75.62 | 78.68 | 0.741 |
NB | 79.99 | 78.35 | 81.63 | 0.712 |
SVM | 82.53 | 81.96 | 83.09 | 0.651 |
Deep-m6Am | 83.43 | 82.64 | 84.22 | 0.669 |
Table 6 shows that Deep-m6Am outperforms other ML algorithms, achieving the highest ACC (83.43%) and MCC (0.669). SVM follows with an ACC of 82.53%, while NB and KNN achieve 79.99% and 77.15%, respectively. DT (71.80%) and RF (68.72%) perform lower. These results highlight Deep-m6Am as the most effective model for m6Am site identification. To analyze further, we evaluate the proposed model performance using the Area Under the ROC Curve (AUC), as shown in Figure 5. Figure 5 show that the proposed model achieved an AUC value of 0.853, indicating excellent performance compared with widely used ML algorithms.
Furthermore, Table 7 evaluates various ML algorithms on an independent dataset to assess generalizability and robustness. From Table 7, the proposed Deep-m6Am model demonstrated superior performance among the ML classifiers, achieving an ACC of 82.86% with an MCC of 0.657%. The SVM classifier had an ACC of 81.64% with an MCC of 0.632. In contrast, KNN achieved an ACC of 75.45%, while NB, DT, and RF performed at 77.29%, 68.88%, and 66.22%, respectively. This analysis identifies Deep-m6Am as the top-performing model, showcasing its superiority in handling dataset complexities and ensuring reliable and accurate m6Am site prediction.
Classifiers | ACC (%) | SN (%) | SP (%) | MCC |
RF | 66.22 | 64.50 | 68.00 | 0.325 |
DT | 68.88 | 67.10 | 70.66 | 0.378 |
KNN | 75.45 | 73.20 | 77.70 | 0.509 |
NB | 77.29 | 75.20 | 79.38 | 0.545 |
SVM | 81.64 | 79.50 | 83.78 | 0.632 |
Deep-m6Am | 82.86 | 83.65 | 82.07 | 0.657 |
In this section, we conduct a detailed comparative analysis of the proposed Deep-m6Am predictor against several state-of-the-art predictors, including MultiRM [6], m6AmPred [7], DLm6Am [8], and EMDL_m6Am [9]. This comparison is shown in Table 8.
Predictor | ACC (%) | SN (%) | SP (%) | MCC |
MultiRM [6] | 71.13 | 78.59 | 63.66 | 0.427 |
m6AmPred [7] | 73.10 | 72.11 | 74.08 | 0.462 |
DLm6Am [8] | 79.55 | 81.71 | 77.40 | 0.591 |
EMDL_m6Am [9] | 80.98 | 82.25 | 79.72 | 0.619 |
Deep-m6Am | 82.86 | 83.65 | 82.07 | 0.657 |
From Table 8, the MultiRM achieved an ACC of 71.13% and MCC of 0.427, while m6AmPred had an ACC of 73.10% and MCC of 0.462. DLm6Am demonstrated an ACC of 79.55% and MCC of 0.591, and EMDL_m6Am obtained an ACC of 80.98% and MCC of 0.619. In comparison, the proposed Deep-m6Am outperformed all these models, achieving the highest ACC of 82.86% and MCC of 0.657. These results highlight the superior predictive accuracy and robustness of Deep-m6Am for m6Am site identification, making it the most effective model among the evaluated predictors.
The biological function of N6,2′-O-dimethyladenosine (m6Am) in RNA sequences underscores its critical role in regulating post-transcriptional processes, RNA stability, and translation. This study introduces the Deep-m6Am model, which employs a hybrid feature extraction approach, incorporating SHAP (SHapley Additive exPlanations) feature selection and DNN classifier to precisely identify m6Am sites within RNA sequences. Through 5-fold cross-validation, compared with popular ML methods, Deep-m6Am demonstrated unique advantages that resulted in more precise m6Am site predictions. Furthermore, the proposed Deep-m6Am model showed superior performance metrics, achieving an average accuracy of 82.86% compared to the existing models. These results underscore the potential of Deep-m6Am as a reliable and efficient tool for advancing RNA modification analysis.
Future research could expand Deep-m6Am to analyze other RNA modifications and integrate multi-omics data for enhanced predictive accuracy. Exploring its role in disease-specific studies could advance precision medicine. Optimizing computational efficiency through transfer learning, hyperparameter optimization, and parallel programming will improve the model's scalability and applicability in RNA biology and medical research [27].
[1] | Cardiovascular diseases (CVDs), World Health Organization Available from: https://www.who.int/health-topics/cardiovascular-diseases. |
[2] |
Fuchs M, Schibilsky D, Zeh W, et al. (2019) Does the heart transplant have a future? Eur J Cardio-thorac 55: i38-i48. doi: 10.1093/ejcts/ezz107
![]() |
[3] |
Qasim M, Haq F, Kang M, et al. (2019) 3D printing approaches for cardiac tissue engineering and role of immune modulation in tissue regeneration. Int J Nanomed 14: 1311-1333. doi: 10.2147/IJN.S189587
![]() |
[4] |
Takahashi K, Yamanaka S (2006) Induction of pluripotent stem cells from mouse embryonic and adult fibroblast cultures by defined factors. Cell 126: 663-676. doi: 10.1016/j.cell.2006.07.024
![]() |
[5] |
Smith Z, Sindhu C, Meissner A (2016) Molecular features of cellular reprogramming and development. Nat Rev Mol Cell Biol 17: 139-154. doi: 10.1038/nrm.2016.6
![]() |
[6] |
Okita K, Ichisaka T, Yamanaka S (2007) Generation of germline-competent induced pluripotent stem cells. Nature 448: 313-317. doi: 10.1038/nature05934
![]() |
[7] |
Yoshida Y, Yamanaka S (2017) Induced pluripotent stem cells 10 years later. Circ Res 120: 1958-1968. doi: 10.1161/CIRCRESAHA.117.311080
![]() |
[8] |
Maherali N, Sridharan R, Xie W, et al. (2007) Directly reprogrammed fibroblasts show global epigenetic remodeling and widespread tissue contribution. Cell Stem Cell 1: 55-70. doi: 10.1016/j.stem.2007.05.014
![]() |
[9] |
Somers A, Jean JC, Sommer CA, et al. (2010) Generation of transgene-free lung disease-specific human induced pluripotent stem cells using a single excisable lentiviral stem cell cassette. Stem cells 28: 1728-1740. doi: 10.1002/stem.495
![]() |
[10] | Fusaki N, Ban H, Nishiyama A, et al. (2009) Efficient induction of transgene-free human pluripotent stem cells using a vector based on Sendai virus, an RNA virus that does not integrate into the host genome. P Jpn Acad B Phys 85: 348-362. |
[11] |
Carey BW, Markoulaki S, Hanna J, et al. (2009) Reprogramming of murine and human somatic cells using a single polycistronic vector. Proc Natl Acad Sci USA 106: 157-162. doi: 10.1073/pnas.0811426106
![]() |
[12] |
Stadtfeld M, Nagaya M, Utikal J, et al. (2008) Induced pluripotent stem cells generated without viral integration. Science 322: 945-949. doi: 10.1126/science.1162494
![]() |
[13] |
McLenachan S, Sarsero J, Ioannou P (2007) Flow-cytometric analysis of mouse embryonic stem cell lipofection using small and large DNA constructs. Genomics 89: 708-720. doi: 10.1016/j.ygeno.2007.02.006
![]() |
[14] |
Subramanyam D, Lamouille S, Judson R, et al. (2011) Multiple targets of miR-302 and miR-372 promote reprogramming of human fibroblasts to induced pluripotent stem cells. Nat Biotechnol 29: 443-448. doi: 10.1038/nbt.1862
![]() |
[15] |
Kim D, Kim CH, Moon JI, et al. (2009) Generation of human induced pluripotent stem cells by direct delivery of reprogramming proteins. Cell Stem Cell 4: 472-476. doi: 10.1016/j.stem.2009.05.005
![]() |
[16] |
Warren L, Manos PD, Ahfeldt T, et al. (2010) Highly efficient reprogramming to pluripotency and directed differentiation of human cells with synthetic modified mRNA. Cell Stem Cell 7: 618-630. doi: 10.1016/j.stem.2010.08.012
![]() |
[17] |
Malik N, Rao MS (2013) A review of the methods for human iPSC derivation. Pluripotent Stem Cells: Methods and Protocols Totowa: Humana Press, 23-33. doi: 10.1007/978-1-62703-348-0_3
![]() |
[18] |
Hong H, Takahashi K, Ichisaka T, et al. (2009) Suppression of induced pluripotent stem cell generation by the p53–p21 pathway. Nature 460: 1132-1135. doi: 10.1038/nature08235
![]() |
[19] |
Zhao S, Jiang E, Chen S, et al. (2016) PiggyBac transposon vectors: the tools of the human gene encoding. Transl Lung Cancer Res 5: 120-125. doi: 10.21037/tcr.2016.04.02
![]() |
[20] |
Al Abbar A, Ngai SC, Nograles N, et al. (2020) Induced pluripotent stem cells: Reprogramming platforms and applications in cell replacement therapy. Biores Open Access 9: 121-136. doi: 10.1089/biores.2019.0046
![]() |
[21] |
Gouveia C, Huyser C, Egli D, et al. (2020) Lessons learned from somatic cell nuclear transfer. Int J Mol Sci 21: 2314. doi: 10.3390/ijms21072314
![]() |
[22] |
Tsuji Y, Kato Y, Tsunoda Y (2009) The developmental potential of mouse somatic cell nuclear-transferred oocytes treated with trichostatin A and 5-aza-2′-deoxycytidine. Zygote 17: 109-115. doi: 10.1017/S0967199408005133
![]() |
[23] |
Polstein LR, Perez-Pinera P, Kocak DD, et al. (2015) Genome-wide specificity of DNA binding, gene regulation, and chromatin remodeling by TALE- and CRISPR/Cas9-based transcriptional activators. Genome Res 25: 1158-1169. doi: 10.1101/gr.179044.114
![]() |
[24] |
Weltner J, Balboa D, Katayama S, et al. (2018) Human pluripotent reprogramming with CRISPR activators. Nat Commun 9: 2643. doi: 10.1038/s41467-018-05067-x
![]() |
[25] |
Liu P, Chen M, Liu Y, et al. (2017) CRISPR-based chromatin remodeling of the endogenous Oct4 or Sox2 locus enables reprogramming to pluripotency. Cell Stem Cell 22: 252-261.e4. doi: 10.1016/j.stem.2017.12.001
![]() |
[26] |
Ben JR, Shemer Y, Binah O (2018) Genome editing in induced pluripotent stem cells using CRISPR/Cas9. Stem Cell Rev Rep 14: 323-336. doi: 10.1007/s12015-018-9811-3
![]() |
[27] |
Brodehl A, Ebbinghaus H, Deutsch MA, et al. (2019) Human induced pluripotent stem-cell-derived cardiomyocytes as models for genetic cardiomyopathies. Int J Mol Sci 20: 4381. doi: 10.3390/ijms20184381
![]() |
[28] |
Kempf H, Zweigerdt R (2017) Scalable cardiac differentiation of pluripotent stem cells using specific growth factors and small molecules. Engineering and Application of Pluripotent Stem Cells Cham: Springer, 39-69. doi: 10.1007/10_2017_30
![]() |
[29] |
Wu S, Cheng CM, Lanz RB, et al. (2013) Atrial identity is determined by a COUP-TFII regulatory network. Dev Cell 25: 417-426. doi: 10.1016/j.devcel.2013.04.017
![]() |
[30] |
Prowse AB, Timmins NE, Yau TM, et al. (2014) Transforming the promise of pluripotent stem cell-derived cardiomyocytes to a therapy: challenges and solutions for clinical trials. Can J Cardiol 30: 1335-1349. doi: 10.1016/j.cjca.2014.08.005
![]() |
[31] |
Evans SM, Yelon D, Conlon FL, et al. (2010) Myocardial lineage development. Circ Res 107: 1428-1444. doi: 10.1161/CIRCRESAHA.110.227405
![]() |
[32] | Mahmood T, Nasser A, Hossein B (2015) Human cardiomyocyte generation from pluripotent stem cells: A state-of-art. Life Sciences 145: 98-113. |
[33] |
Guo NN, Liu LP, Zheng YW, et al. (2020) Inducing human induced pluripotent stem cell differentiation through embryoid bodies: A practical and stable approach. J Stem Cells 12: 25-34. doi: 10.4252/wjsc.v12.i1.25
![]() |
[34] |
Brickman JM, Serup P (2017) Properties of embryoid bodies. WIREs Dev Biol 6: e259. doi: 10.1002/wdev.259
![]() |
[35] |
Yang L, Soonpaa MH, Adler ED, et al. (2008) Human cardiovascular progenitor cells develop from a KDR+ embryonic-stem-cell-derived population. Nature 453: 524-528. doi: 10.1038/nature06894
![]() |
[36] |
Kattman J, Witty AD, Gagliardi M, et al. (2011) Stage-specific optimization of activin/nodal and BMP signaling promotes cardiac differentiation of mouse and human pluripotent stem cell lines. Cell Stem Cell 8: 228-240. doi: 10.1016/j.stem.2010.12.008
![]() |
[37] |
Besser RR, Ishahak M, Mayo V, et al. (2018) Engineered microenvironments for maturation of stem cell derived cardiac myocytes. Theranostics 8: 124-140. doi: 10.7150/thno.19441
![]() |
[38] |
Tohyama S, Hattori F, Sano M, et al. (2012) Distinct metabolic flow enables large-scale purification of mouse and human pluripotent stem cell-derived cardiomyocytes. Cell Stem Cell 12: 127-137. doi: 10.1016/j.stem.2012.09.013
![]() |
[39] |
Mummery CL, Zhang J, Elliott DA, et al. (2012) Differentiation of human embryonic stem cells and induced pluripotent stem cells to cardiomyocytes: a methods overview. Circ Res 111: 344-358. doi: 10.1161/CIRCRESAHA.110.227512
![]() |
[40] |
Graichen R, Xu X, Braam SR, et al. (2008) Enhanced cardiomyogenesis of human embryonic stem cells by a small molecular inhibitor of p38 MAPK. Differentiation 76: 357-370. doi: 10.1111/j.1432-0436.2007.00236.x
![]() |
[41] |
Vlahos CJ, Matter WF, Hui KY, et al. (1994) A specific inhibitor of phosphatidylinositol3-kinase, 2-(4-morpholinyl)-8-phenyl-4H-1-benzopyran-4-one (LY294002). J Biol Chem 269: 5241-5248. doi: 10.1016/S0021-9258(17)37680-9
![]() |
[42] |
Ieda M, Fu JD, Delgado P, et al. (2010) Direct reprogramming of fibroblasts into functional cardiomyocytes by defined factors. Cell 142: 375-386. doi: 10.1016/j.cell.2010.07.002
![]() |
[43] |
Jayawardena TM, Egemnazarov B, Finch EA, et al. (2012) MicroRNA-mediated in vitro and in vivo direct reprogramming of cardiac fibroblasts to cardiomyocytes. Circ Res 110: 1465-1473. doi: 10.1161/CIRCRESAHA.112.269035
![]() |
[44] |
Burridge PW, Matsa E, Shukla P, et al. (2014) Chemically defined generation of human cardiomyocytes. Nat Methods 11: 855-860. doi: 10.1038/nmeth.2999
![]() |
[45] |
Srivastava D, DeWitt N (2016) In vivo cellular reprogramming: the next generation. Cell 166: 1386-1396. doi: 10.1016/j.cell.2016.08.055
![]() |
[46] |
Chen Y, Yang Z, Zhao ZA, et al. (2017) Direct reprogramming of fibroblasts into cardiomyocytes. Stem Cell Res Ther 8: 118. doi: 10.1186/s13287-017-0569-3
![]() |
[47] |
Wang J, Jiang X, Zhao L, et al. (2019) Lineage reprogramming of fibroblasts into induced cardiac progenitor cells by CRISPR/Cas9-based transcriptional activators. Acta Pharm Sin B 10: 313-326. doi: 10.1016/j.apsb.2019.09.003
![]() |
[48] |
Murphy SV, Atala A (2014) 3D bioprinting of tissues and organs. Nat Biotechnol 32: 773-785. doi: 10.1038/nbt.2958
![]() |
[49] |
Cui X, Boland T, DLima DD, et al. (2012) Thermal inkjet printing in tissue engineering and regenerative medicine. Recent Pat Drug Deliv Formul 6: 149-155. doi: 10.2174/187221112800672949
![]() |
[50] |
Cui X, Dean D, Ruggeri ZM, et al. (2010) Cell damage evaluation of thermal inkjet printed Chinese hamster ovary cells. Biotechnol Bioeng 106: 963-969. doi: 10.1002/bit.22762
![]() |
[51] |
Fang Y, Frampton JP, Raghavan S, et al. (2012) Rapid generation of multiplexed cell cocultures using acoustic droplet ejection followed by aqueous two-phase exclusion patterning. Tissue Eng Part C-Me 18: 647-657. doi: 10.1089/ten.tec.2011.0709
![]() |
[52] |
Jones N (2012) Science in three dimensions: the print revolution. Nature 487: 22-23. doi: 10.1038/487022a
![]() |
[53] |
Mironov V, Visconti RP, Kasyanov V, et al. (2008) Organ printing: tissue spheroids as building blocks. Biomaterials 30: 2164-2174. doi: 10.1016/j.biomaterials.2008.12.084
![]() |
[54] |
Zhang YS, Pi Q, van Genderen AM Microfluidic bioprinting for engineering vascularized tissues and organoids (2017) . doi: 10.3791/55957
![]() |
[55] |
Bohandy J, Kim B, Adrian F (1986) Metal deposition from a supported metal film using an excimer laser. J Appl Phys 60: 1538-1539. doi: 10.1063/1.337287
![]() |
[56] |
Guillemot F, Souquet A, Catros S, et al. (2010) Laser-assisted cell printing: principle, physical parameters versus cell fate and perspectives in tissue engineering. Nanomedicine 5: 507-515. doi: 10.2217/nnm.10.14
![]() |
[57] |
Guillotin B, Souquet A, Catros S, et al. (2010) Laser assisted bioprinting of engineered tissue with high cell density and microscale organization. Biomaterials 31: 7250-7256. doi: 10.1016/j.biomaterials.2010.05.055
![]() |
[58] |
Kattamis NT, Purnick PE, Weiss R, et al. (2007) Thick film laser induced forward transfer for deposition of thermally and mechanically sensitive materials. Appl Phys Lett 91: 171120-171123. doi: 10.1063/1.2799877
![]() |
[59] |
Gauvin R, Chen YC, Lee JW, et al. (2012) Microfabrication of complex porous tissue engineering scaffolds using 3D projection stereolithography. Biomaterials 33: 3824-3834. doi: 10.1016/j.biomaterials.2012.01.048
![]() |
[60] |
Alonzo M, AnilKumar S, Roman B, et al. (2019) 3D Bioprinting of cardiac tissue and cardiac stem cell therapy. Transl Res 211: 64-83. doi: 10.1016/j.trsl.2019.04.004
![]() |
[61] |
Bishop ES, Mostafa S, Pakvasa M, et al. (2017) 3-D bioprinting technologies in tissue engineering and regenerative medicine: Current and future trends. Genes Dis 4: 185-195. doi: 10.1016/j.gendis.2017.10.002
![]() |
[62] |
Morris VB, Nimbalkar S, Younesi M, et al. (2017) Mechanical properties, cytocompatibility and manufacturability of chitosan: PEGDA hybrid-gel scaffolds by stereolithography. Ann Biomed Eng 45: 286-296. doi: 10.1007/s10439-016-1643-1
![]() |
[63] |
Lu Y, Mapili G, Suhali G, et al. (2006) A digital micro-mirror device-based system for the microfabrication of complex, spatially patterned tissue engineering scaffolds. J Biomed Mater Res A 77: 396-405. doi: 10.1002/jbm.a.30601
![]() |
[64] |
Zhang J, Hu Q, Wang S, et al. (2019) Digital light processing based three-dimensional printing for medical applications. Int J Bioprint 6: 242. doi: 10.18063/ijb.v6i1.242
![]() |
[65] |
Kelly BE, Bhattacharya I, Heidari H, et al. (2019) Volumetric additive manufacturing via tomographic reconstruction. Science 363: 1075-1079. doi: 10.1126/science.aau7114
![]() |
[66] |
Türker E, Demirçak N, Arslan YA (2018) Scaffold-free three-dimensional cell culturing using magnetic levitation. Biomater Sci 6: 1745-1753. doi: 10.1039/C8BM00122G
![]() |
[67] |
Matai I, Kaur G, Seyedsalehi A, et al. (2019) Progress in 3D bioprinting technology for tissue/organ regenerative engineering. Biomaterials 226: 119536. doi: 10.1016/j.biomaterials.2019.119536
![]() |
[68] |
Tseng H, Gage JA, Haisler WL, et al. (2016) A high-throughput in vitro ring assay for vasoactivity using magnetic 3D bioprinting. Sci Rep 6: 30640. doi: 10.1038/srep30640
![]() |
[69] |
Bowser DA, Moore MJ (2019) Biofabrication of neural microphysiological systems using magnetic spheroid bioprinting. Biofabrication 12: 015002. doi: 10.1088/1758-5090/ab41b4
![]() |
[70] |
Wang X (2019) Advanced polymers for three-dimensional (3D) organ bioprinting. Micromachines 10: 814. doi: 10.3390/mi10120814
![]() |
[71] |
Axpe E, Oyen ML (2016) Applications of alginate-based bioinks in 3D bioprinting. Int J Mol Sci 17: E1976. doi: 10.3390/ijms17121976
![]() |
[72] |
Bajpai SK, Sharma S (2004) Investigation of swelling/degradation behaviour of alginate beads crosslinked with Ca2+ and Ba2+ ions. React Funct Polym 59: 129-140. doi: 10.1016/j.reactfunctpolym.2004.01.002
![]() |
[73] |
Gao T, Gillispie GJ, Copus JS, et al. (2018) Optimization of gelatin–alginate composite bioink printability using rheological parameters: a systematic approach. Biofabrication 10: 034106. doi: 10.1088/1758-5090/aacdc7
![]() |
[74] |
Giuseppe MD, Law N, Webb BA, et al. (2018) Mechanical behaviour of alginate-gelatin hydrogels for 3D bioprinting. J Mech Behav Biomed Mater 79: 150-157. doi: 10.1016/j.jmbbm.2017.12.018
![]() |
[75] |
Markstedt K, Mantas A, Tournier I, et al. (2015) 3D bioprinting human chondrocytes with nanocellulose-alginate bioink for cartilage tissue engineering applications. Biomacromolecules 16: 1489-1496. doi: 10.1021/acs.biomac.5b00188
![]() |
[76] |
Frantz C, Stewart KM, Weaver VM (2010) The extracellular matrix at a glance. J Cell Sci 123: 4195-4200. doi: 10.1242/jcs.023820
![]() |
[77] |
Drzewiecki KE, Parmar AS, Gaudet ID, et al. (2014) Methacrylation induces rapid, temperature-dependent, reversible self-assembly of type-I collagen. Langmuir 30: 11204-11211. doi: 10.1021/la502418s
![]() |
[78] |
Lee A, Hudson AR, Shiwarski DJ, et al. (2019) 3D bioprinting of collagen to rebuild components of the human heart. Science 365: 482-487. doi: 10.1126/science.aav9051
![]() |
[79] |
Wang X, Yu X, Yan Y, et al. (2008) Liver tissue responses to gelatin and gelatin/chitosan gels. J Biomed Mater Res A 87: 62-68. doi: 10.1002/jbm.a.31712
![]() |
[80] |
Skardal A, Zhang J, McCoard L, et al. (2010) Photocrosslinkable hyaluronan-gelatin hydrogels for two-step bioprinting. Tissue Eng Part A 16: 2675-2685. doi: 10.1089/ten.tea.2009.0798
![]() |
[81] |
Zhu H, Yang H, Ma Y, et al. (2020) Spatiotemporally controlled photoresponsive hydrogels: design and predictive modeling from processing through application. Adv Funct Mater 30: 2000639. doi: 10.1002/adfm.202000639
![]() |
[82] |
Xiao S, Zhao T, Wang J, et al. (2019) Gelatin methacrylate (GelMA)-based hydrogels for cell transplantation: an effective strategy for tissue engineering. Stem Cell Rev Rep 15: 664-679. doi: 10.1007/s12015-019-09893-4
![]() |
[83] |
Hoffman AS (2002) Hydrogels for biomedical applications. Adv Drug Deliv Rev 54: 3-12. doi: 10.1016/S0169-409X(01)00239-3
![]() |
[84] |
Jungst T, Smolan W, Schacht K, et al. (2016) Strategies and molecular design criteria for 3D printable hydrogels. Chem Rev 116: 1496-1539. doi: 10.1021/acs.chemrev.5b00303
![]() |
[85] |
Astete CE, Sabliov CM (2006) Synthesis and characterization of PLGA nanoparticles. J Biomater Sci Polym Ed 17: 247-289. doi: 10.1163/156856206775997322
![]() |
[86] |
Samadi N, Abbadessa A, Di Stefano A, et al. (2013) The effect of lauryl capping group on protein release and degradation of poly (D, L-lactic-co-glycolic acid) particles. J Control Release 172: 436-443. doi: 10.1016/j.jconrel.2013.05.034
![]() |
[87] |
Mazzola M, Pasquale E (2020) Toward cardiac regeneration: Combination of pluripotent stem cell-based therapies and bioengineering strategies. Front Bioeng Biotechnol 8: 455. doi: 10.3389/fbioe.2020.00455
![]() |
[88] |
Homma J, Shimizu S, Sekine H, et al. (2020) A novel method to align cells in a cardiac tissue-like construct fabricated by cell sheet-based tissue engineering. J Tissue Eng Regen Med 14: 944-954. doi: 10.1002/term.3074
![]() |
[89] |
Wang Z, Lee SJ, Cheng HJ, et al. (2018) 3D bioprinted functional and contractile cardiac tissue constructs. Acta Biomater 70: 48-56. doi: 10.1016/j.actbio.2018.02.007
![]() |
[90] |
Redd MA, Zeinstra N, Qin W, et al. (2019) Patterned human microvascular grafts enable rapid vascularization and increase perfusion in infarcted rat hearts. Nat Commun 10: 584. doi: 10.1038/s41467-019-08388-7
![]() |
[91] |
Shimizu A, Goh WH, Itai S, et al. (2020) ECM-based microchannel for culturing in vitro vascular tissues with simultaneous perfusion and stretch. Lab Chip 20: 1917-1927. doi: 10.1039/D0LC00254B
![]() |
[92] |
Ma X, Qu X, Zhu W, et al. (2016) Deterministically patterned biomimetic human iPSC-derived hepatic model via rapid 3D bioprinting. Proc Natl Acad Sci 113: 2206-2211. doi: 10.1073/pnas.1524510113
![]() |
[93] |
Taylor DA, Sampaio LC, Ferdous Z, et al. (2018) Decellularized matrices in regenerative medicine. Acta Biomater 74: 74-89. doi: 10.1016/j.actbio.2018.04.044
![]() |
[94] |
Ott HC, Matthiesen TS, Goh SK, et al. (2008) Perfusion-decellularized matrix: using nature's platform to engineer a bioartificial heart. Nat Med 14: 213-219. doi: 10.1038/nm1684
![]() |
[95] |
Jovic TH, Kungwengwe G, Mills AC, et al. (2019) Plant-derived biomaterials: A review of 3D bioprinting and biomedical applications. Front Mech Eng 5: 19. doi: 10.3389/fmech.2019.00019
![]() |
[96] |
Marga F, Jakab K, Khatiwala C, et al. (2012) Toward engineering functional organ modules by additive manufacturing. Biofabrication 4: 022001. doi: 10.1088/1758-5082/4/2/022001
![]() |
[97] |
Hockaday LA, Kang KH, Colangelo NW, et al. (2012) Rapid 3D printing of anatomically accurate and mechanically heterogeneous aortic valve hydrogel scaffolds. Biofabrication 4: 035005. doi: 10.1088/1758-5082/4/3/035005
![]() |
[98] |
Madden LR, Mortisen DJ, Sussman EM, et al. (2010) Proangiogenic scaffolds as functional templates for cardiac tissue engineering. Proc Natl Acad Sci 107: 15211-15216. doi: 10.1073/pnas.1006442107
![]() |
[99] |
Zhang YS, Arneri A, Bersini S, et al. (2016) Bioprinting 3D microfibrous scaffolds for engineering endothelialized myocardium and heart-on-a-chip. Biomaterials 110: 45-59. doi: 10.1016/j.biomaterials.2016.09.003
![]() |
[100] |
Hann SY, Cui H, Esworthy T, et al. (2019) Recent advances in 3D printing: vascular network for tissue and organ regeneration. Transl Res 211: 46-63. doi: 10.1016/j.trsl.2019.04.002
![]() |
[101] |
Duan B, Hockaday LA, Kang KH, et al. (2013) 3D bioprinting of heterogeneous aortic valve conduits with alginate/gelatin hydrogels. J Biomed Mater Res A 101: 1255-1264. doi: 10.1002/jbm.a.34420
![]() |
[102] |
Grigoryan B, Paulsen SJ, Corbett DC, et al. (2019) Multivascular networks and functional intravascular topologies within biocompatible hydrogels. Science 364: 458-464. doi: 10.1126/science.aav9750
![]() |
[103] |
Esther C, Claudia K, Petra J (2011) Vascularization is the key challenge in tissue engineering. Adv Drug Deliv Rev 63: 300-311. doi: 10.1016/j.addr.2011.03.004
![]() |
[104] |
Kobayashi J, Akiyama Y, Yamato M, et al. (2018) Design of temperature-responsive cell culture surfaces for cell sheet-based regenerative therapy and 3D tissue fabrication. Adv Exp Med Biol 1078: 371-393. doi: 10.1007/978-981-13-0950-2_19
![]() |
[105] |
Inui A, Sekine H, Sano K, et al. (2019) Generation of a large-scale vascular bed for the in vitro creation of three-dimensional cardiac tissue. Regen Ther 11: 316-323. doi: 10.1016/j.reth.2019.10.001
![]() |
[106] |
Masuda S, Shimizu T (2015) Three-dimensional cardiac tissue fabrication based on cell sheet technology. Adv Drug Deliv Rev 96: 103-109. doi: 10.1016/j.addr.2015.05.002
![]() |
[107] |
Daley MC, Fenn SL, Black LD (2018) Applications of cardiac extracellular matrix in tissue engineering and regenerative medicine. Cardiac Extracellular Matrix Cham: Springer, 59-83. doi: 10.1007/978-3-319-97421-7_4
![]() |
[108] |
Seignez C, Phillipson M (2017) The multitasking neutrophils and their involvement in angiogenesis. Curr Opin Hematol 24: 3-8. doi: 10.1097/MOH.0000000000000300
![]() |
[109] |
Miller JS (2014) The billion cells construct: will three-dimensional printing get us there? PLoS Biol 12: e1001882. doi: 10.1371/journal.pbio.1001882
![]() |
1. | Bilal Khan, Hari M. Srivastava, Nazar Khan, Maslina Darus, Muhammad Tahir, Qazi Zahoor Ahmad, Coefficient Estimates for a Subclass of Analytic Functions Associated with a Certain Leaf-Like Domain, 2020, 8, 2227-7390, 1334, 10.3390/math8081334 | |
2. | H. M. Srivastava, Operators of Basic (or q-) Calculus and Fractional q-Calculus and Their Applications in Geometric Function Theory of Complex Analysis, 2020, 44, 1028-6276, 327, 10.1007/s40995-019-00815-0 | |
3. | Muhammad Sabil Ur Rehman, Qazi Zahoor Ahmad, H. M. Srivastava, Nazar Khan, Maslina Darus, Muhammad Tahir, Coefficient inequalities for certain subclasses of multivalent functions associated with conic domain, 2020, 2020, 1029-242X, 10.1186/s13660-020-02446-1 | |
4. | Huo Tang, Shahid Khan, Saqib Hussain, Nasir Khan, Hankel and Toeplitz determinant for a subclass of multivalent $ q $-starlike functions of order $ \alpha $, 2021, 6, 2473-6988, 5421, 10.3934/math.2021320 | |
5. | Bilal Khan, Zhi-Guo Liu, Hari M. Srivastava, Nazar Khan, Maslina Darus, Muhammad Tahir, A Study of Some Families of Multivalent q-Starlike Functions Involving Higher-Order q-Derivatives, 2020, 8, 2227-7390, 1470, 10.3390/math8091470 | |
6. | Shahid Khan, Saqib Hussain, Maslina Darus, Inclusion relations of $ q $-Bessel functions associated with generalized conic domain, 2021, 6, 2473-6988, 3624, 10.3934/math.2021216 | |
7. | Nazek Alessa, B. Venkateswarlu, K. Loganathan, T.S. Karthik, P. Thirupathi Reddy, G. Sujatha, Ming-Sheng Liu, Certain Class of Analytic Functions Connected with q -Analogue of the Bessel Function, 2021, 2021, 2314-4785, 1, 10.1155/2021/5587886 | |
8. | Mohammad Faisal Khan, Anjali Goswami, Shahid Khan, Certain New Subclass of Multivalent Q-Starlike Functions Associated with Q-Symmetric Calculus, 2022, 6, 2504-3110, 367, 10.3390/fractalfract6070367 | |
9. | Dong Liu, Muhey U Din, Mohsan Raza, Sarfraz Nawaz Malik, Huo Tang, Convexity, Starlikeness, and Prestarlikeness of Wright Functions, 2022, 10, 2227-7390, 3858, 10.3390/math10203858 | |
10. | Bilal Khan, H. M. Srivastava, Sama Arjika, Shahid Khan, Nazar Khan, Qazi Zahoor Ahmad, A certain q-Ruscheweyh type derivative operator and its applications involving multivalent functions, 2021, 2021, 1687-1847, 10.1186/s13662-021-03441-6 | |
11. | Wali Khan Mashwan, Bakhtiar Ahmad, Muhammad Ghaffar Khan, Saima Mustafa, Sama Arjika, Bilal Khan, A. M. Bastos Pereira, Pascu-Type Analytic Functions by Using Mittag-Leffler Functions in Janowski Domain, 2021, 2021, 1563-5147, 1, 10.1155/2021/1209871 | |
12. | Bilal Khan, Zhi-Guo Liu, H. M. Srivastava, Serkan Araci, Nazar Khan, Qazi Zahoor Ahmad, Higher-order q-derivatives and their applications to subclasses of multivalent Janowski type q-starlike functions, 2021, 2021, 1687-1847, 10.1186/s13662-021-03611-6 | |
13. | Hari M. Srivastava, Nazar Khan, Shahid Khan, Qazi Zahoor Ahmad, Bilal Khan, A Class of k-Symmetric Harmonic Functions Involving a Certain q-Derivative Operator, 2021, 9, 2227-7390, 1812, 10.3390/math9151812 | |
14. | Bakhtiar Ahmad, Wali Khan Mashwani, Serkan Araci, Saima Mustafa, Muhammad Ghaffar Khan, Bilal Khan, A subclass of meromorphic Janowski-type multivalent q-starlike functions involving a q-differential operator, 2022, 2022, 2731-4235, 10.1186/s13662-022-03683-y | |
15. | Alina Alb Lupaş, Applications of the q-Sălăgean Differential Operator Involving Multivalent Functions, 2022, 11, 2075-1680, 512, 10.3390/axioms11100512 | |
16. | Qiuxia Hu, Hari M. Srivastava, Bakhtiar Ahmad, Nazar Khan, Muhammad Ghaffar Khan, Wali Khan Mashwani, Bilal Khan, A Subclass of Multivalent Janowski Type q-Starlike Functions and Its Consequences, 2021, 13, 2073-8994, 1275, 10.3390/sym13071275 | |
17. | Sercan Kazımoğlu, Erhan Deniz, Partial sums of the Rabotnov function, 2022, 14, 2066-7752, 250, 10.2478/ausm-2022-0017 | |
18. | Bilal Khan, H. M. Srivastava, Muhammad Tahir, Maslina Darus, Qazi Zahoor Ahmad, Nazar Khan, Applications of a certain $q$-integral operator to the subclasses of analytic and bi-univalent functions, 2021, 6, 2473-6988, 1024, 10.3934/math.2021061 | |
19. | Timilehin Gideon Shaba, Serkan Araci, Babatunde Olufemi Adebesin, 2023, Investigating q-Exponential Functions in the Context of Bi-Univalent Functions: Insights into the Fekctc-Szcgö Problem and Second Hankel Determinant, 979-8-3503-5883-4, 1, 10.1109/ICMEAS58693.2023.10429891 | |
20. | Sarem H. Hadi, Maslina Darus, 2024, 3023, 0094-243X, 070002, 10.1063/5.0172085 | |
21. | Basem Aref Frasin, Sercan Kazımoğlu, Applications of the normalized Le Roy-type Mittag-Leffler function on partial sums of analytic functions, 2025, 36, 1012-9405, 10.1007/s13370-025-01272-2 |
Dataset | Number of samples | Positive samples | Negative samples |
Cross validation | 3548 | 1774 | 1774 |
Training dataset | 2838 | 1419 | 1419 |
Independent dataset | 710 | 355 | 355 |
Feature extraction methods | Features |
Pseudo single nucleotide composition (PseSNC) | 4 |
pseudo dinucleotide composition (PseDNC) | 16 |
Pseudo trinucleotide composition (PseTNC) | 64 |
Hybrid features | 84 |
Parameter | Optimal value |
Dropout rate | 0.5 |
Weight initialization function | Xavier |
Seed | 12345L |
Dropout | 0.001 |
Number of hidden layers | 3 |
Optimizer | Adam, SGD |
L2 regularization | 0.001 |
Epochs | 100 |
Learning rate | 0.01 |
Batch size | 16 |
Activation functions | ReLU, Softmax |
Momentum | 0.9 |
LR | ACC (%) | SN (%) | SP (%) | MCC |
0.01 | 83.43 | 82.64 | 84.22 | 0.669 |
0.02 | 80.05 | 79.71 | 80.38 | 0.601 |
0.03 | 79.43 | 80.27 | 78.58 | 0.589 |
0.04 | 78.86 | 78.58 | 79.14 | 0.577 |
0.05 | 78.70 | 75.00 | 82.40 | 0.672 |
Method | ACC (%) | SN (%) | SP (%) | MCC |
PseSNC | 71.70 | 66.55 | 76.40 | 0.570 |
PseDNC | 77.73 | 76.89 | 78.58 | 0.555 |
PseTNC | 79.43 | 80.27 | 78.58 | 0.589 |
Hybrid features | 80.83 | 80.27 | 81.40 | 0.617 |
Hybrid features after SHAP | 83.43 | 82.64 | 84.22 | 0.669 |
Classifiers | ACC (%) | SN (%) | SP (%) | MCC |
RF | 68.72 | 66.91 | 70.53 | 0.667 |
DT | 71.80 | 70.22 | 73.38 | 0.706 |
KNN | 77.15 | 75.62 | 78.68 | 0.741 |
NB | 79.99 | 78.35 | 81.63 | 0.712 |
SVM | 82.53 | 81.96 | 83.09 | 0.651 |
Deep-m6Am | 83.43 | 82.64 | 84.22 | 0.669 |
Classifiers | ACC (%) | SN (%) | SP (%) | MCC |
RF | 66.22 | 64.50 | 68.00 | 0.325 |
DT | 68.88 | 67.10 | 70.66 | 0.378 |
KNN | 75.45 | 73.20 | 77.70 | 0.509 |
NB | 77.29 | 75.20 | 79.38 | 0.545 |
SVM | 81.64 | 79.50 | 83.78 | 0.632 |
Deep-m6Am | 82.86 | 83.65 | 82.07 | 0.657 |
Dataset | Number of samples | Positive samples | Negative samples |
Cross validation | 3548 | 1774 | 1774 |
Training dataset | 2838 | 1419 | 1419 |
Independent dataset | 710 | 355 | 355 |
Feature extraction methods | Features |
Pseudo single nucleotide composition (PseSNC) | 4 |
pseudo dinucleotide composition (PseDNC) | 16 |
Pseudo trinucleotide composition (PseTNC) | 64 |
Hybrid features | 84 |
Parameter | Optimal value |
Dropout rate | 0.5 |
Weight initialization function | Xavier |
Seed | 12345L |
Dropout | 0.001 |
Number of hidden layers | 3 |
Optimizer | Adam, SGD |
L2 regularization | 0.001 |
Epochs | 100 |
Learning rate | 0.01 |
Batch size | 16 |
Activation functions | ReLU, Softmax |
Momentum | 0.9 |
LR | ACC (%) | SN (%) | SP (%) | MCC |
0.01 | 83.43 | 82.64 | 84.22 | 0.669 |
0.02 | 80.05 | 79.71 | 80.38 | 0.601 |
0.03 | 79.43 | 80.27 | 78.58 | 0.589 |
0.04 | 78.86 | 78.58 | 79.14 | 0.577 |
0.05 | 78.70 | 75.00 | 82.40 | 0.672 |
Method | ACC (%) | SN (%) | SP (%) | MCC |
PseSNC | 71.70 | 66.55 | 76.40 | 0.570 |
PseDNC | 77.73 | 76.89 | 78.58 | 0.555 |
PseTNC | 79.43 | 80.27 | 78.58 | 0.589 |
Hybrid features | 80.83 | 80.27 | 81.40 | 0.617 |
Hybrid features after SHAP | 83.43 | 82.64 | 84.22 | 0.669 |
Classifiers | ACC (%) | SN (%) | SP (%) | MCC |
RF | 68.72 | 66.91 | 70.53 | 0.667 |
DT | 71.80 | 70.22 | 73.38 | 0.706 |
KNN | 77.15 | 75.62 | 78.68 | 0.741 |
NB | 79.99 | 78.35 | 81.63 | 0.712 |
SVM | 82.53 | 81.96 | 83.09 | 0.651 |
Deep-m6Am | 83.43 | 82.64 | 84.22 | 0.669 |
Classifiers | ACC (%) | SN (%) | SP (%) | MCC |
RF | 66.22 | 64.50 | 68.00 | 0.325 |
DT | 68.88 | 67.10 | 70.66 | 0.378 |
KNN | 75.45 | 73.20 | 77.70 | 0.509 |
NB | 77.29 | 75.20 | 79.38 | 0.545 |
SVM | 81.64 | 79.50 | 83.78 | 0.632 |
Deep-m6Am | 82.86 | 83.65 | 82.07 | 0.657 |
Predictor | ACC (%) | SN (%) | SP (%) | MCC |
MultiRM [6] | 71.13 | 78.59 | 63.66 | 0.427 |
m6AmPred [7] | 73.10 | 72.11 | 74.08 | 0.462 |
DLm6Am [8] | 79.55 | 81.71 | 77.40 | 0.591 |
EMDL_m6Am [9] | 80.98 | 82.25 | 79.72 | 0.619 |
Deep-m6Am | 82.86 | 83.65 | 82.07 | 0.657 |